Thyroid Cancer
BASIC THYROID ANATOMY AND PHYSIOLOGY
Gross Anatomy
The thyroid gland is located in the central anterior neck at the cervical–thoracic junction. The bulk of the gland is located immediately anterior and inferior to the thyroid cartilage. It has two lateral lobes connected by a central isthmus. Approximately 50% of individuals have a pyramidal lobe that extends superiorly from the central aspect of the gland, which is a remnant of the gland’s embryologic origin, the thyroglossal duct. The average adult thyroid gland measures approximately 5 × 5 cm and weighs 10 to 20 g.
The location of the gland in the central neck and its relation to critical structures explain the presenting symptoms of advanced neoplastic processes arising from the thyroid as well as potential complications of thyroid surgery and radiotherapy (Fig. 50.1). The lateral lobes extend superiorly to the level of the midthyroid cartilage overlying the larynx and inferiorly to the sixth tracheal ring. The gland wraps around 75% of the tracheal circumference, posteriorly encroaching on the esophagus. The lateral extent is just medial to the common carotid arteries. The recurrent laryngeal nerves, sympathetic trunks, vagus, and phrenic nerves are all found immediately posterior to the gland, and the gland is anteriorly bound by the strap muscles. Parathyroid glands are located posterior to the thyroid gland and vary in location and number.
Vasculature and Lymphatics
The arterial blood supply to the thyroid gland is provided by the paired superior thyroid arteries (branches of the external carotid arteries) and inferior thyroid arteries (branches of the thyrocervical trunk from the subclavian arteries). Venous blood drains via the paired superior and middle thyroid veins to the internal jugular veins and from the inferior thyroid veins to the subclavian and innominate veins. There is a dense lymphatic network draining the gland in multiple directions, and bilateral involvement of lymph node metastases is common. According to the American Joint Committee on Cancer’s (AJCC) seventh edition of the AJCC Cancer Staging Handbook, the first-echelon nodes for thyroid cancer metastases are located in level 6 (the central or “visceral” compartment) between the hyoid bone and the thoracic inlet.1 Specifically, these are the paralaryngeal, paratracheal, and prelaryngeal (Delphian) nodes. Second-echelon nodal spread is to the mid- and lower cervical nodes (levels 3 and 4), supraclavicular nodes, upper mediastinal nodes (level 7), and to a lesser extent the upper cervical nodes (level 2). Retropharyngeal node involvement is unusual but can be encountered in the setting of advanced nodal disease. Level 1 (submental and submandibular) lymph nodes are rarely involved.
FIGURE 50.1. Anatomy of the thyroid gland. A: Digitally reconstructed radiograph of a patient’s head and neck showing the relative position of the thyroid gland (blue) to the hyoid bone (green) and bottom of the cricoid cartilage (purple). B: Contrast-enhanced axial computed tomography slice at the level of the bottom of the cricoid cartilage (purple) showing the position of the thyroid gland (blue) relative to the esophagus (orange), carotid arteries (red), jugular vein (lavender) and the approximate position of the recurrent laryngeal nerves (yellow-green).
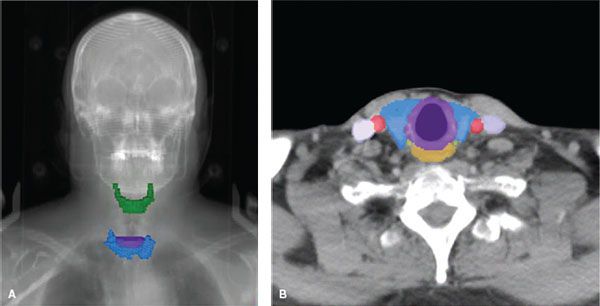
Microscopic Anatomy
Microscopically, the normal thyroid gland consists of numerous lobules comprising individual follicles forming the structural and functional unit of the gland. The supporting stoma and vasculature are intertwined around the follicles. Each follicle consists of a single layer of cuboidal surface epithelium comprising thyroid follicular cells surrounding a central lumen-containing colloid, a substance rich in thyroglobulin (Tg). Approximately 10% of the follicular epithelium contains parafollicular cells, or C cells, which are neural crest-derived cells containing granules of calcitonin. The gland is incompletely surrounded by a connective tissue capsule.
Physiology
The primary physiologic role of the thyroid gland is the production of thyroid hormone, which plays an important role in metabolic homeostasis. A secondary role is the production of calcitonin, a hormone involved in calcium homeostasis. The follicular cells of the thyroid gland synthesize and secrete Tg and thyroid hormone in two biologically active forms, thyroxine (3,5,3′,5′ iodothyronine or T4) and triiodothyronine (3,5,3′ iodothyronine or T3). It is useful to consider T4 as the storage and transport form of thyroid hormone and T3 as the metabolically active form. Most circulating thyroid hormone is bound to thyroxine-binding globulin. In peripheral tissues, where thyroid hormone executes its endocrine function, T4 is rapidly converted to the more active form T3 by the action of T4 monodeiodinase. T3 is transported to the nucleus where it binds to specific nuclear receptors and interacts with regulatory genes, influencing their expression. The gene products ultimately increase the cell’s basal metabolic rate, protein synthesis, catecholamine effects, and growth of long bones and play an essential role in metabolism of proteins, fats, and carbohydrates. Thyroid hormone exerts it endocrine function on virtually all cells in the body.
Iodine is a critical component of thyroid hormone and is essential for thyroid function. The recommended daily intake is 150 μg, and at least 50 μg of daily intake is necessary to prevent deficiency, resulting in a goiter. The follicular cells of the thyroid gland possess a unique ability to actively uptake and concentrate iodine. The sodium iodine symporter (NaIS) actively transports sodium and iodine against an electrochemical gradient across the cell membrane in an energy-dependent fashion. This transmembrane protein is stimulated by thyroid-stimulating hormone (TSH or thyrotropin), which is synthesized in the anterior pituitary. Functional NaIS is present on malignant follicular cells seen in multiple variants of differentiated thyroid cancer. The unique ability to concentrate iodine within these malignant cells makes radioactive iodine (RAI) a potent targeted therapy. The central role of TSH in driving the internalization of iodine within follicular thyroid cells is exploited in preparation for RAI. To increase the efficacy of iodine-131 (I-131) therapy, T4 deprivation, a low-iodine diet, and administration of recombinant human TSH (rhTSH) can be used to increases the effects of TSH on follicular thyroid cells (see the section on preparing patients for I-131). NaIS is also present in the parotid glands, breast tissues, gastric mucosa, and nasolacrimal ducts, placing them at risk for injury from RAI therapy.
Upon entering the follicular cells, iodine is transported across the apical membrane and oxidized to a form that binds to tyrosyl residues of Tg, a large (660 kDa) glycoprotein synthesized by the follicular cells. Iodinated Tg becomes the main component of the intraluminal colloid stores of the thyroid follicles. T3 and T4 are synthesized by the coupling of iodinated tyrosine. They remain attached to the Tg until leaving the gland. TSH stimulation results in endocytosis of colloid droplets from the lumen into the follicular cells where it is hydrolyzed and releases Tg, T4, and, to lesser degree, T3 into the circulation. Malignant differentiated follicular cells retain this function. Thus, serum Tg becomes a unique tumor marker after thyroidectomy and thyroid remnant ablation.2
A feedback loop between the thyroid, pituitary, and hypothalamus regulates thyroid hormone synthesis and secretion. Thyrotropin-releasing hormone (TRH) is synthesized in the hypothalamus, and its primary function is to increase the secretion of TSH in the pituitary. Both T3 and T4 in turn inhibit TRH release and TSH secretion.
CLASSIFICATIONS OF THYROID CANCER
Correct pathological classification of thyroid carcinoma is fundamental to the appropriate clinical management of the malignancy. A system of classification is shown in Table 50.1 and discussed below. The cell of origin, cytological features, and growth morphology determine the pathologic classification. All carcinomas of the thyroid gland are derived from the follicular epithelium, except medullary thyroid carcinoma (MTC), which is derived from the parafollicular C cells. Capsular invasion is important in the pathologic diagnosis of certain thyroid carcinomas. The diagnosis of papillary thyroid carcinoma (PTC) is entirely predicated on the presence of diagnostic nuclear features and does not require invasive growth, while follicular carcinoma (FC) requires the presence of capsular or vascular invasion.3 Other rarely encountered malignant neoplasms of the thyroid gland include thyroid lymphoma and sarcomas. These two entities will not be discussed in this chapter and are better evaluated and managed under paradigms detailed in the chapters on lymphoma and sarcoma.
Differentiated (Follicular-Derived) Thyroid Carcinoma
The two major subgroups comprising differentiated thyroid carcinoma (DTC) are PTC and FC. They are distinguished by architectural and cytological features.
TABLE 50.1 PATHOLOGICAL CLASSIFICATION FOR THYROID CANCER
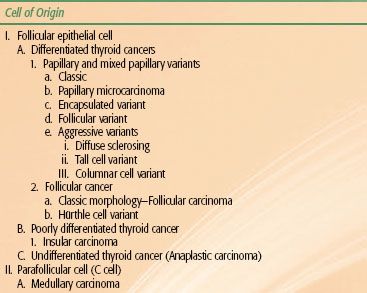
Papillary Thyroid Cancer
PTC is the most common histologic type, representing approximately 80% to 90% of all thyroid cancer diagnoses, and has the best prognosis of all thyroid malignancies.4 Malignant nuclear cytological characteristics are the distinguishing feature of PTC and include nuclear enlargement, hypochromasia, intranuclear cytoplasmic inclusions (nuclear pseudoinclusions), nuclear grooves, and distinct nucleoli. After formalin fixation, nuclei may appear pale, optically clear, and resemble “Orphan Annie’s eyes.” PTC is strongly lymphotropic, and multicentric disease within the parenchyma of the gland is often present secondary to early lymphatic spread. All PTC stain strongly for Tg.5–7
In its classic variant, PTC has a tumor architecture comprising branching papillae with fibrovascular cores. Papillary microcarcinoma is used to describe incidentally identified papillary carcinoma measuring <1.0 cm in diameter. The encapsulated variant of PTC (approximately 10% of PTCs) is surrounded by a thick fibrous capsule, unlike most PTCs that are nonencapsulated and infiltrate the surrounding thyroid parenchyma. Although not as favorable as microcarcinoma, encapsulated PTC has a favorable prognosis compared with classic PTC. The follicular variant of PTC differs from the classic variant by its follicular growth pattern; however, it retains the nuclear features diagnostic of PTC. Its prognosis roughly mirrors that of classic PTC. Other variants include diffuse sclerosing, tall cell, and columnar cell PTC, which have a much poorer prognosis.
Diffuse sclerosing is a rare form of PTC, with histologic features of extensive sclerosis, lymphocytic infiltrate, and psammoma bodies. A greater percentage of patients with diffuse sclerosing PTC present with extrathyroidal extension or distant metastases. Likewise, tall cell and columnar variants are commonly associated with poor prognostic features such as advanced age, large tumor size, aggressive histologic features with early invasion, and distant metastases. The distinguishing feature of tall cell PTC is that at least 70% of the carcinoma is composed of cells that are at least twice as tall as they are wide because of abundant cellular cytoplasm. Columnar cell variant is extremely rare. These carcinomas histologically show striking nuclear stratification, usually with papillary architecture. However, the usual nuclear features of PTCs may be absent; therefore, some pathologists prefer to classify columnar cell as poorly differentiated carcinomas.
Follicular Carcinoma
FC, like PTC, is a DTC of follicular cell origin. They comprise 15% to 30% of all thyroid carcinomas and share a similar prognosis to classic PTC. This malignant thyroid neoplasm lacks the cytological features of PTC. These carcinomas show evidence of thyroid follicle formation and are usually well circumscribed with a defined tumor capsule. They are grossly indistinguishable from follicular adenomas. The diagnosis of FC is dependent on the presence of one of two histologic features: (a) tumor invasion through the entire tumor capsule or (b) tumor invasion into a blood vessel located in the tumor capsule or immediately outside the tumor capsule. Minimally invasive forms of FC may present a pathologic challenge and often require serial sectioning to find evidence of capsular or vascular invasion, while widely invasive forms of FC are obviously invasive tumors both on gross and microscopic evaluation.
Hürthle Cell Carcinoma
This follicular epithelial-derived carcinoma, also known as oncocytic carcinoma, is characterized by large cells with abundant granular eosinophilic cytoplasms. Hürthle cells may be seen in both benign and other non-Hürthle cell malignant neoplastic lesions; however, at least 75% of the tumor must be comprised of Hürthle cells to designate it Hürthle cell carcinoma. Similar to FC, Hürthle cell carcinoma requires the presence of capsular or vascular invasion to classify it as a malignant neoplasm. Although Hürthle cell carcinoma is classically included under the subclassification FC, it is now apparent that there are both follicular and papillary types. Hürthle cell carcinoma has a less-favorable prognosis and usually presents with large tumor size, more invasive features, early regional and distant metastatic spread, and lower likelihood to concentrate RAI.
Poorly Differentiated Thyroid Carcinoma (Insular Carcinoma)
These follicular epithelial cell-derived tumors are intermediate between DTC and undifferentiated (anaplastic) thyroid cancers (ATC). Both their histologic appearance and biological behavior are more aggressive than DTC. They tend to be widely invasive and many will extend beyond the gland. Insular carcinoma is characterized by tumor cells that are arranged in discrete nests separated by a fibrous stroma. Microscopically, they are usually solid with some evidence of follicle formation. Mitoses and necrosis are often present. The presence of more differentiated areas of tumor admixed with insular carcinoma suggests that the latter represents “dedifferentiated” tumors.
Undifferentiated (Anaplastic) Thyroid Carcinoma
ATC comprise <5% of all malignant thyroid neoplasms. This is the most aggressive form of thyroid carcinoma, accounting for 14% to 50% of all thyroid cancer deaths. Most patients are diagnosed at the age of 65 years or older.8 ATC originates from the thyroid follicular epithelium but shows little to no evidence of histologic differentiation. This tumor is defined as much by its clinical behavior as its histologic appearance. When diagnosed, most ATCs are widely invasive, replacing most if not all of the normal-appearing gland, and freely infiltrate the perithyroidal tissues. They are usually accompanied by bulky metastatic lymphadenopathy and distant metastatic spread. Gross pathologic evaluation is characterized by widely infiltrative disease with areas of necrosis and hemorrhage. Various microscopic patterns have been described, including small cell, spindle cell, giant cell, squamoid, or pleomorphic. They are hypothesized to be lesions that have dedifferentiated from more benign differentiated follicular neoplasms.
Medullary Thyroid Carcinoma
Medullary thyroid carcinoma (MTC) does not originate from the follicular epithelial cells, but from the parafollicular C cells, which are neural crest-derived cells whose function is to produce calcitonin. MTC comprises less that 5% to 10% of all thyroid cancers. Cases are seen sporadically (80%) or in association with familial multiple endocrine neoplasia (MEN IIa, MEN IIb, and pure familial MTC) syndromes. Multifocal and bilateral MTC are usually seen in patients with MEN, but, otherwise, familial and sporadic MTCs are indistinguishable. Grossly, these tumors are well circumscribed and nonencapsulated. Microscopically, tumors can have different appearances, including patterns that mimic other types of thyroid tumors. The most common pattern is of solid growth or nests similar to insular carcinoma. Amyloid, which is present in approximately 80% of cases, is a characteristic feature of MTC. Calcitonin stains are usually positive and specific for MTC, but up to 20% of cases may not stain for calcitonin; therefore, other neuroendocrine markers such as chromogrannin may be useful.
Classification Based on the Capacity to Concentrate Radioactive Iodine
Different pathologic classes of thyroid carcinoma concentrate RAI to various degrees. This characteristic is central to management decisions because the ability to concentrate RAI presents a useful therapeutic target. Table 50.2 presents a classification system that categorizes cancers based on whether they always concentrate RAI, infrequently concentrate RAI, or never concentrate RAI. Papillary and follicular carcinomas (with the exception of the more aggressive variants), usually concentrate RAI. The follicular variant of papillary cancer has the same avidity for RAI as papillary cancer with classic morphology. Therefore, from the treatment standpoint, the distinction between these types of DTCs is not important. The aggressive variants of papillary carcinoma and Hürthle cell carcinoma can concentrate RAI, but to a lesser degree than the classic morphologies. In fact, there is often no measurable iodine uptake in metastases of these tumors. Anaplastic and medullary cancers never concentrate RAI to a clinically useful degree.
TABLE 50.2 A SYSTEM OF CLASSIFICATION FOR THYROID CANCER BASED ON THE ABILITY TO CONCENTRATE RADIOACTIVE IODINE
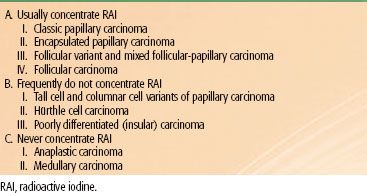
EPIDEMIOLOGY OF THYROID CANCER
Thyroid cancer is the most commonly diagnosed endocrine cancer. There will be an estimated 48,000 new thyroid cancer diagnoses and 1,740 thyroid cancer deaths in the United States by the end of 2011.9 The overall incidence is 7.7 per 100,000 persons per year.10 There is a strong female prevalence with women being three times more likely than men to be diagnosed with thyroid cancer. Thyroid cancer is the fifth most common cancer diagnosis in women, accounting for approximately 5% of all new female cancer diagnoses.9 DTC comprises the overwhelming majority (94%) of new thyroid cancer diagnoses. Approximately 5% are medullary thyroid carcinoma and 1% are anaplastic thyroid carcinoma.11 Per the National Cancer Institute’s Surveillance, Epidemiology, and End Results (SEER) database, the incidence of thyroid cancer has increased in the United States from 3.6 per 100,000 people in 1973 to 8.7 in 2002. The bulk of these cases result from an increase in detection of small primary cancer, with 49% and 87% of new cancers measuring <1 cm and 2 cm, respectively.12 According to another SEER database analysis, the most commonly diagnosed PTC in 2006 was papillary microcarcinoma (<1 cm).13 An even greater proportion of thyroid cancers remain clinically occult, and the prevalence of PTCs at autopsy has been reported as high as 35%.14
The incidence of thyroid cancer increases with age with a peak incidence at 40 to 44 years old for women and 65 to 69 years for men. The peak incidence for ATC is at 60 years for both sexes, and for MTC it can vary depending on whether it is familial or sporadic.15 The incidence of PTC varies significantly across race, with Asian women having the highest incidence of PTC at 10.96 per 100,000 woman-years compared with the lowest incidence for African American women of 4.9 per 100,000 woman-years.10
The prevalence of thyroid cancer in the U.S. population is much higher than the incidence of new diagnoses, a phenomenon attributed to the indolent nature of most DTCs. In 2001, there were an estimated 300,000 individuals living in the United States with thyroid cancer, a number similar to much more commonly diagnosed fatal cancers such as primary carcinomas of the bronchus and lung.15 Patients living with recurrent thyroid cancer comprise a substantial proportion of the prevalence of thyroid cancer. In a series of 1,355 patients with papillary and follicular thyroid cancers, the 30-year cumulative rate of recurrence was approximately 30%, while cancer-related mortality was <10% (Fig. 50.2).16 Studies reporting the outcomes of patients treated for thyroid cancer must be interpreted with consideration of the length of follow-up. Roughly 25% of all recurrences will present after 5 years of follow-up and as many as 16% will be detected after 15 years of follow-up.15
FIGURE 50.2. Tumor recurrence and cancer deaths in 1,355 patients treated for follicular and papillary thyroid carcinoma. (From Mazzaferri EL, Jhiang SM. Long-term impact of initial surgical and medical therapy on papillary and follicular thyroid cancer. Am J Med 1994;97:418–28, with permission from Elsevier.)
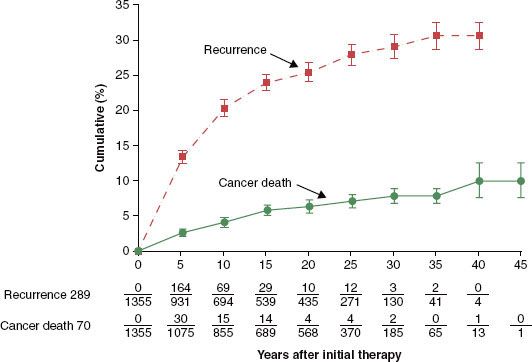
Radiation-Induced Thyroid Cancer
Exposure to ionizing radiation is an established risk factor for thyroid nodules and cancer. Well-recognized data come from survivors of atomic bombs in Hiroshima and Nagasaki and the nuclear reactor accident in Chernobyl.17–18,19–20,21–23 A recent analysis of the 59,687 atomic bomb survivors in the Life Span Study demonstrated a total of 24.5% of cases of thyroid cancer in this cohort were attributed to radiation exposure with a strong dose response.21 Women and young children appeared to be most susceptible. An estimated additional 1,000 cases of thyroid cancer throughout Europe have been attributed to the Chernobyl accident.23 An increased risk of thyroid cancer has also been attributed to exposure to therapeutic radiation in children treated for Hodgkin lymphoma, tinea capitis, enlarged tonsils, and enlarged thymus.24,25–26 Radiation-induced thyroid cancer is pathologically indistinguishable from spontaneous forms of the disease and is not associated with more aggressive biology or poorer prognosis.27,28–30
Familial Medullary Thyroid Carcinoma
Twenty-five percent of cases of MTCs are hereditary. All patients with MTC should be screened for familial disease, and genetic testing is considered the standard of care for all first-degree relatives of patients with newly diagnosed MTC. Heritable forms of MTC are associated with the familial MEN syndromes (MEN IIa, MEN IIb, and pure familial MTC) and have their own unique epidemiology. These disorders are inherited in an autosomal dominant fashion due to a germline-point mutation in the RET gene on chromosome 10q11.2. Men and women are affected equally given the pattern of inheritance. These patients typically present with bilateral and multifocal tumors compared to sporadic forms of MTC. The aggressiveness and age of onset of familial MTC differs depending on the specific genetic mutation. Prophylactic thyroidectomies are increasingly being performed on patients at risk for developing MTC.31
CLINICAL MANIFESTATIONS OF THYROID CANCER
The most common presentation of thyroid cancer is an asymptomatic thyroid nodule found incidentally by the patient, clinicians, or on an imaging study performed for other reasons. Palpable thyroid nodules occur in 3% to 4% of the normal population, and their incidence at autopsy has been reported as high as 50%.32,33–34 However, <1% of thyroid nodules represent a cancer. The differential diagnosis of thyroid nodules includes adenomas (macrofollicular, microfollicular, Hürthle cell, and atypical), cystic lesions (simple cysts or hemorrhagic cysts), colloid nodules, thyroiditis, granulomatous disease, and cancer. Positive findings on history and physical examination that raise suspicion that a thyroid nodule is malignant include rapid growth, firmness, fixation, vocal cord paralysis, cervical adenopathy, stridor, dysphagia, or neurological compromise. In cases where two or more of these symptoms or signs are present, the incidence of thyroid cancer is over 70%, and surgery should be recommended even in the presence of a nondiagnostic fine-needle aspiration (FNA).35
Clinically occult thyroid cancer and benign thyroid nodules will often present on imaging studies performed for other diagnoses. Studies utilizing computed tomography (CT), magnetic resonance imaging (MRI), or ultrasound (US) have reported the incidence of clinically occult thyroid nodules to be 19% to 67%.36 Fluorodeoxyglucose (FDG)-positron emission tomography (PET) studies have also reported the incidence of focal uptake within the thyroid gland at 2% to 3%, with only 25% to 50% ultimately representing cancer.37,38 Many incidentally detected thyroid cancers will ultimately prove to be clinically significant lesions with pathologically identified extrathyroidal extension, lymph node metastases, or both.39
Alternatively, locally advanced thyroid cancer presents with symptoms from involvement of the critical structures intimately associated with the thyroid gland, massive cervical lymphadenopathy, or metastatic disease. These patients present with symptoms such as hoarseness from tumor compression of the recurrent laryngeal nerve, airway compromise, dysphagia or respiratory symptoms, and pain and weight loss resulting from metastatic dissemination.
DIAGNOSTIC EVALUATION OF THYROID CANCER
Laboratory Studies
All patients with thyroid nodules should undergo a serum TSH in addition to basic metabolic, renal, and liver function tests and a complete blood count. Other lab tests, such as Tg, T3, and T4, are commonly obtained but are rarely useful in the initial management of thyroid cancer. Serum calcitonin can be measured during the initial evaluation of patients with MTC and is essential during follow-up for these patients.
TABLE 50.3 ULTRASOUND FINDINGS USED TO SELECT THYROID NODULES FOR FINE-NEEDLE ASPIRATION
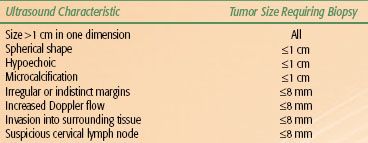
Ultrasound and Ultrasound-Guided Fine-Needle Aspirate
Neck US with Doppler and US-FNA are the standard diagnostic approaches for evaluating thyroid nodules, both palpable and incidentally detected on imaging. The ultrasonographic features used to select thyroid nodules for FNA are shown in Table 50.3. For a more-detailed discussion on the use of US in the evaluation of thyroid nodules, please refer to a consensus statement by the Society of Radiologists in Ultrasound on the management of thyroid nodules detected by US40 and the American Thyroid Association’s recently updated management guidelines for patients with thyroid nodules and differentiated thyroid cancer.41
US-FNA has several benefits in the evaluation of thyroid nodules over other means of obtaining a tissue diagnosis: (a) the technique is minimally invasive and is usually performed as an outpatient procedure; (b) the operator can visually verify that the biopsy needle is in the nodule(s) of suspicion; and (c) it allows for evaluation of nonpalpable nodules and, in the setting of cystic lesions, lowers the rate of inadequate specimens.
US-FNA of thyroid nodules yields cytology that can be broadly classified into four categories: (a) insufficient for diagnosis, (b) benign, (c) positive for cancer, and (d) indeterminate for diagnosis. Approximately 5% to 10% of US-FNA specimens are insufficient for diagnosis, and the rate of insufficient biopsies can be reduced by 50% with repeated biopsies or evaluation by personnel trained in cytological evaluation of thyroid nodules at the time of the procedure. Sixty-five percent to 75% of US-FNA cytology will be benign. Approximately 5% of all US-FNAs of thyroid lesions yield specimens with the diagnostic cytological features of PTC, anaplastic carcinoma, medullary carcinoma, or other poorly differentiated carcinomas. Finally, 15% to 20% of US-FNA will be classified as “suspicious” or indeterminate for diagnosis. Other synonyms for this cytological classification are follicular neoplasm or follicular tumor. The diagnostic evaluation of these lesions is aided by the use of laboratory evaluation of thyroid function and nuclear imaging with RAI uptake studies if the TSH is suppressed. About 20% of these will ultimately show FC or Hürthle cell carcinoma on surgical specimens.42
The overall diagnostic accuracy of US-FNA will depend on the skill and experience of the operator and the cytologist in interpreting the specimen. In a large series of 1,135 cases, there were 1,127 satisfactory samples: 6% cancer (or suspicious for cancer), 10% follicular neoplasms, 82% benign, and 0.7% indeterminate; the false-negative rate was 0% and only 1.5% were false positives. Surgery was performed on 169 patients, and a review of final pathology yielded a sensitivity of 100% and specificity of 67%. The corresponding positive and negative predictive values of US-FNA were 87% and 100%, respectively.43 Another study of 1,613 cases of FNA reported a sensitivity of 78%, specificity of 98%, and a predictive value of 100% for papillary carcinoma.44 The major limitation of this diagnostic tool is the inability to distinguish benign follicular adenomas from FC and follicular PTC.
Cervical US is also an important staging tool because it is effective in identifying lymph node metastases. Cervical lymph node size, presence of calcification, and irregular diffuse intranodular blood flow are the most important US features suggestive of lymph node metastases.45,46 Using criteria based on the ratio of largest to smallest lymph node diameter, nodule echogenicity, intranodal structure, and margin irregularity, the sensitivity and specificity of cervical US for identifying malignant cervical lesions were 90% and 82%, respectively, in a study of 112 patients followed for thyroid cancer by high-resolution US with Doppler.47 Preoperative cervical US identified lymph node metastases that were occult on physical examination in 33% to 39% of patients in a large retrospective series.48,49 Cervical US findings will alter the surgical approach in 14% to 24% of patients with PTC by identifying nonpalpable cervical lymph node metastases.45,49
Computed Tomography and Magnetic Resonance Imaging
CT and MRI commonly detect otherwise clinically occult thyroid nodules. Malignancy is likely with either imaging technique when there are findings of a mass with extrathyroidal extension, lymph node metastases, or both. In this setting, the studies are useful in presurgical planning by establishing the extent of extraglandular tumor and helping the surgeon determine the need for extended neck dissections (Fig. 50.3). MRI is superior to CT for establishing the local extent of a known cancer, because it will more clearly show esophageal or tracheal invasion (Fig. 50.4).50 MRI is indicated in the presence of hoarseness, stridor, dysphagia, or other clinical signs of locally extensive thyroid cancer that cannot be adequately assessed by US.
A major downside to CT is that useful imaging of the neck requires contrast enhancement, which can interfere with subsequent RAI therapy. Because of their high concentration of iodine, CT contrast agents can seriously compromise the effectiveness of therapeutic RAI. When patients receiving iodinated contrast agents require RAI, therapy should be delayed in order to achieve adequate efficacy. Given this drawback as well as the effectiveness of US in defining the local-regional extent of tumor, the authors strongly discourage the use of CT in the staging evaluation of known thyroid cancer. In selected cases, a noncontrasted CT of the chest may be indicated to evaluate the presence of mediastinal and lung metastases, but, generally speaking, it is not necessary to perform a CT before primary therapy of most DTCs.
Nuclear Medicine Studies
The four major nuclear medicine studies related to thyroid cancer evaluation with iodine isotopes are radioactive iodine uptake (RAIU), thyroid scan, diagnostic whole-body scan (DxWBS), and posttreatment whole-body scan (RxWBS). Table 50.4 summarizes each of these studies.
RAIU tests are used to quantify the RAI-concentrating ability of remnant thyroid tissue after thyroidectomy. This value is required by federal regulation for outpatient I-131 therapy.
Thyroid scans produce images of the thyroid’s ability to concentrate RAI and have a historical use in the evaluation of thyroid nodules, characterizing them as functional (“hot”) or nonfunctional (“cold”). The authors do not use thyroid scans in the workup or management of patients with thyroid cancer.
The typical DxWBS involves a total body image several days after administering approximately 3 mCi of I-131. The current value of a DxWBS in thyroid cancer management is controversial. Some experts use a DxWBS to determine the dose of I-131 in a patient being treated for thyroid cancer. Some experts use a DxWBS as part of the surveillance program following initial treatment. A comprehensive discussion of the issues related to DxWBS is beyond the scope of this chapter. The main arguments against doing DxWBS are low sensitivity, stunning of residual cancer cells, and unnecessary radiation exposure. The bottom line is that reasonable people will disagree about the role of DxWBS in thyroid cancer management. The authors do not use DxWBS to stage or monitor thyroid cancer patients and have not ordered a DxWBS in over 5 years.
FIGURE 50.3. A patient presenting with a neck mass. A: Computed tomography study showing the mass to be due to a primary papillary thyroid carcinoma coming into contact with a related level 4 metastatic lymph node (arrows). The lymph node at level 4 and in the tracheoesophageal groove is shown by the arrowheads. B: A section more superiorly shows level 3 adenopathy that is multicystic in a pattern typical but not necessarily diagnostic of papillary thyroid carcinoma. There is upper level 4 cystic adenopathy with an enhancing mural nodule (arrow)—a morphology suggesting of thyroid carcinoma. A contralateral enhancing positive node with a focal low-density defect (arrowhead) is also present. (From Mancuso AA, Mendenhall WM, Vaysberg M. Thyroid: nodules and malignant tumors. In: Mancuso AA, ed. Head and neck radiology. Philadelphia: Lippincott Williams & Wilkins, 2010:1457–1481, with permission.)
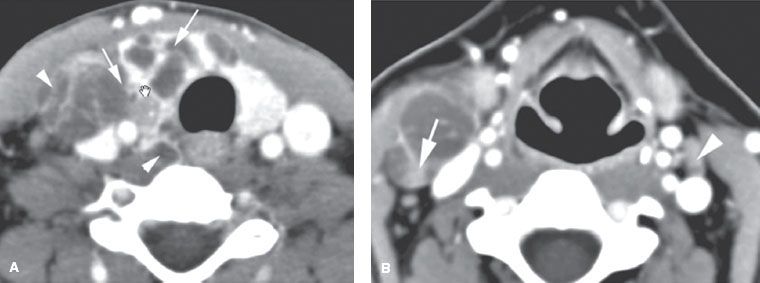
FIGURE 50.4. A patient demonstrating the value of magnetic resonance in the detection of invasion of the trachea and cervical esophagus, especially as it pertains to treatment planning. This patient has anaplastic carcinoma of the thyroid gland. In A: T1-weighted images show distortion of the trachea (arrow) and esophagus (arrowhead), but it is difficult to determine whether invasion is present. In B: the T2-weighted image shows obvious growth of tumor replacing the normal signal of cartilage of the trachea with growth on both sides of the cartilage (arrow) as well as replacement of the normal muscular wall signal of the cervical esophagus (arrowhead), indicating esophageal wall invasion. (From Mancuso AA, Mendenhall WM, Vaysberg M. Thyroid: nodules and malignant tumors. In: Mancuso AA, edr. Head and neck radiology. Philadelphia: Lippincott Williams & Wilkins, 2010:1457–1481, with permission.)
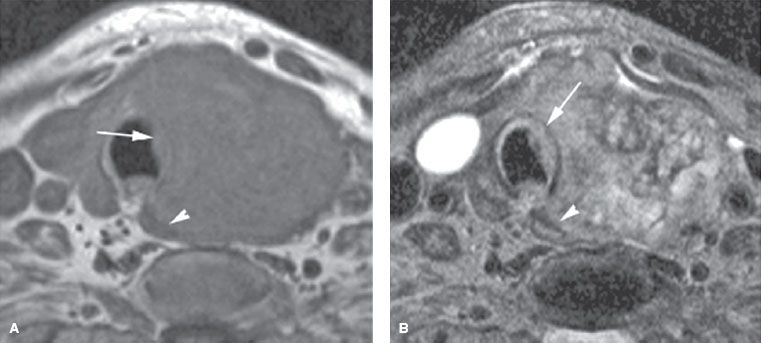
An RxWBS should be done in every patient who receives a therapeutic dose of RAI. The optimal interval between administration and scan in most patients is approximately 7 days. The primary purposes of an RxWBS is to detect gross residual disease in the regional lymphatics or in distant sites and to determine if known disease concentrates RAI.
FDG-PET is another nuclear medicine study that is commonly used to detect metastatic disease, but the predictive value of PET in thyroid cancer is not well defined. TSH stimulation increases the accuracy of FDG-PET in most types of DTC. A prospective trial comparing FDG-PET studies during thyroid hormone suppression to studies after administration of rhTSH showed increased detection of occult metastases in the stimulated studies.51 Some insurers will not reimburse for FDG-PET for thyroid cancer without prior documentation of follicular-derived thyroid cancer with a suppressed or stimulated Tg over 10 ng/mL and a negative DxWBS.41
When FDG-PET is performed for reasons other than thyroid cancer and reveals a hypermetabolic thyroid nodule, a biopsy is warranted in most cases as these lesions carry a 15% risk of malignancy.50 A recent study of 5,877 patients who received FDG-PET for cancer screening or staging or restaging purposes, 3.7% revealed hypermetabolic activity within the thyroid gland. Of the patients sent for US-FNA, 14% were diagnosed with thyroid malignancies.52 A larger review of pooling data from over 55,000 FDG-PET studies reported a 1% prevalence of focal thyroid gland abnormalities, and the rate of confirmed thyroid malignancies in this group was as high as 33%.53
TABLE 50.4 NUCLEAR IMAGING STUDIES USED IN THE MANAGEMENT OF DIFFERENTIATED THYROID CARCINOMAS
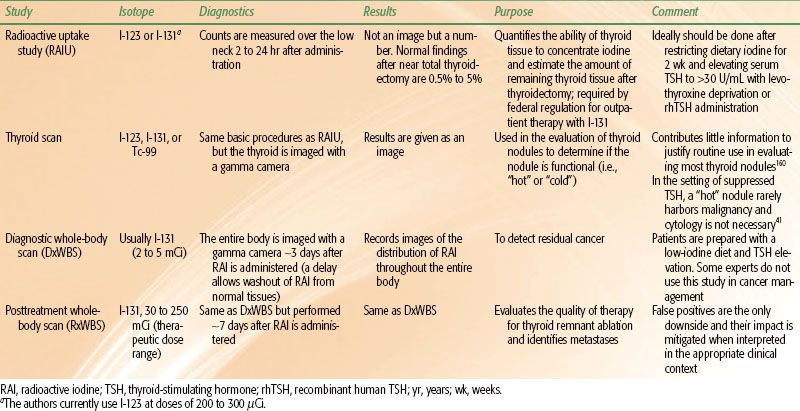
PROGNOSTIC FACTORS
The most important prognostic factor for disease recurrence and cancer mortality is the histologic classification. DTC, when diagnosed in an early stage, has a favorable prognosis. However, tall-cell variant can have a 10-year mortality of up to 25%.54 Hürthle cell carcinoma carries a relatively poor prognosis, with a 25% rate of metastatic disease55,56 and decreased survival at 10 years of 76%, compared with 93% and 85% for PTC and FC, respectively.57 Columnar cell variant and diffuse-sclerosing variants likewise carry a poor prognosis relative to other forms of DTC. Contrarily, follicular-variant PTC and most FC share the same favorable prognosis as classic PTC relative to age and stage at diagnosis. ATC has an abysmal prognosis. All ATCs are considered stage IV under the current AJCC system and the overall 5-year survival is approximately 5%.1
Within the classification of DTC there are several important prognostic factors, including age, gender, family history, tumor size, extrathyroidal extension, vascular invasion, cervical and mediastinal lymph node metastases, degree of cytologic atypia and tumor necrosis, presence of distant metastases, and the degree to which the cancer can concentrate RAI. The prognostic variables are summarized in Table 50.5 and several of these factors are discussed in detail below.
Age
Age is a major prognostic variable that is accounted for in the current and previous AJCC staging systems (Fig. 50.5). The current AJCC system dichotomizes staging for DTC based on age under 45 years versus 45 years and older. In the current system, patients under 45 years of age with any T or N stage (based on tumor-node-metastasis [TNM] classification) are considered overall stage I, unless metastatic disease is present, which elevates the overall stage to stage II. Conversely, patients 45 years of age and older are given an overall stage I and II only if their T and N stages are T1N0 and T2N0, respectively. Thereby, this system considers age to be one of the most important prognostic variables. A limitation of this system is that it fails to account for the poor prognosis seen in patients diagnosed at a young age (<15 years old). Children present with more advanced tumors than young adults and have more recurrences and distant metastases.58,59 In one series of 56 children (ages 4 to 20 years) with PTC or FC, the 10-year progression-free survival was only 61%, although survival remained favorable at 98%.60 Another series of 21 children (ages 4 to 15 years) reported a 24% rate of distant metastases at presentation.61
Gender
Compared to women, men who develop DTC are diagnosed at an age approximately 20 years older, are twice as likely to develop distant metastases, and are 30% more likely to have regional metastases.62,63 It is unclear why there is a gender bias toward increasing age at diagnosis for men, but it may be attributed to delayed access to the U.S. health care system for men. Delaying the diagnosis for more than 12 months is associated with a doubling in the chance of thyroid cancer mortality.16
Primary Tumor Size
A linear relationship exists between primary tumor size and cancer-specific mortality.16,62,64,65–66 Very large tumors are associated with increased rates of extrathyroidal disease, lymph node and distant metastases, less-favorable histologies, and, in some cases, unresectable disease, all of which harbor a poor prognosis. Papillary microcarcinoma (<1 cm) have a disease course characterized by exceedingly favorable outcomes. These cancers, often found incidentally during surgery for benign thyroid conditions, are considered clinically insignificant malignancies and generally do not portend to decreased survival. Recurrences after total thyroidectomy in some series are nearly zero. Some small tumors, however, are associated with other poor prognostic factors that warrant more aggressive management. Lymph node metastases occur in as many as 20% to 60% of cases with small tumors; 30% to 40% can be multifocal, and 17% to 30% will have extrathyroidal extension.67–68,69 These outcomes have led some investigators to argue that treatment for papillary microcarcinoma should be no different from that for PTC larger than 1 cm in size, advocating for the routine use of RAI.70 The authors agree with other investigators that additional prognostic factors such as extrathyroidal extension, lymph node metastases,71 age, and multifocality should drive the decision to treat with adjuvant RAI when managing papillary microcarcinoma.
TABLE 50.5 PROGNOSTIC FACTORS IN DIFFERENTIATED THYROID CANCER
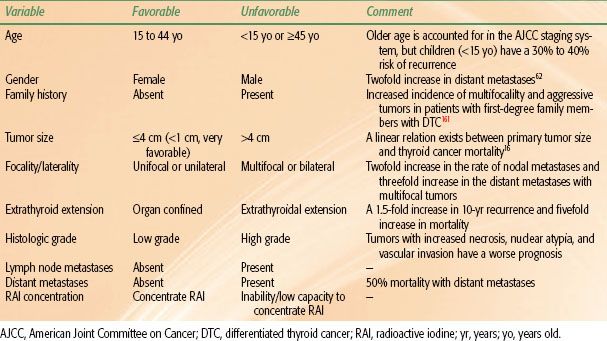
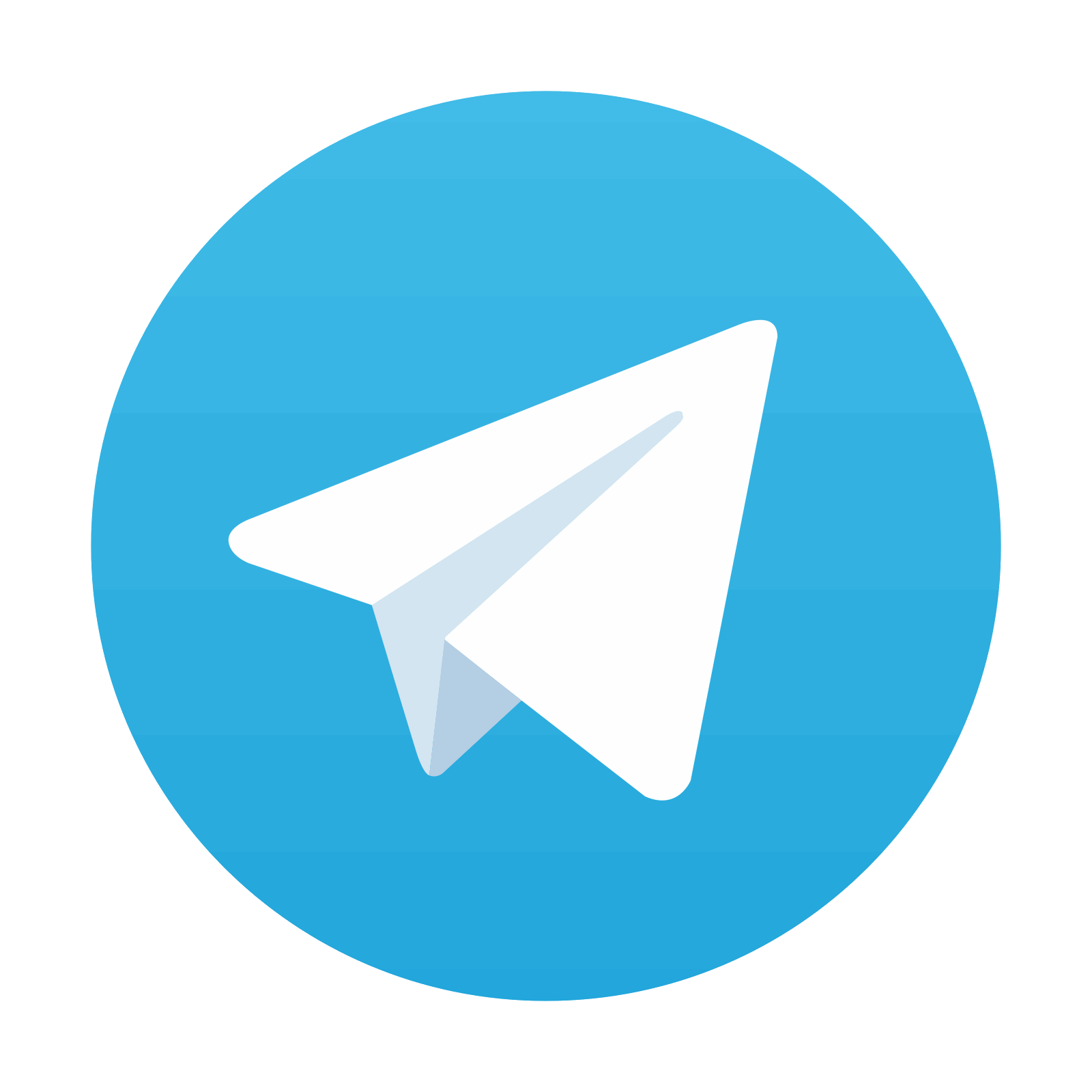