- Gene therapy for diabetes can be defined as transfer of DNA to somatic cells in order to understand, treat or prevent the disease or its complications.
- Because the etiology of diabetes is multifactorial in most cases, gene therapy aimed at correcting a single missing or multifunctioning gene will probably not be meaningful. Instead, gene therapy will need to interfere with more distal steps in the pathogenesis of diabetes, correct insulin deficiency or treat secondary complications.
- It is possible to outline several different gene transfer strategies for diabetes; prevention of β-cell destruction could be achieved by manipulating β-cells to produce a protection or survival factor.
- β-cell destruction might also be prevented by immunomodulation.
- Stimulation of β-cell differentiation and regeneration might involve gene therapy with transcription factors that control β-cell development.
- Ectopic production of insulin by substitute cells has been achieved with fibroblasts, hepatocytes, myocytes, pituitary and exocrine cells.
- Although experimental results are promising, none of the proposed gene therapy models for treatment or prevention of diabetes have reached the stage of clinical testing.
- Clinical trials are in progress with a view to treat diabetic complications such as foot ulcer and limb ischemia.
Gene transfer techniques for genetic modification of pancreatic islets
The ability to engineer pancreatic β-cells is a prerequisite for a successful application of most gene therapy approaches in diabetes. Because pancreatic islets are terminally differentiated cell clusters, gene transfer into islet cells poses significant technical hurdles. To date, several gene therapy vectors have demonstrated their utility in genetic modification of islet cells (Table 61.3). While viral vectors, such as adenovirus [1], lentivirus [2], retrovirus [3] and adeno-associated virus [4], show the most promising gene transfer efficiency into islet cells, it is likely that non-viral vector systems will more easily satisfy biosafety concerns in clinical trials.
Prevention of β-cell destruction in type 1 diabetes
β-Cell survival factors
Prevention of β-cell destruction could be achieved by genetic manipulation of β-cells so that they produce a β-cell protection and/or survival factor. Such an approach would, in general, leave the immune system unaffected as the transgene production is localized to the islets. Targeting of a survival factor to the β-cells could be applied to individuals in whom autoimmune destruction of β-cells has begun, but not reached the end stage. Candidate transgenes currently under investigation include those encoding antioxidant enzymes: glutathione peroxidase, mitochondrial manganese superoxide dismutase (MnSOD), catalase, cytosolic copper-zinc superoxide dismutase, anti -apoptotic proteins (members of the heat shock proteins and Bcl-2 families) and modulators of cytokine signaling pathways (modulation of nuclear factor κB, NFκB), and suppressors of cytokine signaling. Alternative approaches for cytoprotection of β-cells have been envisaged, such as immune modulators; for example, an interleukin-1 (IL-1) receptor antagonist, hepatocyte growth factor, transforming growth factor β (TGF-β), adenoviral E3 and calcitonin gene-related peptide, inhibitors of Fas ligand signaling, and antistress factors such as thioredoxin. These factors have been addressed experimentally and could possibly, when expressed by β-cells in patients with diabetes, promote β -cell survival.
Genetic modulation of the immune system
In individuals with a high risk of developing T1DM, as indicated by genetic and humoral markers, but who have not yet entered the phase of autoimmune β-cell destruction, it might be possible to prevent the progression of the disease by DNA vaccination. In mice, it has already been observed that DNA vaccination with a glutamic acid decarboxylase 65 (GAD65) gene construct generates a protective humoral immune response and significantly delays the onset of diabetes [5]. Other studies of DNA vaccination in non-obese diabetic (NOD) mice were performed with a preproinsulin/glutamic acid decarboxylase 65 (Ins-GAD) fusion construct as the target antigen to introduce a larger number of autoantigenic target epitopes. DNA co-vaccination with Ins-GAD and B7-1wa (a membrane-bound molecule that can engage cytotoxic T-lymphocyte associated antigen 4 [CTLA-4] and promote negative signaling) generated protective regulatory T-cells and ameliorated the disease [6]. Both insulin and GAD65 may be key autoantigens in T1DM, and if the DNA vaccination approach leads to tolerance, β-cell destruction might be avoided.
Table 61.3 Properties of gene therapy vectors with demonstrated utility in islet transduction.
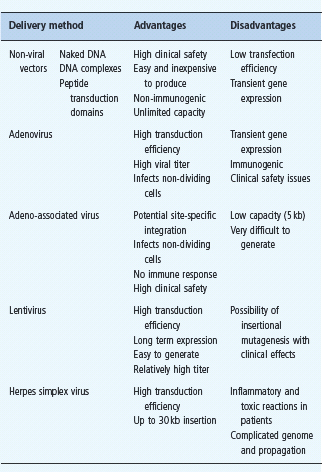
Furthermore, virus-based IL-10 genetic studies showed beneficial effects on the development of T1DM, both effectively blocking progression of insulitis as well as suppressing autoimmunity in NOD mice transplanted with syngeneic islet grafts [7]. Despite these results, significant immunosuppression, resulting from systemic expression of high levels of IL-10, give rise to serious concerns regarding the applicability of such an approach in clinical settings; however, the development of viral vectors promoting a tightly regulated transgene expression could overcome this obstacle.
Targeted immunomodulation has also been achieved by transducing autoantigen-specific CD4+ T-cells ex vivo with retroviruses that encode immune regulatory proteins [8]. Following intravenous injection of the transduced T-cells, it appears that the cells accumulate at the site of inflammation. Using this site-specific delivery, immune regulatory proteins have been observed to protect against autoimmune reactions, possibly by converting the immune reaction from a Th1 to a Th2 response.
An alternative approach may be targeting expression of disease-specific epitopes to activated B lymphocytes .9]. Antigen presentation by these cells seems to result in immunosupression and therapeutic efficacy. It is not clear why this is the case, but it has been suggested that antigen presentation by B lymphocytes leads to immune downregulation, whereas presentation by macrophages or dendritic cells is immune stimulatory.
Stimulation of β-cell differentiation and regeneration
A better understanding of the cellular sources for the expansion and turnover of β-cells seen in postnatal life could make way for a possible gene therapy approach leading to in situ regeneration of β-cells in patients with diabetes. Indirect evidence has suggested that postnatal β-cells derive from adult stem cells, proposed to reside in the pancreatic ducts, bone marrow, spleen or within islets. Lineage tracing experiments, however, demonstrated that the vast majority of adult β-cells derive from preexisting β-cells, suggesting that terminally differentiated β-cells retain a significant proliferative capacity and thus could represent an attractive target for expansion using gene therapy [10]. For example, mice over-expressing gastrin and TGF-α display a significantly increased islet cell mass. In addition, the cyclin-dependent kinase 4 (CDK4) and cyclins D1/D2 emerge as key determinants of β-cell proliferation and β-cell mass. Indeed, mice lacking CDK4 display a selective loss of β-cell function and over-expression of CDK4 results in preferential hyperplasia of the β-cells; however, it is likely that all approaches aiming at regenerating β-cells in patients with T1DM must be combined with a strategy to prevent autoimmune destruction of the newly formed β-cells.
Ectopic production of insulin by substitute cells
With the cloning of the insulin gene in the late 1970s, it was proposed that using a suitable promoter and insulin gene construct, non-insulin-producing cells could be made into insulin-producing substitute cells, and that such cells could restore insulin production in T1DM and some patients with T2DM. The original experiments were carried out on cell lines and fibroblasts, but since then hepatocytes, myocytes, pituitary and exocrine cells have also been made into insulin-producing substitute cells. Proinsulin is only converted into insulin by the prohormone convertases PC2 and PC3, which are not expressed in most substitute cells. This limitation has been overcome by mutating the insulin gene so that a furin cleavable site is generated (INS-FUR). The protease furin, unlike PC2 and PC3, is expressed in most cells. Although processing of proinsulin to insulin can be achieved in substitute cells, none of these cells would be able to respond to insulin secretagogues with a physiologic secretion of insulin. Recent studies showed that both lentivirus [11] and adeno-associated virus vectors [12] were able to deliver INS-FUR cDNA efficiently to hepatocytes in vitro and in vivo. resulting in long-term correction of diabetes in rats.
Given its role in β-cell maintenance, the transcription factor Pdx-1 was investigated for its ability to induce ectopic insulin production in non β-cells in vivo.Ferber and colleagues have used adenoviral-mediated Pdx-1 overexpression for ex vivo transduction of adult human hepatocytes for cell-based therapy. In addition to glucagons, insulin and somatostatin, exogenous expression of Pdx-1 induced transcription of several β-cell products, including glucose transporter 2 and glucokinase, endogenous Pdx-1 and multiple downstream pro-endocrine developmental factors [13].
In a recent study Zhou et al.describe a way to reprogram pancreatic exocrine cells into insulin producing β-cells [14]. By using adenoviruses, they introduced combinations of nine different genes, previously identified to be essential to the embryonic development of β-cells, into the pancreas of live mice. They found that the transfer of three transcription factors (Ngn3, Pdx1 and Mafa) induces trans-differentiation of up to 20% of the successfully manipulated exocrine cells into β-like cells. By lineage tracing experiments, the authors proved that the trans-differentiated cells were, indeed, exocrine cells. These reprogrammed β-cells had similar morphology, protein expression and the capacity to secrete insulin as their “natural” counterparts. Moreover, they were able to improve hyperglycemia in mice with induced diabetes. This study elegantly shows that adult cells can be directly converted into another type of adult cell, which opens the possibility of directly converting cells in vivo for repair and regeneration as a therapeutic tool for diabetes and beyond [14].
Cell therapy using β-cells derived from embryonic stem cells
This topic is discussed in detail in the first part of this chapter.
Ex vivo gene transfer to islets destined for transplantation
Pancreatic islet transplantation has been validated as a realistic alternative to correct the insulin deficiency in T1DM; however, islets are exposed to a plethora of insults before, during, after isolation and at the site of implantation, all of which could result in cellular death and impaired function, therefore reducing the yield of viable islets that engraft after implant. As a result, much effort has been made to understand better the molecular mechanisms involved in all these processes as well as in developing new therapeutic strategies to increase durable functional islet mass. In this context, ex vivogene transfer to pancreatic islets represents an attractive approach towards enhancing graft survival after transplantation. Most of the gene therapy strategies described above show promise for the cytoprotection of islets in transplant settings and may ultimately promote significantly enhanced function and survival of transplanted islets leading to an improved outcome of the transplantation procedures (Table 61.4); however, considering the complex pathways involved in β-cell destruction and loss of function following islet transplantation, it is likely that gene therapy strategies targeting multiple genes, using multicistronic vectors, will be more beneficial for the improvement of graft survival. For this purpose, a number of factors still remain to be investigated to find which combination of genes proves optimal, what threshold level of gene expression is required and what delivery strategy is the most efficient for targeting the mechanisms leading to graft failure.
Table 61.4 Examples of gene therapy-based strategies leading to improved outcome of islet transplantation in animal models.
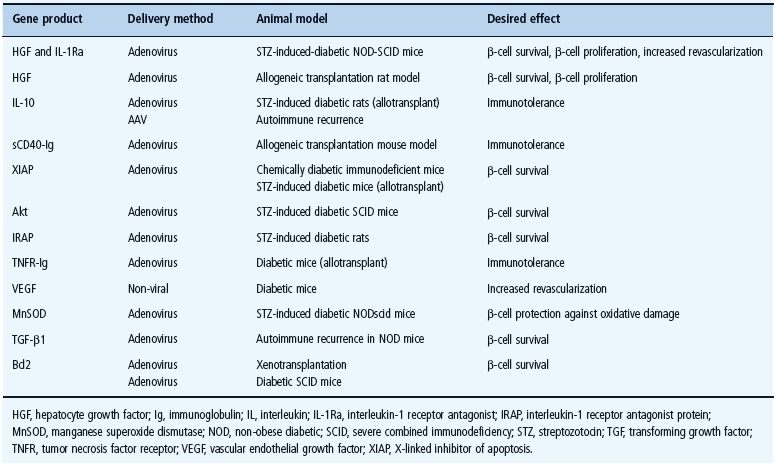
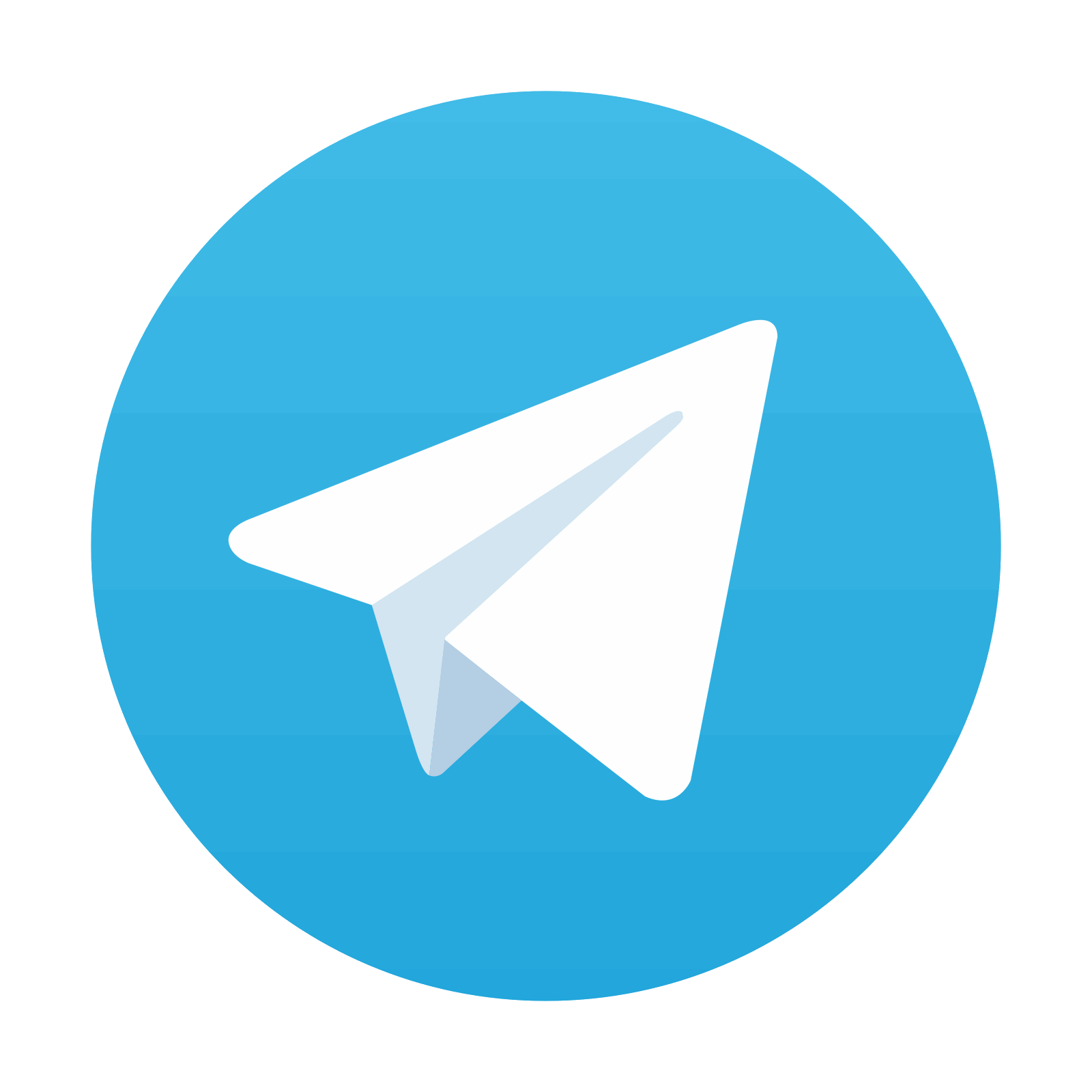
Stay updated, free articles. Join our Telegram channel

Full access? Get Clinical Tree
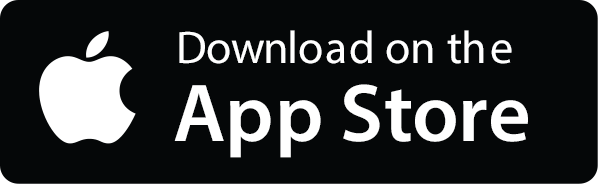
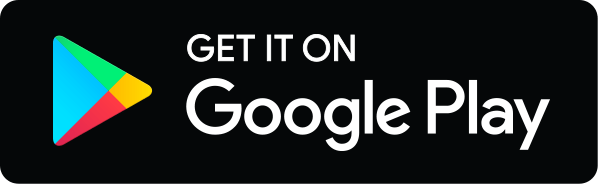