Objectives
- 1.
Explain the mechanisms of thyroid hormone synthesis.
- 2.
Describe the regulation of thyroid function and the actions of thyroid hormones.
- 3.
Compare and contrast the functions of thyroxine and triiodothyronine.
- 4.
Discuss the peripheral conversion of thyroid hormones by deiodinases.
- 5.
Draw the regulatory feedback loop for the regulation of thyroid function.
- 6.
Understand the etiology, major symptoms, and pathophysiology of the symptoms for Graves disease, Hashimoto thyroiditis, sporadic congenital hypothyroidism, and cretinism.
The thyroid gland produces the prohormone, tetraiodothyronine (T 4 ), and the active hormone, triiodothyronine (T 3 ). The synthesis of T 4 and T 3 requires iodine, which can be a limiting factor in some parts of the world. Much of T 3 is also made by peripheral conversion of T 4 to T 3 . T 3 acts primarily through a nuclear receptor that regulates gene expression. T 3 is critical for normal brain and skeletal development, and has broad effects on metabolism and cardiovascular function in the adult.
Anatomy and Histology of the Thyroid Gland
The thyroid gland is composed of right and left lobes that sit anterolaterally to the trachea ( Fig. 6.1 ). Normally, the lobes of the thyroid gland are connected by a midventral isthmus. The functional unit of the thyroid gland is the thyroid follicle , a spherical structure about 200 to 300 μm in diameter ringed by a single layer of thyroid epithelial cells ( Fig. 6.2 ). The epithelium sits on a basal lamina, surrounded by a rich capillary supply. The apical side of the follicular epithelium faces the lumen of the follicle. The lumen is filled with colloid composed of thyroglobulin , which is secreted and iodinated by the thyroid epithelial cells. The size of the epithelial cells and the amount of colloid are dynamic features that change with the activity of the gland. The thyroid gland contains another type of cell in addition to follicular cells. Scattered within the gland are parafollicular cells , or C cells . These cells are the source of the polypeptide hormone calcitonin , whose function is unclear in humans.
Although parafollicular C cells and calcitonin may be of minimal importance in normal humans, C cells can give rise to medullary thyroid carcinoma . Medullary cancer is an aggressive form of thyroid cancer, and metastasis to lungs, liver, bone, or other organs drastically reduces survival. Although most medullary cancer is sporadic, about one fifth of cases are familial. The familial forms are due to activating mutations of the RET protooncogene , a tyrosine kinase receptor that interacts with coreceptors and is activated by glial-derived neurotrophic factor and related proteins. Familial medullary thyroid cancer can occur independently of other endocrine glands or as part of a multiple endocrine neoplasia (MEN) syndrome, which in this case also involves adrenal medulla chromaffin cells (pheochromocytoma), parathyroid glands, and/or ganglioneuromas. These cancers remain differentiated enough to secrete calcitonin , and assay of calcitonin in the blood is useful in assessing treatment and possible recurrence during follow-up. Medullary thyroid cancer is treated by total thyroidectomy and removal of regional lymph nodes. Effective chemotherapy regimens that target the tyrosine kinase receptor, c-ret, have also been developed.
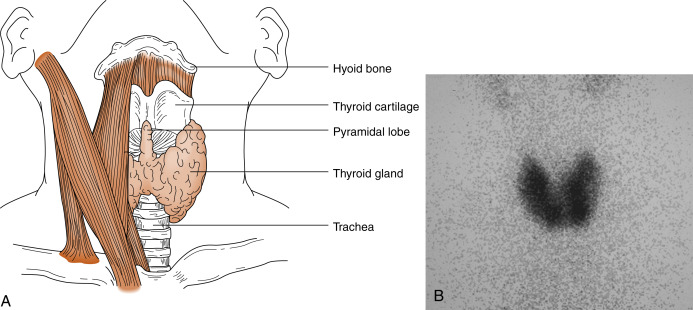
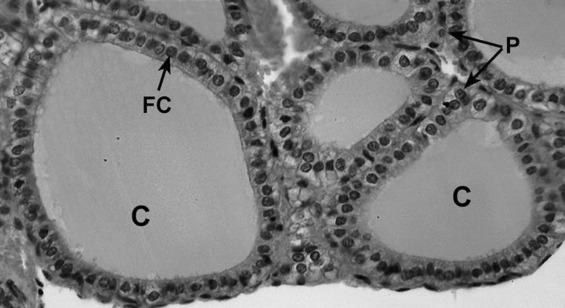
Production of Thyroid Hormones
The secretory products of the thyroid gland are iodothyronines ( Fig. 6.3 ), a class of hormones resulting from the coupling of two iodinated tyrosine molecules. About 90% of the thyroid output is 3,5,3′,5′-tetraiodothyronine (thyroxine , or T 4 ). T 4 is a prohormone. About 10% is 3,5,3′-triiodothyronine (T 3 ), which is the active form of thyroid hormone. Less than 1% of thyroid output is 3,3′,5′-triiodothyronine (reverse T 3 , or rT 3 ), which is inactive. Normally, these three products are secreted in the same proportions at which they are stored in the gland.
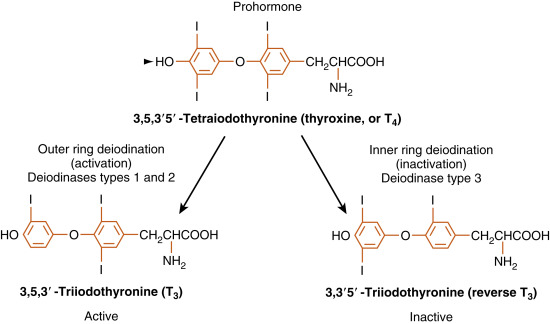
Because the primary product of the thyroid gland is T 4 , yet the active form of thyroid hormone is T 3 , the thyroid axis relies heavily on peripheral conversion through the action of thyronine-specific deiodinases (see Fig. 6.3 ).
Type 1 deiodinase , which is expressed in the plasma membrane of liver and kidney cells, is a low-affinity enzyme capable of both outer- and inner-ring deiodination of T 4 . Current evidence suggests that type 1 deiodinase may function primarily as a scavenger enzyme that removes iodine from sulfated thyroid hormones before they are excreted in bile or urine. However, in hyperthyroidism, type 1 deiodinase is a major contributor to elevated circulating T 3 levels in this disease.
Type 2 deiodinase , on the other hand, is a high-affinity ( Km = 1 nM) outer-ring deiodinase that converts T 4 to T 3 . It is localized intracellularly in the endoplasmic reticulum and expressed in several cell types, including glial cells of the central nervous system (CNS), the pituitary gland, brown fat, placenta, and skeletal muscle (albeit at low levels). Cell types that express the type 2 deiodinase are able to customize the levels of available T 3 in their local environment. For example, the brain can maintain constant intracellular levels of T 3 by upregulating type 2 deiodinase in hypothyroidism, when free T 4 falls to low levels. Importantly, type 2 deiodinase is also present in the thyrotropes of the pituitary, where it acts as a “thyroid axis sensor” by integrating total circulating free T 3 and T 4 . T 3 in the thyrotrope, which either enters the cell as T 3 or is converted from T 4 by the type 2 deiodinase, represents the feedback signal that regulates thyroid-stimulating hormone (TSH) secretion (see later). Brown fat is able to increase heat production in response to adrenergic stimulation of local T 3 production by the type 2 deiodinase (discussed later). In addition to local production of T 3 , type 2 deiodinase generates most of the circulating pool of T 3 in humans under euthyroid conditions.
Finally, there also exists an “inactivating” deiodinase, called type 3 deiodinase . Type 3 deiodinase is a high-affinity, inner-ring deiodinase that converts T 4 to the inactive rT 3 . Type 3 deiodinase is increased during hyperthyroidism, which helps to blunt the overproduction of T 4 . All forms of iodothyronines are further deiodinated, eventually to noniodinated thyronine ( Table 6.1 ).
T 4 | T 3 | rT 3 | |
---|---|---|---|
Daily production (μg) | 90 | 35 | 35 |
From thyroid (%) | 100 | 25 | 5 |
From T 4 (%) | — | 75 | 95 |
Extracellular pool (μg) | 850 | 40 | 40 |
Plasma concentration | |||
Total (μg/dL) | 8.0 | 0.12 | 0.04 |
Free (ng/dL) | 2.0 | 0.28 | 0.20 |
Half-life (days) | 7 | 1 | 0.8 |
Metabolic clearance (L/day) | 1 | 26 | 77 |
Fractional turnover per day (%) | 10 | 75 | 90 |
Iodide Balance
Because of the unique role of iodide (iodide, or I − , is the water-soluble ionized form of diatomic iodine, or I 2 ) in thyroid physiology, a description of thyroid hormone synthesis requires some understanding of iodide turnover ( Fig. 6.4 ). The average dietary intake of iodide is 400 μg in the United States , compared with a minimum daily requirement of 150 μg for adults, 90 to 120 μg for children, and 200 μg for pregnant women. In the steady state, virtually the same amount, 400 μg, is excreted in the urine. Iodide is actively concentrated in the thyroid gland, salivary glands, gastric glands, lacrimal glands, mammary glands, and choroid plexus. About 70 to 80 μg of iodide is taken up daily by the thyroid gland. The total iodide content of the thyroid gland averages around 7500 μg, virtually all of which is in the form of iodothyronines. In the steady state, 70 to 80 μg of iodide, or about 1% of the total, is released from the gland daily. Of this amount, 75% is secreted as thyroid hormone, and the remainder is secreted as free iodide. The large ratio (100:1) of iodide stored in the form of hormone to the amount turned over daily protects against iodide deficiency for about 2 months. Iodide is also conserved by a marked reduction in the renal excretion of iodide as the concentration in serum falls.
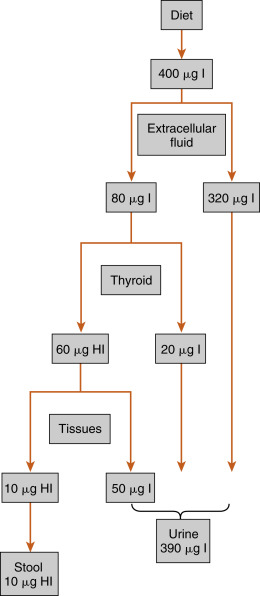
Overview of Thyroid Hormone Synthesis
To understand thyroid hormone synthesis and secretion, one must appreciate the directionality of each process as it relates to the polarized thyroid epithelial cell ( Fig. 6.5 ). Synthesis of thyroid hormone requires two precursors, thyroglobulin and iodide . Iodide is transported across cells from the basal (vascular) side to the apical (follicular luminal) side of the thyroid epithelium. Amino acids are assembled by translation into thyroglobulin, which is then secreted from the apical membrane into the follicular lumen. Thus synthesis involves a basal-to-apical movement of precursors into the follicular lumen (see Fig. 6.5 ; black arrows ). Actual synthesis of iodothyronines occurs enzymatically in the follicular lumen close to the apical membrane of the epithelial cells (see later). Secretion involves fluid phase endocytosis of iodinated thyroglobulin and apical-to-basal movement of the endocytic vesicles and their fusion with lysosomes. Thyroglobulin is then enzymatically degraded, which results in the release of thyroid hormones from the thyroglobulin peptide backbone. Finally, thyroid hormones move across the basolateral membrane, probably through a specific transporter, and ultimately into the blood. Thus secretion involves an apical-to-basal movement (see Fig. 6.5 ; orange arrows ). There are also scavenger pathways within the epithelial cell that reuse iodine and amino acids after enzymatic digestion of thyroglobulin (see Fig. 6.5 ; open arrows ).
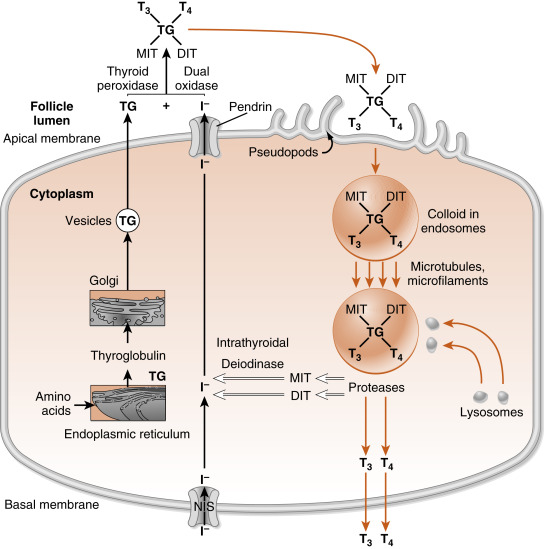
Synthesis of Iodothyronines Within a Thyroglobulin Backbone
Iodide is transported into the gland against chemical and electrical gradients by a Na + -I – symporter (NIS) located in the basolateral membrane of thyroid epithelial cells. Normally, a thyroid-to-plasma free iodide ratio of 30 is maintained. This so-called iodide trap is highly expressed in the thyroid gland, but NIS is also expressed at lower levels in the placenta, salivary glands, and actively lactating breast. One iodide ion is transported uphill against an iodide gradient, whereas two sodium ions move down the electrochemical gradient from the extracellular fluid into the thyroid cell. The energy source for this secondary active transporter is provided by a Na + , K + -ATPase in the plasma membrane. Expression of the NIS gene is inhibited by iodide and stimulated by TSH (see later in the text). Numerous inflammatory cytokines also suppress NIS gene expression. A reduction in dietary iodide intake depletes the circulating iodide pool and greatly enhances the activity of the iodide trap. When dietary iodide intake is low, the rates of thyroid uptake of iodide can reach 80% to 90%.
The steps in thyroid hormone synthesis are shown in Fig. 6.6 . After entering the gland, iodide rapidly moves to the apical plasma membrane of the epithelial cells. From there, iodide is transported into the lumen of the follicles by a sodium-independent iodide-chloride transporter, named pendrin .
Pendred syndrome refers to a condition caused by an autosomal recessive mutation in the pendrin gene (referred to as PDS or SLC26A4 ). Because iodide is not efficiently transported into the follicular lumen, hypothyroidism develops in patients. Some patients exhibit enlarged thyroid glands called goiters . This form of hypothyroidism can be treated with replacement thyroxine. Unfortunately, pendrin is also expressed in the inner ear and is required for normal structural development of the inner ear. Thus patients with Pendred syndrome experience hearing loss in infancy or early childhood.
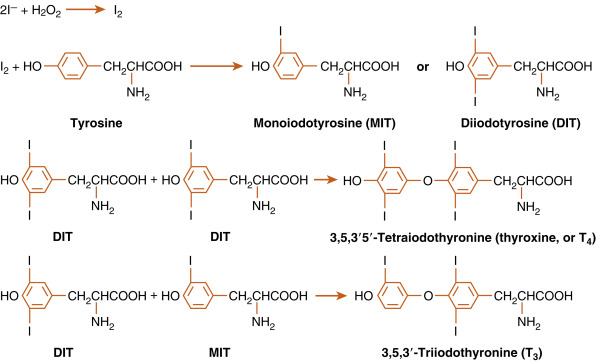
Once in the follicular lumen, iodide (I – ) is immediately oxidized and incorporated into tyrosine residues within the primary structure of thyroglobulin. Thyroglobulin is continually synthesized, exocytosed into the follicular lumen and iodinated to form either monoiodotyrosine (MIT) or diiodotyrosine (DIT) (see Fig. 6.6 ). After iodination, two DIT molecules are coupled to form T 4 , or one MIT and one DIT molecule are coupled to form T 3 . The coupling occurs between iodinated tyrosines that remain part of the primary structure of thyroglobulin. This entire sequence of reactions is catalyzed by thyroid peroxidase (TPO), an enzyme complex that spans the apical membrane. The immediate oxidant (electron acceptor) for the reaction is hydrogen peroxide (H 2 O 2 ). H 2 O 2 is generated in the thyroid gland by an enzyme called dual oxidase (Duox) that is also localized to the apical membrane.
When iodide availability is restricted, the formation of T 3 is favored. This response provides more active hormone per molecule of organified iodide. The proportion of T 3 is also increased when the gland is hyperstimulated by TSH or other activators.
Secretion of Thyroid Hormones
After thyroglobulin has been iodinated, it is stored in the lumen of the follicle as colloid (see Fig. 6.2 ). Release of the T 4 and T 3 into the bloodstream requires endocytosis and lysosomal degradation of thyroglobulin (see Fig. 6.5 ; orange arrows ). Enzymatically released T 4 and T 3 then leave the basal side of the cell and enter the blood.
The MIT and DIT molecules, which also are released during proteolysis of thyroglobulin, are rapidly deiodinated within the follicular cell by an enzyme called intrathyroidal deiodinase (see Fig. 6.5 ; open arrows ). This deiodinase is specific for MIT and DIT and cannot use T 4 and T 3 as substrates. The iodide is then recycled into T 4 and T 3 synthesis. Amino acids from the digestion of thyroglobulin reenter the intrathyroidal amino acid pool and can be reused for protein synthesis. Only minor amounts of intact thyroglobulin leave the follicular cell under normal circumstances.
Because of its ability to trap and incorporate iodine into thyroglobulin (called organification ), the activity of the thyroid can be assessed by radioactive iodine uptake (RAIU) . For this, a tracer dose of 123 I is administered, and the RAIU is measured by placing a gamma detector on the neck after 4 to 6 hours and after 24 hours. In the United States, where the diet is relatively rich in iodine, the RAIU is about 15% after 6 hours and 25% after 24 hours ( Fig. 6.7 ). Abnormally high RAIU (> 60%) after 24 hours indicates hyperthyroidism. Abnormally low RAIU (< 5%) after 24 hours indicates hypothyroidism. In individuals with extreme chronic stimulation of the thyroid (Graves disease–associated thyrotoxicosis), iodide is trapped, organified, and released as hormone very rapidly. In these cases of elevated turnover, the 6-hour RAIU will be very high, but the 24-hour RAIU will be lower ( Fig. 6.8 ). A number of anions, such as thiocyanate (SCN − ), perchlorate ( <SPAN role=presentation tabIndex=0 id=MathJax-Element-1-Frame class=MathJax style="POSITION: relative" data-mathml='HCIO4−’>HCIO−4HCIO4−
HCIO 4 −
), and pertechnetate ( <SPAN role=presentation tabIndex=0 id=MathJax-Element-2-Frame class=MathJax style="POSITION: relative" data-mathml='TcO−4′>TcO4−TcO−4
TcO − 4
), are inhibitors of iodide transport through the NIS. If iodide cannot be rapidly incorporated into tyrosine ( organification defect ) after its uptake by the cell, administration of one of these anions will, by blocking further iodide uptake, cause a rapid release of the iodide from the gland (see Fig. 6.8 ). This release occurs as a result of the high thyroid-to-plasma concentration gradient of iodide.
