and Francesco J. DeMayo2
(1)
Translational Biology and Molecular Medicine Graduate Program, Baylor College of Medicine, Houston, TX 77030, USA
(2)
Department of Molecular and Cellular Biology, Baylor College of Medicine, Houston, TX 77030, USA
Abstract
The ovarian hormones, estrogen and progesterone, and their receptors, the estrogen receptor (ER) and progesterone receptor (PR), orchestrate the complex sequence of events required for uterine receptivity and the establishment of pregnancy. The actions of ER, PR, and other steroid hormone receptors (SHRs) direct the uterus through the processes of implantation and decidualization. Due to the ethical concerns of studying pregnancy in humans, genetically engineered rodent models have facilitated many of the discoveries that have elucidated the molecular events directing early pregnancy. This chapter will cover the conserved structure and function of the SHRs. ER and PR will be highlighted for their pivotal roles in uterine receptivity, implantation, and decidualization. The dynamic regulation of ER and PR expression and activity throughout the estrous cycle and early pregnancy, and the importance of SHRs in coordinating paracrine signaling between the endometrial compartments will also be explored. Finally, the roles of androgen receptor (AR) and glucocorticoid receptor (GR) in the establishment of pregnancy will be discussed.
3.1 Introduction
Pregnancy is a complex process, requiring the intricate coordination of implantation, decidualization, placentation, and parturition. Following fertilization, blastocysts are transported to the uterus via the oviducts. If the epithelium of the uterine endometrium is receptive, competent blastocysts are able to implant and invade into the surrounding endometrium. In the mouse, embryo implantation induces the endometrial stromal cells underlying the implantation site to undergo the process of decidualization, or differentiation into decidual cells; in humans, this process occurs independently of embryo implantation (reviewed in Cha et al. 2012). Decidual cells serve many functions during early pregnancy, as they support the developing embryo, regulate trophoblast invasion, enhance vascularization, and modulate maternal immunity. Successful decidualization sets the stage for placentation, in which a robust placental vascular network develops to support the developing embryo until parturition. Implantation, decidualization, and placentation are tightly regulated, and defects in any of these early processes can result in adverse pregnancy outcomes or infertility (Cha et al. 2012). The complex coordination of these sequential, interrelated processes is achieved through the activities of steroid hormone receptors (SHRs), notably estrogen receptor (ER) and progesterone receptor (PR). This chapter will detail the conserved structure and function of the SHRs, and the role of these crucial signaling molecules in the establishment of pregnancy in rodents. ER and PR, which mediate the activities of the ovarian hormones, estrogen and progesterone, will be highlighted for their critical roles in uterine receptivity and the establishment of pregnancy. Additionally, the roles of the androgen receptor (AR) and glucocorticoid receptor (GR) in the establishment of pregnancy will be discussed. Throughout the chapter, the activities of the SHRs will be illustrated by findings obtained from genetically engineered mouse models, which have facilitated essential insights into the biology of reproduction.
3.2 The Structure and Function of Steroid Hormone Receptors
Nuclear receptors (NRs) are crucial effectors of signaling networks, directing changes in gene transcription in response to changing conditions. The NR superfamily includes 48 proteins that regulate gene transcription to direct critical cellular processes, including survival, proliferation, and differentiation (reviewed in Tata 2002). Many of the NRs are activated by the binding of ligand molecules, while others are considered “orphan” receptors with no identified ligand regulating their transcriptional activity. The estrogen receptor-like subfamily of NRs (NR3) houses the steroid hormone-binding receptors, including ER, PR, AR, and GR. These SHRs function as transcription factors, effecting changes in gene transcription in response to extracellular signals that are communicated via hormone ligands.
Steroid hormones are secreted by endocrine organs to coordinate complex developmental and physiological processes. These molecules circulate through the blood to reach their target organs, where the lipophilic nature of their steroid rings allows them to cross the cell membrane via simple diffusion. Because diffusion is a nonspecific process, the cellular response to these hormones is directed by the specific expression of SHRs. In the absence of ligand, SHRs reside in the cytoplasm, bound to a complex of chaperone proteins (Smith 1993). These chaperones hold the receptor in an inactive state, primed to bind ligand (Picard et al. 1990). Upon ligand binding, SHRs undergo a conformational change that triggers release from the chaperone complex and favors receptor dimerization. Dimerized hormone-receptor complexes translocate to the nucleus, where they bind to DNA and direct the recruitment of transcriptional coactivators, corepressors, and the transcriptional machinery to modulate the expression of target genes (reviewed in Shibata et al. 1997).
The SHRs share a common structure, composed of an amino-terminal DNA-binding domain (DBD), a carboxy-terminal ligand-binding domain (LBD), and a flexible hinge region that bridges these modular domains (Fig. 3.1) (reviewed in Beato and Klug 2000). The LBD is highly conserved among SHRs, forming a pocket for hormone binding near the receptor’s carboxy-terminus (Wurtz et al. 1996). The LBD is also responsible for SHR interactions with the chaperone protein, heat shock protein 90 (HSP90) (Ricketson et al. 2007). Upon ligand binding, a conformational change is induced, facilitating release of the SHR from the large chaperone complex. The ligand-bound conformation also favors SHR dimerization and DNA binding (Kumar and Chambon 1988). Additionally, the LBD contains a nuclear localization signal (NLS) that targets SHRs to the nucleus (Guiochon-Mantel et al. 1989). This NLS is ligand-dependent, enhancing the translocation of ligand-bound SHRs to the nucleus.
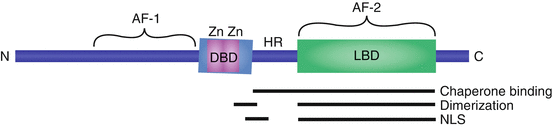
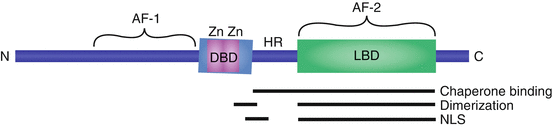
Fig. 3.1
The conserved structure of SHRs. Receptor isoforms vary by the length of their amino-terminus. The DNA-binding domain (DBD) is highly conserved between SHRs and encodes two zinc fingers (Zn). The ligand-binding domain (LBD) forms a pocket for the binding of steroid hormone ligands. The hinge region (HR) bridges the DBD and LBD. The constitutive transactivation domain (AF-1) is encoded by the amino-terminal amino acids, while the ligand-dependent transactivation domain (AF-2) is contained within the LBD. The regions responsible for binding chaperone proteins, receptor dimerization, and nuclear localization (NLS) are shown
The flexible hinge region bridges the DBD and LBD and serves several functions, as it can affect DNA-binding and dimerization activities (Daniel et al. 2010). The flexible hinge region contains an additional, constitutively active NLS (Guiochon-Mantel et al. 1989). Further, this region houses protein-protein interaction domains that, along with the LBD, are responsible for SHR interactions with chaperone proteins.
The DBD is highly conserved among SHRs, encoding two zinc fingers that are responsible for sequence recognition and DNA binding (Green et al. 1988). The DBD targets dimerized SHRs to a specific nucleotide sequence, called a hormone response element (HRE). The nucleotide sequence of an HRE is palindromic; each SHR monomer recognizes one-half site of the HRE (Luisi et al. 1991). HREs are enriched in the promoter regions of target genes, allowing SHRs to recruit the transcriptional machinery and coregulator proteins to alter transcriptional activity. Although each SHR has its own unique HRE, the SHRs are often able to bind to the response elements of other receptors.
SHRs regulate transcriptional activity through two transactivation domains, which directly interact with transcription factors and coactivators to regulate the initiation of transcription at target loci. The first transactivation domain, referred to as activation function 1 (AF-1), is located at the amino-terminus, near the DBD (reviewed in Lavery and McEwan 2005). AF-1 is constitutively activating, promoting the initiation of transcription in a ligand-independent fashion. An important exception is the AF-1 domain of human PR, which encodes an inhibitory domain, resulting in constitutive inhibition of transcriptional activity (Giangrande et al. 1997). The second transactivation domain (AF-2) is ligand inducible, directing enhanced transcriptional activation in response to ligand binding.
In addition to sharing a conserved structure, SHR activity can be regulated by common mechanisms. First, the activity of SHRs is modulated by subcellular localization. SHRs must translocate to the nucleus in order to alter transcription, a process that is directed by the NLSs encoded by each SHR. SHRs are also regulated through differential promoter usage and alternative splicing, resulting in the production of distinct receptor isoforms (Hollenberg et al. 1985; Conneely et al. 1989; Kastner et al. 1990; Encio and Detera-Wadleigh 1991; Mosselman et al. 1996; Wilson and McPhaul 1996). Further regulation of SHR activity can be achieved through posttranslational modifications. Notably, all of the SHRs are phosphoproteins, subject to phosphorylation and dephosphorylation events that modulate their activity (reviewed in Weigel and Moore 2007). Finally, SHR-driven changes in transcription are influenced by the action of transcriptional coregulators. Notably, steroid receptor coactivator (SRC) family proteins are critical coactivators of ER, PR, and GR, interacting with the AF-2 domains of these SHRs in a ligand-dependent fashion (reviewed in Lonard and O’Malley 2012).
3.3 The Establishment of Pregnancy in Rodents
The establishment of pregnancy is an intricate process requiring the coordination of multiple, interdependent steps. The processes of ovulation, fertilization, implantation, decidualization, placentation, and parturition must be coordinated across both time and space. The ovarian hormones, estrogen and progesterone, and their cognate SHRs, ER and PR, are responsible for the orchestration of these events and are absolutely essential for the successful establishment of pregnancy (reviewed in Vasquez and DeMayo 2013). Due to their pivotal importance in directing female reproduction, expression of ER and PR in the uterus is highly specific and dynamic, subject to compartment-specific regulation that changes rapidly during early pregnancy (Tibbetts et al. 1998; Tan et al. 1999).
The uterus is composed of two main compartments: the myometrium and the endometrium. The myometrium is located externally, consisting of an outer, longitudinal muscle layer and an inner, circular muscle layer. The endometrium forms the inner layer of the uterus and is further subdivided into an epithelial compartment, composed of luminal and glandular epithelia, and a subepithelial stromal compartment. The luminal epithelium is the site of blastocyst attachment and implantation. In mice, attachment occurs at the antimesometrial surface of the uterine lumen. The secretory products of the endometrial glands are critical for successful attachment and implantation (Jeong et al. 2010). In rodents, blastocyst attachment triggers the stromal cells underlying the implantation site to differentiate into specialized decidual cells in a process termed decidualization. Decidual cells support the developing embryo, regulate trophoblast invasion, and modulate maternal immunity. Importantly, implantation and decidualization set the stage for healthy pregnancy, and defects in these processes can result in negative outcomes at later time points (reviewed in Cha et al. 2012).
In the mouse, female reproductive function is regulated by the estrous cycle, which lasts 4–5 days. The murine estrous cycle consists of four phases: proestrous, estrous, metestrous, and diestrous. The proestrous phase is marked by estrogen production and the accumulation of mature follicles in the ovaries. Ovulation occurs in the estrous phase, during which females are most receptive to mating. Following fertilization, day 1 of pregnancy (the time of vaginal plug) is marked by proliferation of the endometrial epithelium under the influence of preovulatory estrogen. This parallels the proliferative phase of the menstrual cycle. During days 2 and 3, corresponding to the early secretory phase of the menstrual cycle, the endometrium prepares for implantation under the influence of progesterone, which is produced by the corpus luteum. In the mouse, the maintenance of the corpus luteum and progesterone secretion requires cervical stimulation from mating. ER-driven epithelial proliferation ceases due to declining levels of estrogen and the antagonistic action of progesterone. Expression of PR in the endometrial epithelium spikes, resulting in downregulation of ER target genes. By day 4, however, epithelial PR levels drop, resulting in loss of PR signaling in this compartment despite high circulating levels of progesterone. At the same time, a preimplantation surge in estrogen induces the expression of genes that are critical to uterine receptivity, including leukemia inhibitory factor (LIF) (Finn and Martin 1974; McCormack and Greenwald 1974; Hewitt et al. 2012). This marks the beginning of the window of receptivity. The murine uterus is receptive to blastocyst implantation on day 4 of pregnancy. Blastocyst implantation triggers the differentiation of stromal cells, and decidualization spreads throughout the stroma surrounding the implantation chamber. In the absence of blastocyst implantation, the uterus becomes nonreceptive by day 5. If pregnancy is not achieved, the mouse enters the diestrous phase, characterized by involution of the corpus luteum and resorption of the endometrium.
3.4 Estrogen Receptor (ER)
ER (Esr) exists as two distinct isoforms, ERα (Esr1) and ERβ (Esr2), which are transcribed from distinct genes (Mosselman et al. 1996). These isoforms can form homo- and heterodimers to regulate transcriptional activity (Pettersson et al. 1997). ERα, the first ER isoform to be discovered, is well characterized. Isoform-specific ablation of ER in mice has shown that ERα regulates uterine function during reproduction. Esr1 knockout (αERKO) mice are infertile due to defects in ovarian function and signaling along the hypothalamic-pituitary axis (Couse and Korach 1999). In contrast, Esr2 knockout (βERKO) mice exhibit a mild subfertile phenotype, producing fewer and smaller litters than wild-type mice (Krege et al. 1998; Dupont et al. 2000). This subfertile phenotype is due to loss of the luteinizing hormone (LH) surge, resulting from loss of Esr2 expression in the ovary (Jayes et al. 2014).
The ERα cistrome in the murine uterus has been characterized by chromatin immunoprecipitation followed by deep sequencing (ChIP-seq) (Hewitt et al. 2012). ERα was found to bind 5,814 sites basally, in vehicle-treated control uteri, and 17,240 sites following 1-hour estrogen treatment. Overlaying the ERα cistrome with gene expression data revealed that ERα binding is enriched near the transcription start site (TSS) (<10 kb) of genes that are positively regulated by estrogen. In contrast, genes that are downregulated by estrogen treatment show binding of ERα more distally.
ERα expression is tightly regulated in the reproductive organs of both humans and mice. ERα is expressed in all compartments of the uterus, the androgen-producing theca cells of the ovary, and the mammary glands. ERα is also expressed in the hypothalamic and pituitary glands. In the endometrium, ERα expression varies during the estrous cycle and early pregnancy (Tibbetts et al. 1998; Tan et al. 1999). ERα is strongly expressed in the glandular epithelium of the murine uterus and moderately expressed throughout the luminal epithelium and stroma. On day 1 of pregnancy, epithelial ERα expression is critical to mediate estrogen-dependent proliferation. ERα can still be detected in the epithelium and stroma at day 3 of pregnancy, immediately prior to the window of receptivity. By day 4, however, ERα expression has been lost from the luminal epithelium and stroma and is mostly limited to the mesometrial pole of the implantation chamber. This expression pattern reflects the loss of ERα in the decidualizing stromal cells that surround the implantation chamber. Importantly, strong ERα expression is maintained in the glandular epithelium of the uterus throughout days 3 and 4. During this period, the induction of ER target genes in the uterine glands is critical to prepare the uterus for embryo implantation. The expression of ERα is also tightly regulated during the human menstrual cycle. ERα expression is highest in the endometrial stroma and glands during the proliferative phase of the menstrual cycle. ERα levels decrease during the secretory phase of the menstrual cycle, as progesterone signaling becomes predominant.
3.4.1 ER Signaling Drives Proliferation of the Endometrial Epithelium
Estrogen regulates proliferation in a biphasic manner, directing both early and late proliferative events in the endometrium. Estrogen signaling stimulates a number of changes in the proliferating endometrium, including the transcription of cell cycle genes, DNA synthesis, epithelial mitosis, hyperemia, stromal edema, and the infiltration of immune cells. Estrogen-driven proliferation is mediated by paracrine signaling involving fibroblast growth factor (FGF) ligands. ER signaling induces FGF expression in stromal cells (Fujimoto et al. 1997; Tsai et al. 2002). Stromal FGF ligands activate epithelial FGF receptors (FGFRs), which drive the proliferative response by activating the downstream extracellular signal-regulated kinase (ERK1/2) or phosphoinositide 3-kinase (PI3K) pathways. Estrogen-dependent proliferation is also driven by insulin-like growth factor 1 (IGF1) (Zhu and Pollard 2007). IGF1, an ER target in the endometrium, is required for reproduction; deletion of Igf1 results in infertility in both male and female mice (Baker et al. 1996). The proliferative effect of estrogen is conserved in humans, as endometrial proliferation is dramatically enhanced as estrogen increases during the proliferative phase of the menstrual cycle (Ferenczy et al. 1979) (Fig. 3.2).
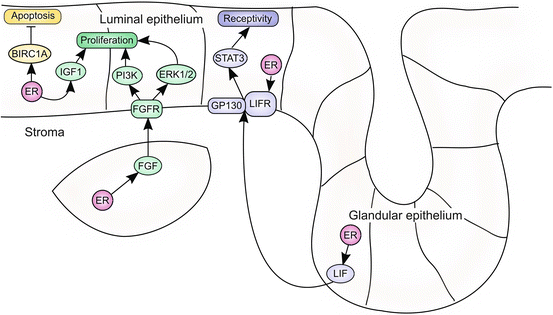
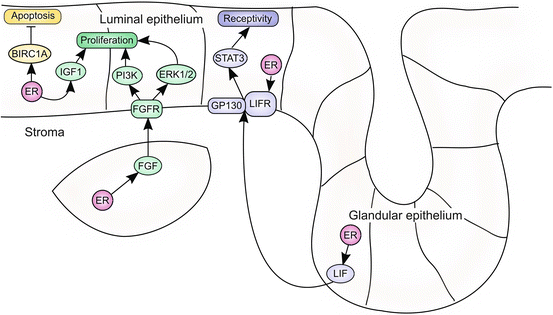
Fig. 3.2
ER signaling regulates endometrial proliferation and receptivity. Stromal ER drives proliferation of the endometrial epithelium via a paracrine signaling network. ER induces FGF expression in stromal cells, which act on FGFR expressed on the surface of epithelial cells. FGFR activates downstream signaling via PI3K and ERK1/2 to drive proliferation of epithelial cells. ER also induces IGF1 to further enhance proliferation. In addition, epithelial ER induces the expression of antiapoptotic targets, including BIRC1A. Uterine receptivity is mediated by ER-induced LIF signaling. ER induces expression of both LIF and its receptor, LIFR. LIF produced by the glandular epithelium signals to LIFR and the co-receptor GP130, which are expressed in the luminal epithelium. LIFR/GP130 activation results in activation of STAT3 and transition of the luminal epithelium to the receptive state
ERα-driven proliferation is a notable example demonstrating the importance of compartmental crosstalk in the endometrium. The proliferative response to estrogen is one of many endometrial functions that are coordinated by paracrine signaling between the epithelium and stroma. A reasonable hypothesis would be that estrogen stimulates ERα expressed in epithelial cells to drive proliferation in this same compartment. However, the use of mouse models has demonstrated that it is stromal ERα which is necessary and sufficient to drive proliferation of the endometrial epithelium. Indeed, in neonatal mice, the endometrial epithelium proliferates in response to circulating estrogen, despite the absence of ERα expression in this compartment (Bigsby and Cunha 1986). Instead, ERα is expressed in the stromal compartment and activates epithelial proliferation in a paracrine manner (Bigsby and Cunha 1986). Similar results have been obtained from tissue-grafting experiments, in which wild-type and αERKO endometrial tissues have been surgically recombined within the kidney capsule of mice (Cooke et al. 1997). Notably, estrogen treatment cannot stimulate proliferation in reconstructions of wild-type, ERα-expressing epithelium with αERKO stroma. However, grafts of αERKO epithelium with wild-type stroma exhibit a normal proliferative response to estrogen treatment, demonstrating that stromal ERα is necessary and sufficient to induce estrogen-driven proliferation of the epithelial compartment. Compartment-specific deletion of ERα from the endometrial epithelium using Wnt7a Cre (UTEpiαERKO) further corroborates the role of epithelial-stromal crosstalk in estrogen-driven proliferation (Winuthayanon et al. 2010). UTEpiαERKO mice are infertile due to implantation failure and exhibit dysfunctional expression of estrogen target genes in the endometrial epithelium. Interestingly, the epithelium retains the ability to proliferate in response to estrogen, despite the absence of ERα expression in the epithelial compartment. Following estrogen treatment, epithelial cells exhibit DNA synthesis and mitogen expression, suggesting that these responses are directed by ERα in the endometrial stroma. While estrogen-dependent proliferation occurs normally in UTEpiαERKO epithelium, these mice display increased epithelial apoptosis. The induction of apoptosis in UTEpiαERKO epithelium is likely due to loss of expression of antiapoptotic ER targets, such as BIRC1A (Yin et al. 2008). This increase in epithelial apoptosis, along with the dysregulation of ER target genes that mediate uterine receptivity, may contribute to the infertile phenotype seen in these mice.
3.4.2 The ER Target LIF Mediates Uterine Receptivity
In mice, a preimplantation estrogen surge is required to prepare the uterus for receptivity (Finn and Martin 1974; McCormack and Greenwald 1974). This estrogen surge occurs 24–48 h prior to implantation and results in the induction of ER target genes that prime the uterus for blastocyst implantation. LIF, a secreted cytokine in the interleukin-6 family, is one of the critical mediators of uterine receptivity. LIF is produced in the uterine glands in response to the preimplantation estrogen surge, and functions as a ligand for the LIF receptor (LIFR), which is expressed in the luminal epithelium (Aghajanova 2004). Binding of LIF to LIFR and the co-receptor, glycoprotein 130 (GP130), results in activation of the signal transducer and activator of transcription 3 (STAT3) signaling pathway in target cells (Ernst et al. 2001). Both Lif and Lifr are direct targets of ERα (Hewitt et al. 2012). LIF production is first evident in the glandular epithelium at day 3.5 of pregnancy and can later be detected in the endometrial stroma surrounding the site of blastocyst attachment (Stewart et al. 1992). Interestingly, LIFR and GP130 are also expressed on the blastocyst, suggesting a role for this ER signaling pathway in coordinating events between the mother and embryo. LIF is essential for uterine receptivity, as uterine deletion of Lif results in infertility due to implantation failure and defective decidualization (Chen et al. 2000). Notably, blastocyst implantation can be rescued by intraperitoneal injection of LIF (Chen et al. 2000). Implantation failure also occurs with deletion of the downstream mediators of the LIF pathway, GP130 (Il6st) or Stat3 (Lee et al. 2013; Pawar et al. 2013; Sun et al. 2013). In humans, a similar surge in circulating estrogen levels occurs during the mid-secretory phase of the menstrual cycle. LIF expression increases at the time of implantation, suggesting that this pathway may play a role in uterine receptivity in humans (Dey et al. 2004). Notably, endometrial samples from women with unexplained infertility and multiple implantation failures exhibit decreased expression of LIF during the mid-secretory phase compared to healthy controls (Wu et al. 2013). However, clear evidence that ER activity is required for receptivity in humans and primates remains elusive.
3.5 Progesterone Receptor (PR)
Progesterone, the hormone of pregnancy, is absolutely required for the establishment and maintenance of pregnancy (reviewed in Wetendorf and DeMayo 2014). In most species, including rodents, PR maintains the conserved structures and functionalities common to the SHRs. The DBD targets dimerized PR to the progesterone response element (PRE), which consists of the palindromic sequence, AGAACAnnnTGTTCT (Ham et al. 1988). Interestingly, PR is able to bind promoters and modulate the transcription of genes that contain only half of the PRE sequence or no PRE at all (Rubel et al. 2012). A crucial exception to the conserved SHR structure and function occurs in human PR. The AF-1 transactivation domain, which constitutively activates transcription in most SHRs, has inhibitory activity in human PR (Giangrande et al. 1997). This results in constitutive repression of transcription of PR target genes. The AF-2 domain of human PR retains ligand-dependent transactivating activity and activates transcription of PR target genes in response to hormone binding.
3.5.1 PR Exists as Multiple Isoforms with Distinct Functions
Similar to ER, which exists as the differentially regulated ERα and ERβ isoforms, PR occurs as two isoforms, PR-A and PR-B. Unlike ERα and ERβ, which are encoded by distinct genes, the PR isoforms arise from differential promoter usage at the Pgr gene (Conneely et al. 1989; Kastner et al. 1990). The longer isoform, PR-B, utilizes an upstream promoter. The amino-terminus of the PR-B isoform encodes an additional 164 amino acids, which form a third activation domain, termed AF-3 (Sartorius et al. 1994). This structural difference results in the strikingly different functions of the human PR-A and PR-B isoforms. Human PR-A suppresses transcription by recruiting the corepressor, nuclear corepressor 2 (NCOR2), to the loci of target genes (Giangrande et al. 2000). In contrast, PR-B functions primarily as a transcriptional activator (Vegeto et al. 1993). The AF-3 domain of PR-B is responsible for this dramatic functional difference. In the absence of an AF-3 domain, the activity of the human PR-A isoform is driven by the AF-1 domain, which is constitutively repressive. In the PR-B isoform, however, the inclusion of the AF-3 domain results in inhibition of the repressive activity of the AF-1 domain. Interestingly, the PR-B isoform is constitutively phosphorylated at Ser294 of the AF-1 domain. This phosphorylation is specific to the PR-B isoform and is thought to be responsible for the inhibition of the AF-1 domain’s repressive activity (Clemm et al. 2000). The interplay between these PR isoforms likely has functional significance, as both PR-A and PR-B are expressed in the human endometrium and can from homo- or heterodimers. Unfortunately, the contrasting functions of the human PR-A and PR-B isoforms are not conserved in all species (Giangrande et al. 1997). This critical difference must be accounted for when translating discoveries from mouse models to human patients and may explain some of the species-dependent discrepancies in PR signaling.
In addition to the well-characterized PR-A and PR-B isoforms, the existence of a third isoform, PR-C, has been postulated (Wei and Miner 1994). The PR-C isoform, once thought to be an artifact, is now known to be transcribed from a TSS downstream from the PR-A TSS, resulting in the production of a 60 kDa protein. The truncated PR-C isoform cannot bind DNA, as it lacks the conserved SHR DBD (Wei et al. 1997). However, PR-C retains the ability to bind progesterone and undergo receptor dimerization, suggesting that it may inhibit progesterone signaling by ligand sequestration. PR-C expression is upregulated in human myometrium during parturition and may contribute to the functional withdrawal of progesterone during the induction of labor (Condon et al. 2006). However, many questions regarding the expression and activity of PR-C have yet to be answered.
3.5.2 PR Is Required for Multiple Reproductive Functions
The use of knockout mouse models has demonstrated that PR is absolutely essential for multiple reproductive functions. Complete knockout of both PR isoforms (PRKO) results in a spectrum of reproductive abnormalities in female mice (Lydon et al. 1995). PRKO mice are completely infertile due to defects in both ovarian and uterine functions. These mice display defective postnatal uterine development, ovulation, and decidualization. In addition, PRKO mice exhibit abnormalities in mating behavior and mammary gland development.
3.5.2.1 The PR-A Isoform Is Necessary and Sufficient for the Establishment of Pregnancy
Specific deletion of the PR-A (PRAKO) and PR-B (PRBKO) isoforms has demonstrated that PR-A, and not PR-B, is required for the establishment of pregnancy in mice. The PRAKO phenotype resembles that of PRKO mice, with complete infertility due to ovarian and uterine defects (Mulac-Jericevic et al. 2000). Intriguingly, PRAKO mice demonstrate increased epithelial proliferation in the endometrium in response to progesterone treatment. This phenotype is in striking contrast to wild-type uterine biology, in which progesterone treatment inhibits estrogen-driven epithelial proliferation. Notably, this proliferative phenotype requires PR-B expression, suggesting that PR-B has pro-proliferative activity that is normally inhibited by PR-A. Interestingly, this suggests that the murine PR-A and PR-B isoforms may exercise antagonizing inhibitory/activating functions, similar to the human PR isoforms. This underscores the importance of PR-A in repressing proliferation of the endometrial epithelium, as it may inhibit both ER-driven and PR-B-driven proliferative activity.
In stark contrast to the infertile PRAKO phenotype, PRBKO mice display normal fertility with no apparent defect in ovarian or uterine function (Mulac-Jericevic et al. 2003). Rather, loss of the PR-B isoform results in abnormal development of the mammary glands, with reduced ductal side branching and limited mammary gland maturation during pregnancy. This recapitulates the mammary gland defects observed in PRKO mice and further supports a role for PR-B in epithelial proliferation. While the murine PR isoforms cannot recapitulate the intricate interactions of the human PR-A and PR-B isoforms, these mouse models have nonetheless demonstrated that the PR-A and PR-B isoforms perform distinct functions during reproduction in rodents.
3.5.3 PR Coregulators and Chaperones Play Important Roles in Early Pregnancy
PR modulates transcription through the recruitment of transcription factors and specific coregulator proteins. Coregulators, either coactivators or corepressors, are specifically recruited by PR in an isoform-dependent manner. The human PR-A isoform exerts its repressive function by recruiting the corepressor, NCOR2 (Giangrande et al. 2000). PR-B, however, recruits steroid receptor coactivator-1 (SRC-1, Ncoa1) to activate transcription at target genes. Knockout mouse models have clearly demonstrated that SHR coregulators play important roles during early pregnancy. Ncoa1 knockout mice are fertile, although they exhibit a decreased decidual response (Xu et al. 1998). Uterine ablation of steroid receptor coactivator-2 (SRC-2, Ncoa2) using Pgr Cre results in infertility due to implantation failure and reduced decidualization (Mukherjee et al. 2006). Of note, crossing these mice to Ncoa1 knockout mice causes complete failure of decidualization, indicating that the coactivators SRC-1 and SRC-2 both play a role in the decidualization of stromal cells.
Chaperone proteins are also essential for optimal PR signaling; these proteins maintain cytosolic PR in an inactive state, prepared to bind ligand. One such chaperone, FK506 binding protein 4 (FKBP52, Fkbp4), forms part of the PR chaperone complex and enhances PR signaling (Barent et al. 1998). Complete ablation of Fkbp4 results in infertility due to implantation failure (Tranguch et al. 2005). Importantly, less progesterone ligand is bound to PR in these mice. Expression of PR target genes is decreased, while ER targets are abnormally upregulated, highlighting the importance of FKBP52 in PR signaling. Interestingly, treatment with excess progesterone can rescue implantation in Fkbp4 knockout mice in a background-dependent manner (Traguch et al. 2007). These findings highlight the importance of chaperone proteins in facilitating optimal PR signaling and bring to light the potentially confounding effects of genetic background.
3.5.4 PR in the Uterus
The roles of PR in the uterus have been further delineated by investigating the signaling pathways that lie downstream of progesterone signaling. These studies have demonstrated that progesterone signaling is absolutely critical during both implantation and decidualization and that PR orchestrates complex intracellular and paracrine signaling networks within the uterus. During implantation, PR activity regulates epithelial proliferation and modulates the activity of ER to establish receptivity. PR also induces signaling molecules in the endometrial epithelium that regulate subepithelial stromal cells to promote the decidual reaction that will take place following embryo attachment. The dynamic expression of PR in the epithelial and stromal compartments highlights the importance of progesterone signaling during both implantation and decidualization. Epithelial PR expression is high in the days preceding implantation, underscoring its importance in the establishment of uterine receptivity. PR expression in the epithelium declines at the time of attachment and instead increases in the endometrial stroma, where PR signaling will play a critical role in the decidualization of stromal cells that surround the implantation site (Fig. 3.3).
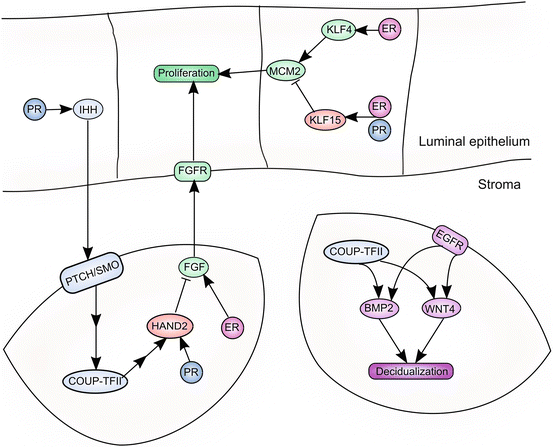
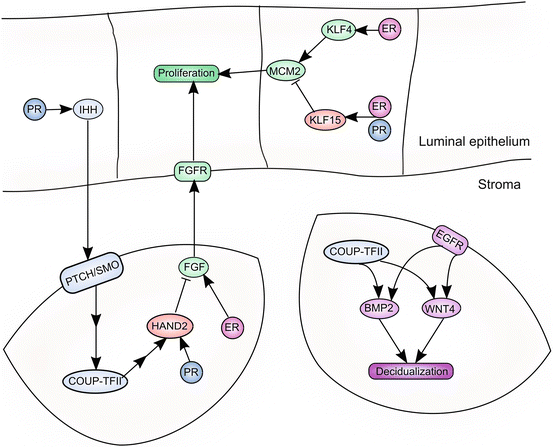
Fig. 3.3
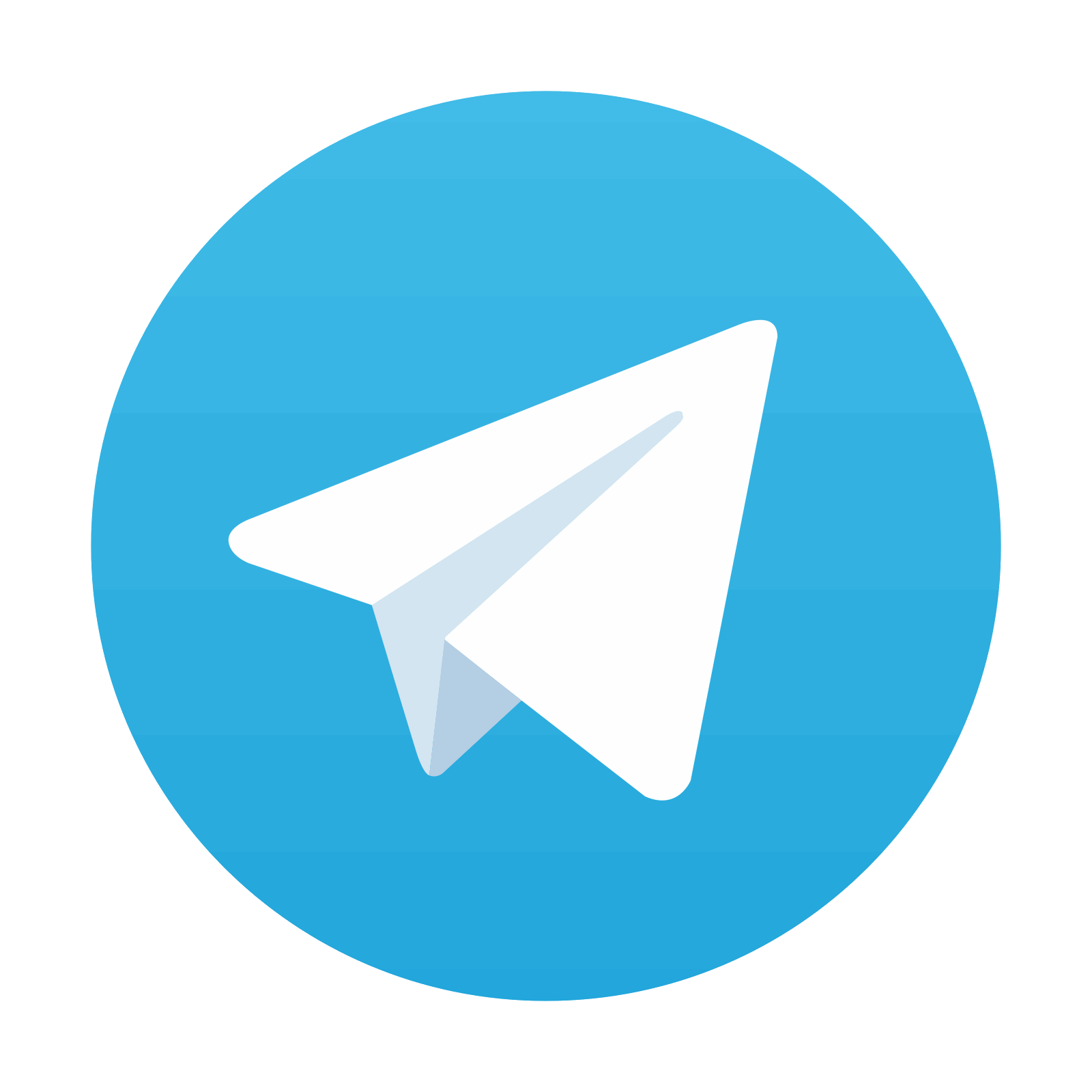
PR regulates multiple signaling pathways in the endometrium and coordinates the functions of the endometrial epithelium and stroma via paracrine signaling networks. Epithelial PR induces expression of IHH, which acts on its receptors, Patched and Smoothened (PTCH/SMO), expressed on stromal cells. This results in induction of COUP-TFII in endometrial stromal cells. HAND2 is induced directly by PR, downstream of COUP-TFII signaling. HAND2 inhibits production of FGF ligands, thereby abrogating proliferative signaling to the epithelium. PR also inhibits epithelial proliferation directly, by favoring production of KLF15, resulting in downregulation of the proliferative target, MCM2. PR signaling also drives the decidualization of stromal cells. COUP-TFII, a downstream target of PR signaling, induces expression of BMP2 and WNT4. Induction of BMP2 and WNT4 also requires EGFR signaling. BMP2 and WNT4 are required for optimal PR signaling and decidualization of endometrial stromal cells
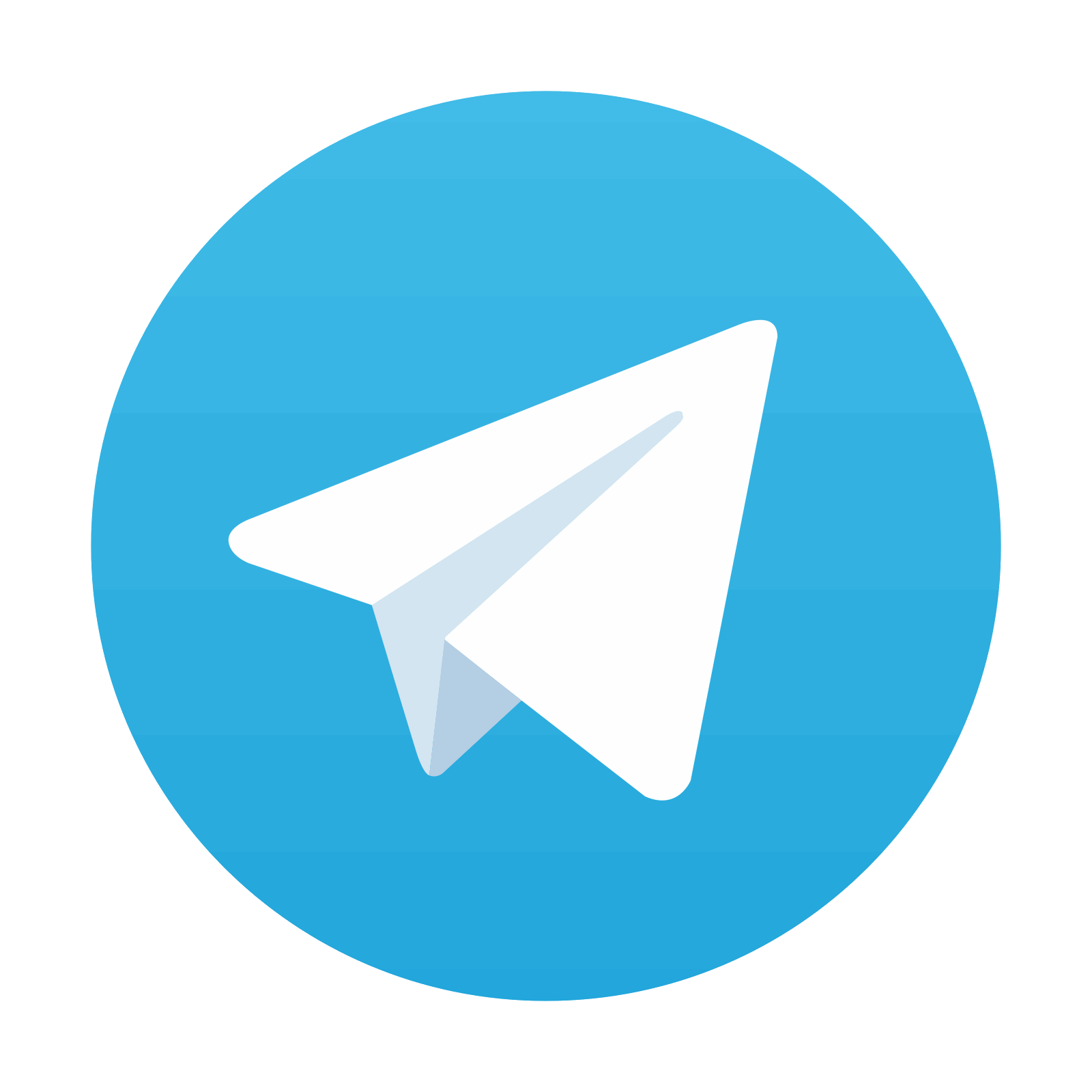
Stay updated, free articles. Join our Telegram channel

Full access? Get Clinical Tree
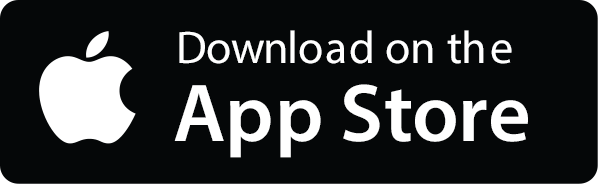
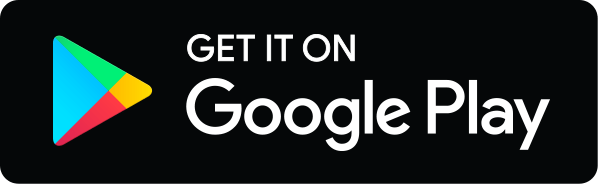
