Thrombopoietin
Kenneth Kaushansky
Ian Hitchcock
HISTORY
The term erythropoietin (EPO) was coined at the turn of the last century to describe the humoral substance responsible for red cell production. At that point in time, blood platelets were barely distinguishable in the best microscopes, prompting their dismissal as “the dust of the blood.” However, the pioneering work of Carnot, Wright, and others in the early 20th century defined the critical role of blood platelets in coagulation and their origin from the marrow megakaryocyte, ultimately prompting the Hungarian physician Keleman to coin the term thrombopoietin (TPO) to describe the humoral substance responsible for the recovery of platelet levels following thrombocytopenia.1
In the mid-1960s, several groups set out to purify TPO from the plasma of thrombocytopenic animals. At the time, the only reliable assay on which to base a purification strategy was the35S uptake assay, in which radioactivity incorporated into blood platelets of injected mice or rats served as a measure of thrombopoietic activity. This in vivo assay proved very cumbersome; the assay was too insensitive, costly, and time-consuming to be practical, and little progress was made. With the availability of in vitro megakaryocyte differentiation assays in the 1980s, additional purifications were attempted; nevertheless, although the availability of what was thought to be partially purified TPO helped to define its expected biologic properties,2,3,4 none of the initial purification efforts led to amino acid determination or cloning of the molecule.
Occasionally in science, a finding from one field, although important in itself, can have a profound, catalytic effect on a seemingly unrelated area of research. The discovery and characterization of the murine myeloproliferative leukemia virus had such an influence on the search for TPO. The virus causes an acute myeloproliferative syndrome in infected mice.5 In 1990, the responsible oncogene was cloned, and the protooncogene obtained 2 years later.6,7 Based on the predicted presence of four spatially conserved cysteine residues and a juxtamembrane pentapeptide sequence (Trp-Ser-Naa-Trp-Ser), it was immediately evident that the cellular gene was a member of the hematopoietic cytokine receptor family,8 which includes the receptors for EPO, the granulopoietic factors interleukin-3 (IL-3), granulocyte colony-stimulating factor (G-CSF), and granulocyte macrophage colony-stimulating factor (GM-CSF), and multiple lymphokines. However, in 1992 its ligand was unknown; c-mpl encoded an orphan receptor. Based on the origin of the c-Mpl cDNA, the bipotent erythroid/MK cell line HEL,9 and on antisense c-mpl knock-out experiments demonstrating a significant reduction in megakaryocytes,10 many groups postulated that the Mpl ligand might be identical to TPO.
THE CLONING OF THROMBOPOIETIN
While progress in understanding megakaryocyte biology and in purifying TPO was being made using the c-Mpl receptor, others had developed improved bioassays for TPO and continued to make efforts using conventional purification strategies. Using a modified in vivo assay in which the polyploidy of marrow megakaryocytes from injected rats was examined, Kuter et al.11 performed an 11-step purification of ovine TPO, although the publication of this work did not report the cloning of the corresponding cDNA. In contrast, using an in vitro megakaryocyte assay, the scientists at Kirin Pharmaceuticals in a 12-step conventional purification scheme obtained sufficient plasma TPO from thrombocytopenic rats to obtain amino acid sequence and cloned cDNA for rat and then multiple species of the protein, including the human hormone.12
Using the c-Mpl protooncogene product coupled to affinity matrices, scientists at Genetech and at AMGen obtained microgram quantities of porcine and canine TPO, respectively, allowing their amino acid sequencing and cDNA cloning.13 In contrast to the biochemical purifications utilized by these four groups, an expression cloning strategy was used by Lok et al.14 to obtain cDNA for murine and then human TPO. In this approach a hematopoietic cell line, BaF3, that requires IL-3 for survival and proliferation, was engineered to express the c-Mpl protooncogene. In this way, if the c-Mpl ligand was present in the culture, the cells should remain viable and potentially proliferate after withdrawal of IL-3. The BaF3/mpl cells were chemically mutated and autonomously growing sublines were obtained; although most such sublines were producing their own IL-3, others were producing the c-Mpl ligand. This subline was used as source for cDNA library construction and an additional functional cloning strategy. Remarkably, the cDNA obtained from the above four groups, three of them cloned nearly simultaneously, all encoded the same polypeptide (except for species differences). Initial in vitro experiments using the corresponding recombinant proteins demonstrated the effect of each of these substances on megakaryocyte maturation, and injections into normal mice resulted in massive increases in peripheral blood platelet counts and marrow megakaryopoiesis. With these efforts TPO was finally cloned.
STRUCTURE OF THROMBOPOIETIN
The cloned human TPO cDNA encodes a polypeptide of 353 amino acids including the 21-amino acid secretory leader sequence.15 The mature protein can be conveniently divided into two domains: the amino-terminal 154 residues bear striking sequence homology with EPO and bind to the mpl receptor; the
carboxyl-terminal domain bears no resemblance to any known proteins, but contains multiple sites of both N– and O-linked carbohydrate. This latter feature accounts for the large discrepancy between the predicted and actual molecular weight of the protein; approximately half of the 70-kDa TPO molecule is carbohydrate.
carboxyl-terminal domain bears no resemblance to any known proteins, but contains multiple sites of both N– and O-linked carbohydrate. This latter feature accounts for the large discrepancy between the predicted and actual molecular weight of the protein; approximately half of the 70-kDa TPO molecule is carbohydrate.
Based on its amino acid sequence, and like its closest homologue EPO, TPO is predicted to fold into a four-helix bundle protein. This prediction was recently confirmed with solution of the tertiary structure of the receptor-binding domain of TPO in complex with an anti-TPO monoclonal antibody.16 Comparisons of the two proteins reveal striking similarities, but also some critical differences, likely accounting for the failure of the two hormones to cross react with the receptors for the other hormone.17
As noted, TPO can be conveniently divided into two domains based on homology to EPO; expression of a truncated version that represents the domain most homologous to EPO revealed that this amino-terminal half of the molecule is responsible for binding to and stimulation of the Mpl receptor.18 However, the circulatory half-life of the isolated aminoterminal fragment is substantially shorter than the full length molecule, unless it is modified with polyethylene glycol.19 In contrast to the stimulatory activity of the amino-terminal domain, the carboxyl terminal domain of TPO acts to enhance secretory efficiency of the protein; the level at which the isolated amino-terminal fragment is expressed from cells that normally produce TPO is reduced about 5- to 10-fold compared to the secretory level of the full length molecule.20,21,22 Whether this is due to enhanced folding of the polypeptide, protection from intracellular proteolysis, or other mechanisms is presently under study.
Several investigators have attempted to ascertain the sites on TPO responsible for binding to its receptor. Using site-directed alanine scanning mutagenesis to study the functional activities of 40 solvent-exposed residues of the protein, monoclonal antibody epitope mapping, and a phage display-binding assay system, Pearce et al.23 determined that the residues vital for hormone binding to c-Mpl are located in the first and fourth predicted α-helices of the molecule and the segment connecting the first and second helices, locations previously shown to be important for functional interactions in several other cytokine/receptor systems.24 Subsequently, Jagerschmidt produced 20 TPO mutants and compared the mutants’ ability to induce the proliferation of a c-Mpl-expressing cell line, M-07e, with that of wild-type TPO. Among the mutations that substantially reduced biologic activity, three were found located in the A helix of the protein (Arg10, Lys14 and Arg17) and four in helix D (His133, Gln132, Lys138, and Phe141). Moreover, they found that Arg10 and Arg17 seem to be specific determinants for TPO/c-Mpl recognition, although Pearce et al. failed to find any reduction in binding affinity of an Arg10 to Ala mutation. As these residues in EPO are Arg and Leu, respectively, the latter residue could help to explain the failure of the two cytokines to cross-react in receptor binding assays.17
THE REGULATION OF THROMBOPOIETIN EXPRESSION
As would be expected for the physiologic regulator of platelet production, plasma concentrations of TPO vary inversely with the platelet count in patients with marrow failure.25 Northern blot analyses of multiple organs reveal that TPO is widely expressed, mRNA being present at highest levels in the liver of normal animals, but the kidney, smooth muscles, and a number of other organs also express transcripts for the hormone.13,14,15,16,17,18 Based on the capacity of platelets to adsorb the hormone from its surroundings, internalize, and destroy it, Kuter and Rosenberg26 and other investigators27 have proposed that the regulation of TPO levels in plasma is dependent entirely on platelet numbers; in the presence of thrombocytosis, the steady-state level of hormone production is overwhelmed by platelet-mediated TPO removal and levels are low, and in states of thrombocytopenia, little of the same amount of TPO production is adsorbed, allowing levels to rise in order to stimulate thrombopoiesis. Initial findings of relatively low TPO levels in patients with immune thrombocytopenia (ITP) were explained by further postulating that the megakaryocytic hyperplasia seen in such states then accounts for the increased TPO adsorption. Ancillary data for this assertion come from the tpo hemizygous mouse28; loss of one allele of tpo leads to a 40% reduction in platelet counts. If active regulation of TPO exists, then increased expression from the second, normal allele should have corrected the reduction in hormone levels and reestablished a normal platelet count, much like what occurs in the EPO system.
These arguments not withstanding, a growing body of evidence suggests that additional mechanisms operate to affect TPO expression. At baseline, it is very difficult to detect specific mRNA in marrow cells. However, transcript levels can be substantially induced in marrow stromal cells in response to thrombocytopenia,29,30,31,32 albeit at platelet levels lower than the 60% found in the tpo hemizygous mice. In addition, a number of inflammatory states are associated with TPO levels above that expected for their platelet count33,34,35 although this finding has not been universal.36 It has been shown that IL-6, a well known inflammatory response mediator, increases TPO production both in vitro and in vivo,37,38,39 potentially helping to explain the enhanced levels seen in inflammatory and possibly other pathologic states.
The mechanism by which TPO production is regulated is coming under increased investigation. Using platelet granule proteins, Sungaran et al.40 determined that the platelet α granule proteins PDGF-BB and FGF2 stimulated, and that platelet factor four, thrombospondin, and transforming growth factor-β inhibited TPO production from cultures of marrow stromal cells; on balance, whole platelet extracts suppressed TPO production. More recently, platelet granule proteins were shown to transcriptionally repress TPO mRNA expression in a marrow stromal cell line as well as in primary bone marrow stromal cell cultures. Like TPO mRNA, secretion of TPO protein was also suppressed by serum. Reporter gene constructs indicate that DNA elements located in an approximately 1.9 kb region between 250 bp upstream of the transcriptional initiation site and the middle of the second intron are able to mediate this transcriptional repression.41 Thus, accumulating evidence indicates that marrow stromal cells can be induced by exogenous stimuli to produce TPO and help explain the response to inflammatory stimuli.
The TPO gene displays an unusual 5′ untranslated region structure. Unlike the majority of genes that initiate translation with the first ATG codon present in the mRNA, TPO translation initiates at the eighth ATG codon in the transcript, located
in the third exon of a full-length mRNA (there are two transcription initiation sites that differ because of alternate splicing of the first exon).42 As the eighth ATG is out of frame but embedded in the short open reading frame (ORF) of the seventh ATG, translation initiation is quite inefficient.43 Thus, under normal circumstances, little TPO protein is produced for any given amount of mRNA. Although it is not yet certain whether this molecular arrangement has physiologic consequences (i.e., it can be differentially regulated), it is now clear from patients with familial essential thrombocythemia (ET) that mutation of the TPO gene in noncoding sequences can lead to enhanced translation efficiency and thrombocytosis. Several cases of autosomal dominant familial thrombocytosis have been linked to mutations in the region surrounding the initiation codon. In two families, a single mutation in different nucleotides of the intron three splice donor sequence results in alternate splicing of the primary transcript, eliminating the seventh and eighth ATG codons and creating a new aminoterminus by fusing of the fifth ORF with the hormone coding sequence. This novel TPO mRNA is efficiently translated resulting in supraphysiologic levels of hormone production and nonclonal expansion of thrombopoiesis.44,45 In another mutant Tpo allele deletion of a single nucleotide within the seventh ORF leads to its fusion with the TPO coding sequence and now enhanced translation of TPO from the seventh ATG codon.46 Finally, another mutation within the seventh ORF leads to its premature termination, preventing its interference with translation initiation from the usual eighth initiation codon47 again enhancing protein production. The molecular mechanisms of diseases of mRNA translation have been summarized.48
in the third exon of a full-length mRNA (there are two transcription initiation sites that differ because of alternate splicing of the first exon).42 As the eighth ATG is out of frame but embedded in the short open reading frame (ORF) of the seventh ATG, translation initiation is quite inefficient.43 Thus, under normal circumstances, little TPO protein is produced for any given amount of mRNA. Although it is not yet certain whether this molecular arrangement has physiologic consequences (i.e., it can be differentially regulated), it is now clear from patients with familial essential thrombocythemia (ET) that mutation of the TPO gene in noncoding sequences can lead to enhanced translation efficiency and thrombocytosis. Several cases of autosomal dominant familial thrombocytosis have been linked to mutations in the region surrounding the initiation codon. In two families, a single mutation in different nucleotides of the intron three splice donor sequence results in alternate splicing of the primary transcript, eliminating the seventh and eighth ATG codons and creating a new aminoterminus by fusing of the fifth ORF with the hormone coding sequence. This novel TPO mRNA is efficiently translated resulting in supraphysiologic levels of hormone production and nonclonal expansion of thrombopoiesis.44,45 In another mutant Tpo allele deletion of a single nucleotide within the seventh ORF leads to its fusion with the TPO coding sequence and now enhanced translation of TPO from the seventh ATG codon.46 Finally, another mutation within the seventh ORF leads to its premature termination, preventing its interference with translation initiation from the usual eighth initiation codon47 again enhancing protein production. The molecular mechanisms of diseases of mRNA translation have been summarized.48
MEGAKARYOPOIETIC ACTIVITIES OF THROMBOPOIETIN IN VITRO
As initially defined, TPO was thought to be a potent megakaryocyte differentiation factor but was not thought to influence cellular proliferation. However, as soon as recombinant protein was available it was established that in contrast to the partially purified samples thought to contain the hormone, TPO clearly acts to induce proliferation of megakaryocytic progenitor cells,49 and in synergy with other cytokines, to support the proliferation of multiple types of immature hematopoietic progenitor cells.50,51,52,53 From the initial studies of its biologic properties, it has been clear that TPO is a potent megakaryocyte colony-stimulating factor. Approximately 75% of a maximal number of megakaryocyte colony-forming cells can be induced to grow using optimal levels of TPO alone.49,54 A number of other cytokines and hormones, including IL-3, kit ligand, EPO, and IL-11 also act in synergy with lower levels of TPO to promote megakaryocyte colony formation.55 However, the hormone appears to exert its proliferative effect primarily on progenitors of limited developmental potential. The number of megakaryocytes present in each colony is greater for those that form in the presence of IL-3 or kit ligand, either alone or together with TPO, than in the presence of TPO alone. However, in the absence of the hormone, IL-3- or kit ligand-induced megakaryocyte formation is aborted; the cells display low ploidy values and poor cytoplasmic maturation.54
Serum-free suspension culture systems have been most useful for demonstrating the differentiative effects of the hormone on megakaryocytes. Using the recombinant protein several groups have established that TPO increases megakaryocyte cell size, shifts the cells to much higher ploidy classes, and enhances the expression of the lineage specific markers glycoprotein (gp)Ib and gpIIb/IIIa.49,56,57,58 When examined under the electron microscope, TPO-induced megakaryocytes display prominent membrane production and numerous platelet specific granules, and the cytoplasm demarcates into regions destined to fragment into mature platelets.49,54,56 When cultured slightly longer, especially in the presence of plasma, the cells form “proplatelet processes,” and fragment into platelets that appear to function normally upon exposure to classic platelet agonists.59 Moreover, this final process appears to be independent of TPO, instead being more dependent on a substance in normal plasma. One report suggests that the activity in plasma responsible for platelet formation is a complex of thrombin and antithrombin III.60 At present, this report remains unconfirmed, so that the nature of a (regulatable) stimulus (if there is one) for the final stage of platelet formation is presently unclear.
One relatively consistent property of hematopoietic growth factors has been that the cytokine or cytokines that stimulate the production of a given cell lineage also activate or “prime” the resulting mature blood cells for activation in response to other signals.61,62,63 Like that for IL-3, GM-CSF and G-CSF in vitro studies indicate that although incubation in the hormone leads to the priming of platelets to respond to an otherwise subthreshold level of classical platelet agonists,64,65,66 TPO does not lead to frank platelet activation.
THROMBOPOIETIN AND THE HEMATOPOIETIC STEM CELL
Analogous to the lineage specific characteristics of EPO, the biologic properties of TPO were initially thought to be restricted to cells of the megakaryocyte lineage. Nevertheless, early studies of the biologic activities of the hormone included the stimulation of sorted CD34+ cells.56 As this population of cells includes megakaryocytic progenitors, it remained formally possible that the hormone was lineage restricted until two groups demonstrated that sorted marrow cells highly enriched in hematopoietic stem cells (HSCs) also survived in the presence of TPO alone, and proliferated in synergy with IL-3 or kit ligand.52,53 Shortly after these in vitro findings were reported, genetic elimination of c-Mpl was found to result in a significant reduction in the number of hematopoietic progenitors of all lineages67 and then using competitive repopulation, Solar et al.68 demonstrated that the number of HSCs were reduced seven to eightfold in c-Mpl null mice. As it became appreciated that the congenital form of amegakaryocytic thrombocytopenia are due to a homozygous or mixed heterozygous mutation in the c-Mpl receptor locus, the role of TPO on stem cells has been extended to humans, as nearly all such children develop aplastic anemia due to dwindling numbers of stem cells within the first 2 to 5 years of life.69,70,71,72 Consistent with this notion, we demonstrated that the self-renewal/expansion of HSCs is also vitally dependent on TPO, as transplantation of normal stem cells into Tpo null mice results in a 15- to 20-fold reduced level of stem cell expansion.73 Thus, it is now clear that TPO joins kit ligand as the only cytokine that plays a nonredundant role in stem cell survival, self-renewal, and proliferation.
IN VIVO ACTIVITIES OF THROMBOPOIETIN
The effects of exogenous TPO on the kinetics of platelet production have been studied extensively. As would be expected from its megakaryopoietic effects in vitro, when TPO is administered to animals or people, the platelet count begins to rise after 3 to 5 days, depending on the dose and the recipient species.13,14,18,74,75,76,77,78,79 These results indicate that TPO does not cause the immediate release of platelets from megakaryocytes, consistent with its failure to accelerate fragmentation of proplatelet processes, the long exvaginations of megakaryocyte cytoplasm felt to represent the most immediate platelet precursor.59 Instead, TPO stimulates the development and maturation of new megakaryocytes. Once the size and numbers of megakaryocytes increase, the platelet count begins to rise until a new steady-state level is reached, the height of which depends on a log-linear relationship with the administered dose.19 Due to a prolonged biologic effect (the result of its slow plasma clearance and action on both primitive and mature hematopoietic cells), enhanced platelet production resulting from parenteral administration of TPO occurs 6 to 16 days after a steady state level of the hormone is achieved.79,80
Given the panhematopoietic effects of TPO in vitro, careful attention was paid to all of hematopoiesis in animals administered the recombinant hormone; the in vivo effects of TPO were found to correlate with its in vitro properties. Administration of the hormone to either normal or myelosuppressed animals increased the numbers of all types of hematopoietic progenitor cells,81 and genetic elimination of Tpo or its receptor causes a 65% to 95% reduction in number of transplantable stem cells and hematopoietic precursors of multiple lineages.67 Thus, TPO should be thought of as a lineage-dominant panhematopoietic cytokine that plays multiple roles in blood cell production.
As noted above, a large body of evidence indicates that cytokines that stimulate hematopoietic cell production also activate the corresponding mature cell type. If this were also true for TPO, platelet activation and thrombosis could result. In vitro studies have indicated that the hormone primes platelets to aggregate in the presence of classical agonists, but does not, by itself, lead to platelet activation.65 Studies performed in vivo are consistent with this conclusion; the administration of TPO to nonhuman primates19 or humans did not result in de novo platelet activation,78 and an excess of thrombotic complications has not been seen in multiple clinical trials of two forms of the hormone (see below). Nevertheless, these clinical trials have all avoided enrolling individuals with any history of pathologic thrombosis, as has the administration of FDA approved small molecule thrombopoietic agonists.
Despite this increased understanding of the vital role of TPO on thrombopoiesis, a remaining major question is what is responsible for the approximately 10% residual platelet formation present in mice and humans in which Tpo or its receptor are genetically altered. Based on the megakaryopoietic activities of IL-3, IL-6, IL-11, GM-CSF, and leukemia inhibitory factor, both in vitro and in vivo, in an attempt to identify the cytokine(s) responsible for residual platelet production in Mpl-/- mice, mice genetically deficient in IL-3, IL6-R, IL-11-R, or the combined signaling subunit of the IL-3, GM-CSF, and IL-5 receptors (βC) were crossed to Mpl null animals; none of these double to quadruple cytokine/cytokine receptor deficient mice displayed more severe thrombocytopenia than found in the Mpl-/- mouse.82,83,84,85 Whether another known (or possibly unknown) cytokine is responsible for the residual platelet production in the absence of TPO function, or as was suggested, this combination of the chemokine SDF-1, which displays a synergistic action on megakaryocyte growth86 and endothelial cells.87
THE Mpl RECEPTOR AND MOLECULAR MECHANISMS OF TPO ACTION
The initial step in hematopoietic growth factor action is binding to its specific, cell-surface receptor. Recent studies of the response of many of these receptors have begun to provide molecular insights into cytokine-induced signaling. It is clear that protein tyrosine phosphorylation of the receptor itself, and several substrates is an integral part of the cellular response to hematopoietic growth factor stimulation, as protein tyrosine kinase inhibitors block the growth induced by many hematopoietic cytokines, and phosphatase inhibitors have the opposite effect.88,89 However, the TPO receptor does not display any enzymatic activity; rather, cytokine receptors recruit cytoplasmic kinases to activate signaling molecules. The EPO receptor was the first member of the hematopoietic cytokine receptor family shown to recruit and activate a member of the Janus family of cytoplasmic tyrosine kinases (JAKs). Subsequent work has shown that JAK kinases mediate much of the secondary protein tyrosine phosphorylation triggered by the binding of hematopoietic growth factors to their corresponding receptors.90,91 The role of other cytoplasmic kinases, such as src and the srcrelated kinases, in growth factor-induced signaling is also under active investigation.92,93 Although many of the signals involved in thrombopoietic cytokine action are rapidly being identified, major challenges lie ahead in deciphering the molecular basis of signal specificity. For example, the cellular response generated upon TPO binding is distinct from that originating at the IL-3 receptor, but many of the same molecules are activated by both receptors; a thorough analysis of the specific patterns of signaling intermediates activated by each of the cytokines that act directly on megakaryocytes will be required to more fully understand the role played by each during thrombopoiesis.
Progress has been rapid in deciphering the signaling pathways employed by TPO. Because of its homology with EPO, and between c-Mpl and the rest of the cytokine receptor family, initial studies of TPO signal transduction concentrated on the JAK and signal transducers and activators of transcription (STAT) families of proteins. The first reports focused on leukemic cell lines that naturally express the c-Mpl receptor, or ones engineered to do so. These studies identified JAK2 and TYK2 as the immediate kinases that bind to c-Mpl and become activated on exposure to TPO.94,95,96,97,98,99,100,101,102 Other proteins reported in various megakaryocytic cell lines to be phosphorylated in response to TPO include STAT3 and STAT5,94,95,100,103,104 the adapter proteins Grb2,100,101 Cbl,101 and son of sevenless (SOS),101 Shc and its related phosphatase SHIP,95,100,101,105 phosphoinositol-3-kinase (PI3K) and its downstream kinase Akt,64,106,107 an inhibitory transcription factor downstream of Akt, FKHRL-1,108 the Raf-1/MAP kinase,100,109,110 and hematopoietic cytokine receptorrelated phosphatases such as SHPTP-2.108
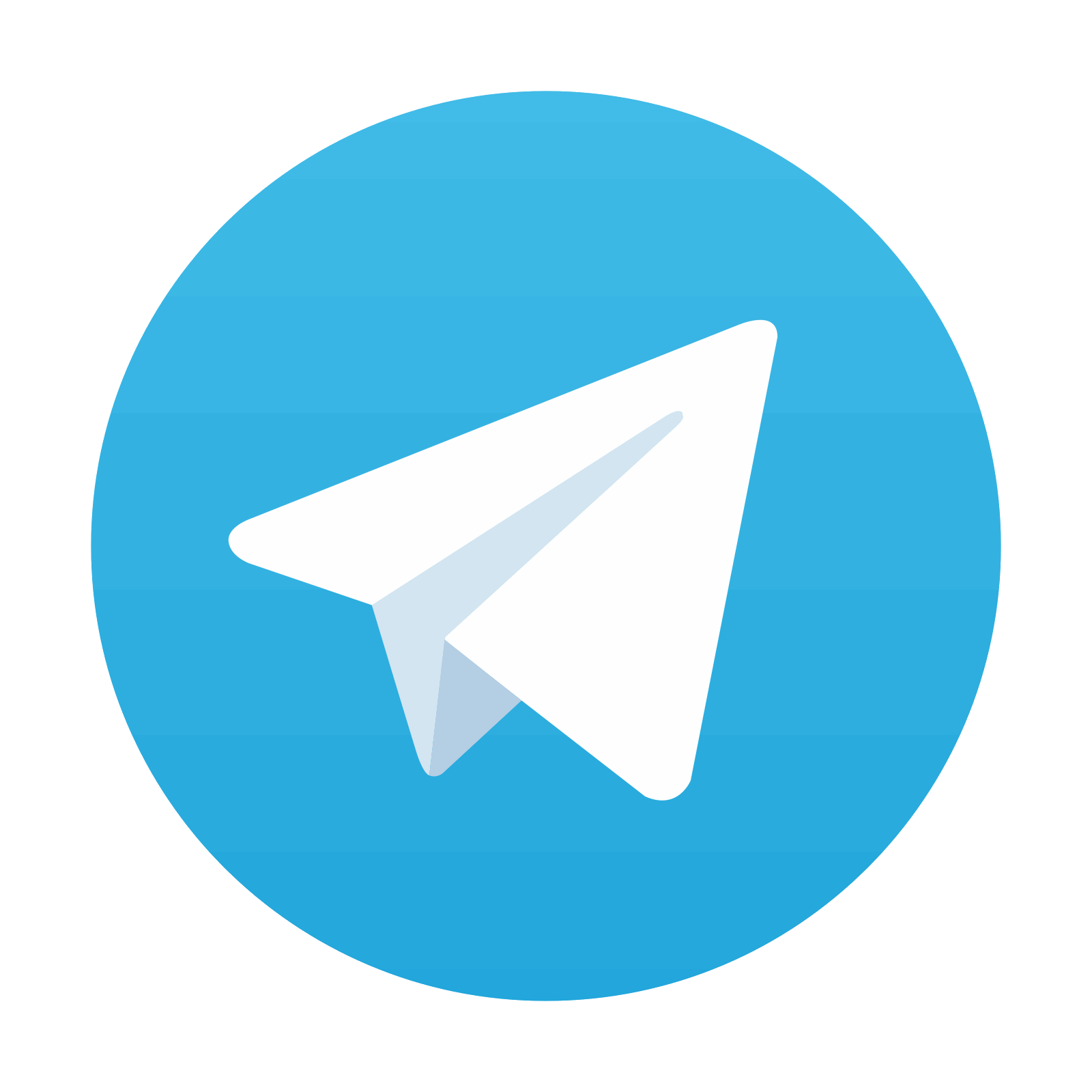
Stay updated, free articles. Join our Telegram channel

Full access? Get Clinical Tree
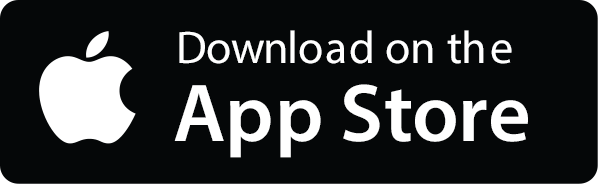
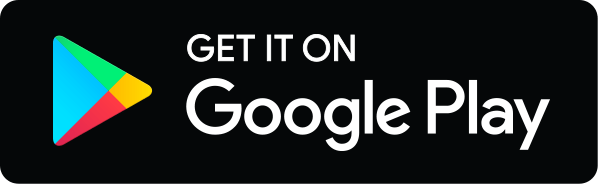