Fig. 12.1
Photoperiodic and lactational control of diapause in the mink (LHS) and tammar (RHS). In the tammar, the sucking stimulus upregulates prolactin, inhibiting the corpus luteum, initiating and maintaining diapause in the first half of the year. In the second half of the year, photoperiod mediated via melatonin maintains the diapause in response to the duration of melatonin secretion. After the summer solstice (December 22 in the southern hemisphere), the increasing night length reactivates the blastocyst remarkably synchronously and births occur at the end of January. In the mink, photoperiod associated with the spring equinox decreases melatonin secretion and releases prolactin from inhibition. Increased prolactin activates the corpora lutea, which releases progesterone (and other factors) that terminate diapause. In both cases, the growth factors and cytokines in the uterine secretions are highly conserved and mediate the crosstalk between blastocyst and uterine epithelium. SCN suprachiasmatic nucleus, SCG superior cervical ganglion
Entry into diapause was long debated as being caused by the release of an inhibitor (e.g. Spindler et al. 1999; Weitlauf 1994) because blastocysts transferred to quiescent uteri themselves entered quiescence, but there remains no direct evidence of this. In contrast, it appears that it is more likely to be due to the absence of a stimulator (Spindler et al. 1999; Thornber et al. 1981). The cannabinoid anandamide has been suggested as a negative regulator as it is downregulated in the mouse uterus during reactivation (Wang et al. 2003).
Both the tammar and mink blastocyst are surrounded by multiple acellular layers. The tammar does not implant at all, but the placenta attaches to the uterine epithelium at around 18 days after reactivation, and the mink embryo does not implant until some days after reactivation, so the uterine secretions are the only means of transferring signals controlling diapause (Fenelon et al. 2014b; Murphy 2012a, b; Renfree 1973; Renfree and Shaw 2000, 2014; Shaw and Renfree 1986). Due to the importance of this, recent studies have focussed on the analysis of the uterine secretions before, during and after diapause.
12.3 Maintenance of Diapause
Diapause is maintained by a variety of mechanisms. With its 11-month suspension of development, the tammar wallaby has the longest diapause of any mammal so far described but remarkably that can be extended even further. Exogenous progesterone reactivates diapausing blastocysts (Renfree and Tyndale-Biscoe 1973), and when given to ovariectomised animals 2 years after the removal of the ovaries, the blastocysts reactivate (Tyndale-Biscoe and Hearn 1981). This must be amongst the longest time a mammalian blastocyst has remained viable. The fisher, Martes pennanti, also has a post-partum estrus and a long period of delay of 9 months (Frost et al. 2005; Mead 1993), and the badger blastocyst is in diapause for 10 months (Canivenc and Bonnin 1980).
In mice and rats, the period of delay is quite short and variable depending on the number of sucking pups. Prolactin is also an important regulator: when rats are treated with the dopamine agonist, bromocriptine, diapause is inhibited and premature implantation occurs, suggesting that lactation-induced hyperprolactinaemia is responsible for the maintenance of delay (Flint and Renfree 1982). A single intramuscular injection of bromocriptine results in reactivation of the diapausing blastocyst in the tammar (Tyndale-Biscoe 1979). However, although both depend on prolactin, it has opposite effects: in rats, prolactin stimulates progesterone secretion by the corpus luteum during diapause, whereas in the tammar, removal of prolactin allows the corpus luteum escape from its luteostatic inhibition.
During diapause, there are no obvious metabolic changes, no cell division and in most no increase in size. It appears that blastocysts are arrested in G1 phase of the cell cycle, but it is not possible to distinguish between the G0 and G1 phase on DNA content (Surani 1975). Quiescent cells have therefore not been characterised in diapausing embryos for stage of the cell cycle but a new technique describes the potential to measure this using a fusion protein (consisting of mVenus and a mutant cyclin-dependent kinase inhibitor). It will be of interest to apply this to the cells of mammalian diapausing blastocyst cells as it is more likely that they are held in G0.
12.4 Reactivation and Molecular Control of Embryonic Diapause
In most diapausing species including the mouse, estrogen is the nidatory stimulus that causes an increase in uterine secretory activity. However, both progesterone and estrogen are required to stimulate endometrial proliferation and the production of various cytokines that can have both autocrine and paracrine actions to regulate the preimplantation embryo and prepare the endometrium for implantation (Sharkey 1998). In marsupials, or at least in macropodids (the kangaroos and wallabies), progesterone alone is needed to reactivate the dormant blastocyst (Renfree and Shaw 2000). In the tammar and the mink, once progesterone begins to be secreted again, the uterus becomes secretory and the blastocyst resumes development (Fig. 12.1). This is in contrast to the mouse, which requires an estrogen surge for reactivation to occur.
Once the reactivation signal has been received, mitoses increase in mice within 12 h at a time that pyruvate is the main energy source, but after 16 h, glucose is the main energy source (Spindler et al. 1996). In the tammar after reactivation, the process is slower, and there is no significant increase in carbohydrate uptake or production for the first 5 days before a switch to lactate production occurs (Spindler et al. 1995, 1998). In the tammar, RNA synthesis also increases by day 5 (Spindler et al. 1998, 1999).
There is a growing list of known growth factors and cytokines present in the uterus, and all have been shown to influence the development and growth of the preimplantation embryo in eutherian mammals. Some of these presumably control the arrested growth that occurs in diapause. These include epidermal growth factor (EGF and HB-EGF), leukaemia inhibitory factor (LIF), insulin-like growth factor (IGF), platelet-derived growth factor (PDGF), fibroblast growth factor (FGF), platelet-activating factor (PAF), transforming growth factor β (TGF-β), interleukin-1ß (IL1B), bone morphogenic protein-2 (BMP2), prostaglandin synthetase PTGS2 (COX2), fibroblast growth factor (FGF), signalling molecules of the wingless (WNT) family and the transcriptional regulators Msx1 and Msx2 (Cha and Dey 2014). Many of these are also present in the blastocyst, and the expression of a number of them is now known to coincide with reactivation.
HB-EGF is produced in the luminal epithelium of the uterus at the site where the blastocyst will attach. It is upregulated at reactivation (Hamatani et al. 2004) and in the endometrium 6–7 h before implantation (Das et al. 1994). Blastocyst signalling to the uterus prepares it for implantation, since HB-EGF is upregulated in the endometrium by the blastocyst which then binds to its receptors ERBB1 and ERBB4 on the blastocyst. However, although blastocysts need to have hatched from the zona before attachment to the luminal epithelium, this is not needed to induce HB-EGF because the induction begins before zona dissolution. Bovine blastocysts also have this EGF receptor-ligand system during the time of trophoblast elongation (Kliem et al. 1998), so clearly EGFs have a wider role than just during diapause. Vascular endothelial growth factor (VEGF) induces endothelial proliferation and vascularization in the uterus and is upregulated in the mink at reactivation (Lopes et al. 2003, 2006) and in the tammar (MB Renfree, H Clark, G Shaw, LJ Parry, SR Frankenberg, AJ Pask, unpublished results).
Leukaemia inhibitory factor (LIF) has multiple roles in regulating blastocyst implantation, diapause and blastocyst viability in mice (Batlle-Morera et al. 2008; Hondo and Stewart 2005; Nichols et al. 2001). Estrogen stimulates the release of LIF from the endometrial glands. LIF activates the STAT3 pathway as well as the ERK pathway. LIF is secreted into the uterine lumen where it binds to two heterodimeric LIF transmembrane receptor complexes (LIFRs) and gp130 (Rosario et al. 2014). LIF is required for implantation in mice, binding to a heterodimer of LIF receptor and IL6ST that are expressed in the blastocyst (Nichols et al. 1996, 2001). In the absence of LIF, mouse blastocysts enter diapause but blastocysts lacking gp130 do not survive (Hondo and Stewart 2005). LIF mRNA is expressed at very low levels during diapause, and its expression increases in the endometrium at the termination of diapause in the mouse, mink, Western spotted skunk and the wallaby (Bhatt et al. 1991; Hearn 2005; Hirzel et al. 1999; Passavant et al. 2000; Song et al. 1998; Stewart et al. 1992) (MB Renfree, H Clark, G. Shaw, SR Frankenberg, CM Hearn AJ Pask, unpublished results). In the skunk, LIF receptor β (LIFRB) increases in the uterus when blastocysts resume development, apparently under prolactin control (Passavant et al. 2000). In the mouse, LIF expression appears to be under estrogenic control and can replace the estrogen injection to induce reactivation (Chen et al. 2000). LIF induces changes in gene expression, downregulating 54 genes in the first hour after treatment, and upregulating 256 genes including Sox, Kfl, Hes, Hey and Hox families of transcription factors (Rosario et al. 2014). Thus, LIF has multiple roles and activates pathways in the luminal epithelium of the uterus to induce a dynamic and complex network of changes essential for reproduction (Rosario et al. 2014) and has an important role in embryonic diapause.
The muscle segment homeobox-1 (MSX–1 and MSX–2) genes are highly conserved transcriptional regulators of some of the uterine implantation factors, and uterine receptivity requires downregulation of Msx1 expression (Cha et al. 2013; Daikoku et al. 2011). Lack of Msx1 affects uterine receptivity by disrupting Wnt signalling through Wnt5a (Cha et al. 2013; Nallasamy et al. 2012). Interestingly, it appears that the function of Msx is to limit uterine stress-mediated inflammatory responses and so to maintain diapause (Cha et al. 2015). Msx1 and Msx2 are found in the uteri of mice, mink and tammar wallabies during embryonic diapause (Cha et al. 2013; Fenelon et al. 2014a; Renfree and Shaw 2014). In mice without diapause, Msx expression is transient early on day 4pc, but during diapause, it is highly expressed, and in its absence, there is reduced blastocyst recovery and survival (Cha and Dey 2014; Cha et al. 2013). Msx and LIF interact, but in LIF knockout mice, Msx1 continues to be expressed (Cha et al. 2013; Daikoku et al. 2011). LIF injection induces implantation and downregulates Msx1/2 expression (Cha et al. 2013). In mice and mink, the predominant gene is Msx1, but in the tammar, it is MSX2 that remains high during diapause but decreases steadily up to day 5 after reactivation. The MSX genes are highly conserved in mammals, but these two genes may have developed subtly different actions as diapause evolved. Since these divergent mammals have been separated for at least 160 million years (Luo et al. 2011), this appears to be an ancestral mechanism that may be widespread in diapausing species.
Platelet-activation factor, or PAF as it is now known, is a phospholipid that is present in the endometrium. PAF receptor (PTAFR) interacts with PAF in the embryos of rodents, rabbits and humans (Ammit and O’Neill 1991; Jin and O’Neill 2011; O’Neill 1985, 1991, 2005). PAF may be important for maternal recognition of pregnancy since it stimulates embryonic metabolism, cell proliferation and viability (O’Neill 1991). PAF production and release is dependent on progesterone and estradiol (Chami et al. 1999; Li et al. 1999). In the diapausing tammar embryo, PAF is low, but endometrial PAF increases around the time of reactivation (Fenelon et al. 2014b; Kojima et al. 1993). It has a reciprocal expression pattern with MSX (Renfree and Shaw 2014). It is present in culture media from endometrial cultures and although variable appears to increase at the time when the first mitoses are observed after blastocyst reactivation (Kojima et al. 1993; Spindler et al. 1996). The release of endometrial PAF appears to upregulate PTAFR expression which is internalised with local cytoplasmic expression in the perinuclear region of the blastocyst cells at reactivation (Fenelon et al. 2014b). Whilst this does suggest that endometrial PAF is involved in reactivation, there is as yet no information as to whether it is essential.
The polyamines putrescine, spermidine and spermine are factors that regulate cell cycling and protein synthesis, and uterine polyamine-related genes appear to be important for embryo implantation (Igarashi and Kashiwagi 2010; Mandal et al. 2013; Zhao et al. 2008). These are candidates for controlling the resumption of development after diapause. Polyamine synthesis is rate limited by the ornithine decarboxylase-1 (ODC1) gene (Lefèvre et al. 2011a, b). They have many functions in reproduction including in spermatogenesis, sperm motility, fertilization, onset of puberty and folliculogenesis (Lefèvre et al. 2011b). ODC1 and putrescine are induced by estradiol-17β and are upregulated at reactivation in the mouse (Van Winkle and Campione 1983) and in the mink (Fenelon et al. 2014a; Lefèvre et al. 2011a, c; Murphy 2012b).
Polyamines may act by inhibiting cell proliferation and arrest of the cell cycle in diapause (Fenelon et al. 2014a; Lefèvre et al. 2011a; Lopes et al. 2004). Since polyamines are essential regulators of cell proliferation and growth, evidence for their role in diapause comes from experimental manipulation of mink diapause. Ornithine decarboxylase inhibitor treatment reduces polyamine levels in the uterus, and in the mink, it rearrests cell proliferation even after reactivation only as long as the inhibitor is given: if withdrawn, the embryos resume development (Lefèvre et al. 2011a). Similarly, mink trophoblast cells in vitro do not proliferate. Administration of this inhibitor also arrests embryo development in the mouse, rat and hamster (Fozard et al. 1980; Galliani et al. 1983; Lopez-Garcia et al. 2008; Reddy and Rukmini 1981) showing that polyamines are critical factors in the reactivation of diapausing blastocysts. Anandamide and other cannabinoids also appear to have a role in cessation of embryonic development in diapause. They are downregulated in the mouse uterus during reactivation and conversely inhibit reactivation in vitro (Wang et al. 2003).
12.5 Genes and miRNAs in Diapause and Reactivation
There are many more factors yet to be discovered. A suite of genes have been identified in the diapausing mouse blastocyst and after reactivation using microarray analysis (Hamatani et al. 2004; Hondo and Stewart 2005). Only 229 (1 %) of the >20,000 genes examined were differentially expressed between blastocysts in diapause (80 genes) and reactivated blastocysts (149 genes) (Hamatani et al. 2004). These 229 genes consisted of major functional categories, including the cell cycle, cell signalling, adhesion molecules and metabolic pathways. One of the earliest genes upregulated at implantation is interleukin-1 (IL-1) of embryonic origin which modulates endometrial cell responsiveness (Bourdiec et al. 2013). In mink, there are 123 genes differentially expressed between diapause and reactivation. Almost half of the genes appear to be secreted products including those involved in cell proliferation, homeostasis, protein folding, electron transport and the innate immune response (Lefèvre et al. 2011c). Specific proteins involved in chromatin and tissue remodelling are changed at reactivation. SPARC (secreted protein acidic and cysteine rich), a secreted glycoprotein, increases, perhaps as a result of progesterone secretion from the reactivation of the corpus luteum (Lefèvre et al. 2011c), and HMGNI (high mobility group nucleosome binding domain 1), a chromatin remodelling factor, is also upregulated in the uterine epithelium at reactivation.
MicroRNAs may also be involved, since miRNAs suppress translation. There are 45 differentially expressed miRNAs between diapause and reactivation, 38 of which are downregulated at reactivation (Liu et al. 2012). Of nine members of the tumour suppressor miRNA family lethal (let) 7, five are downregulated at reactivation of mouse-diapausing embryos (Liu et al. 2012). Let 7 inhibits attachment, and its targets include genes that regulate cell proliferation (Gurtan et al. 2013). The gene data set has now been extended by a proteomic analysis of mouse blastocysts during diapause and after activation (Fu et al. 2014). This study of 6000 mouse blastocysts identified 2255 proteins that have differential regulation of the protein translation, aerobic glycolysis, pentose phosphate pathway, purine nucleotide biosynthesis, glutathione metabolism and chromatin remodelling. Interestingly, reactivation is accompanied by activation of mitochondria and of the endosome-lysosome system (Fu et al. 2014).
12.6 Evolutionary Origins of Diapause
Although each species has its own specific diapause controls, there are enough factors conserved to suggest that diapause may have been an ancestral condition in mammals (Ptak et al. 2012). Transfer of sheep blastocysts to the uteri of mice in diapause induces a period of quiescence of the sheep blastocyst until reimplantation into sheep uteri (Ptak et al. 2012), and these authors suggest that the potential for diapause is not restricted to the species where it is known to occur. Ptak et al. (2013) further agree with the suggestion by Tarin and Cano (1999) that human conceptuses may be able to enter diapause. As yet there is not widespread acceptance of these suggestions.
12.7 Maternal Recognition of Pregnancy in Diapausing Species
Most embryos in diapause, by definition, are totally quiescent so there is no obvious uterine response to their presence. However, once they reactivate, the blastocyst engages in considerable “crosstalk” with the uterus that in most species results in changes to the uterine milieu and implantation, effectively a delayed maternal recognition. The nature of the signal in mammals is varied, from the HCG of humans to the interferon tau (IFN-τ) of ungulates which prevents luteolysis (Flint 1995). This delay of maternal recognition of pregnancy seems likely in the roe deer at least, because it enters diapause after hatching, but at a stage when other ruminants do not produce IFN-τ and when there is no IFN-τ detectable in the uterine secretions (Flint et al. 1994). It is therefore likely that there is no maternal recognition of pregnancy in the formal sense in any delayed species, but that instead it occurs later when reactivation occurs.
This is certainly the case in the tammar wallaby but the timing is even later. Maternal recognition of pregnancy was long thought of as a eutherian-specific character because the signals occur early in pregnancy and around the time of implantation. In most marsupials, since there is no “implantation” or erosion of the uterine endometrium, but rather an attachment of the placental membranes to the uterine epithelium, it was tacitly assumed that these mammals had no maternal recognition of pregnancy. Further, the fact that the estrous cycle progresses uninterrupted in marsupials further hindered interpretation of specific embryo-maternal interactions. Closer examination of the changes in uterine endometrial proliferation and secretion in the tammar shows there is indeed a maternal recognition of pregnancy that is a direct response to the presence of an expanded embryonic vesicle in the gravid uterus, although the specific signalling molecule(s) is not yet identified (Renfree 1972, 2000). Thus, the answer to Brian Heap’s question is that the embryo appears to withhold evidence of its existence during diapause, but that a maternal recognition of pregnancy occurs later after reactivation at a time that is species specific.
12.8 Summary and Outlook
Understanding the way in which the mammalian embryo can be held in a state of suspended animation has progressed in just a few selected species, including nontraditional laboratory species. Whilst the external factors are now well understood, the increasing number of cytokines and growth factors emanating from both the uterine epithelium and the trophoblast and their cellular effects are still being discovered. In addition, the roles of the specific uterine proteins are only now being subjected to proteomic and transcriptomic analysis. However, there are potential therapeutic applications for holding cells in quiescence, such as in certain tumours, but these opportunities have yet to be recognized. Continued studies of this amazing phenomenon are awaited with interest.
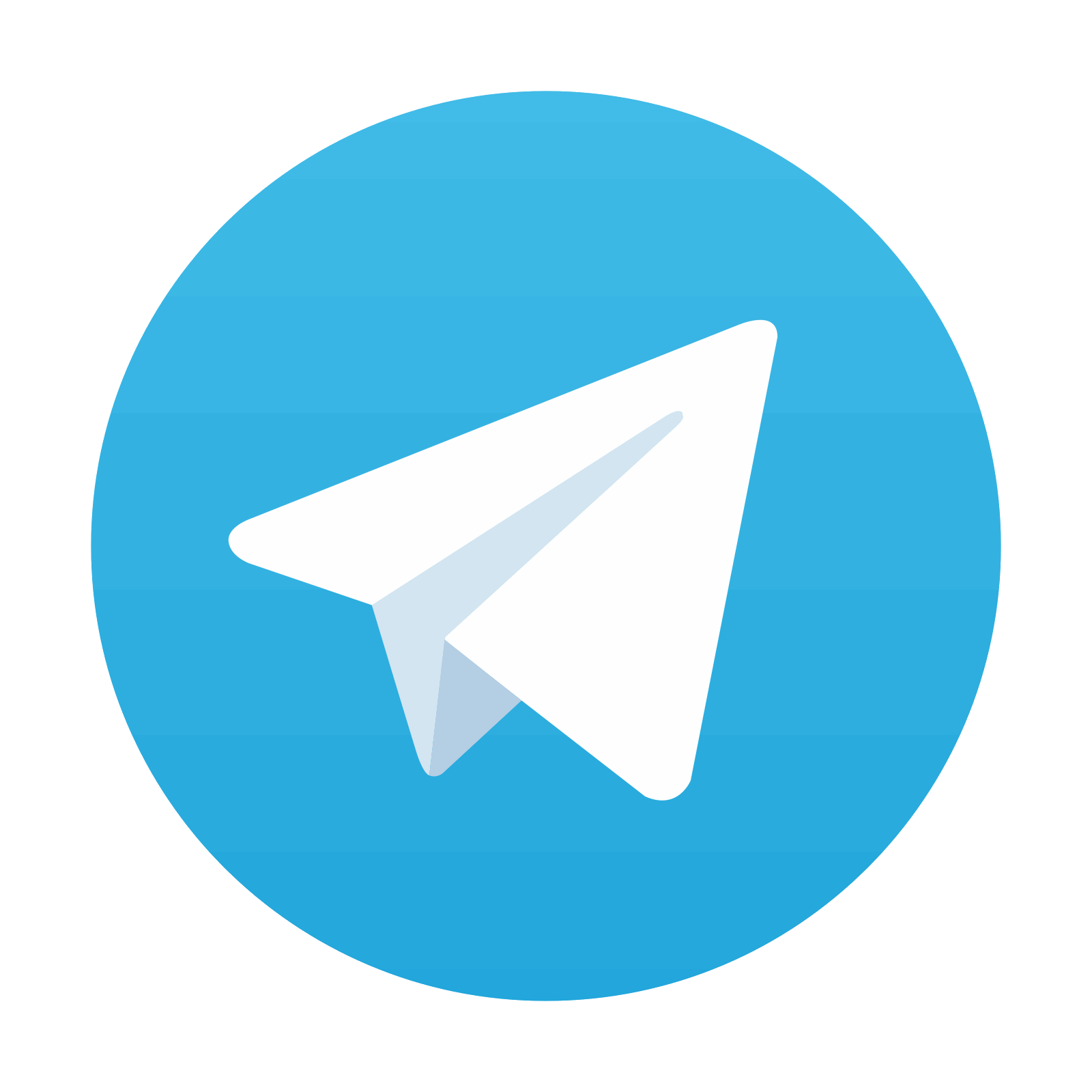
Stay updated, free articles. Join our Telegram channel

Full access? Get Clinical Tree
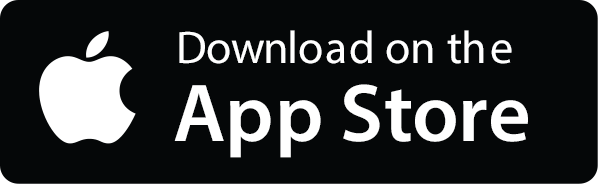
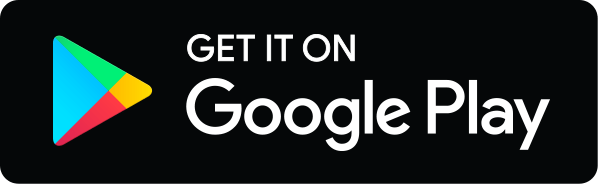