Fig. 24.1
Fetal liver (3° trimester). a Periportal space (arrowhead) and hepatic vein (arrow). b Intrahepatic hemopoiesis and mature biliary ducts (arrow) haematoxylin–eosin stain
From 5th to 9th gestational week a rapid growing of the organ is seen, with the liver reaching up to 10 % of the overall embryo weight. At the third trimester this growth slackens, and the liver reaches the 5 % of the final newborn’s weight [3]. The development of intrahepatic biliary tree proceeds centrifugally from the porta hepatis to the peripheral branches of portal tracts. At birth, the biliary system is still not fully developed, and Keratin 7-positive structures may not be visible in peripheral portal structures until the first month after birth [1].
Many molecules and several molecular pathways have been identified to play a role in liver development. The Nodal signaling pathway—included in the TGFβ[beta] family—is involved in the progression of the definitive endoderm, via Alk4, Alk7, or ActRII A and B, driving the commitment of the endodermic and mesodermic layers [2]. The Nodal signaling is finely regulated by several molecules, such as fibroblast growth factor (FGF) or bone morphogenetic protein (BMP) [2]. The foregut formation in animal models seems to be related to the low expression of β[beta]-catenin in the anterior side of endoderm during gastrulation phase: the low β[beta]-catenin activity in this region causes an upregulation of the Wnt antagonists of the secreted frizzled-related protein (SFRP) family, as well as DKK-1, key molecules in foregut development [2].
The bipotential differentiation od hepatoblasts in hepatocyte or biliocyte is regulated by TGFβ[beta], Wnt, Notch, FGF, and BMP, among others. In particular, an increased TGFβ[beta] activity around the portal tracts together with an increased activity of the Notch and FGF signaling are linked to biliocyte differentiation and proliferation, while the HGF and TNFα[alpha] signaling promote hepatocyte differentiation [6, 7].
Furthermore, mouse models revealed other mechanisms involved in the following phases of hepatocytic differentiation, including Wnt signaling, FGF, BMP, and FoxA2 [2, 8–10], while c-Jun, the hepatoma-derived growth factor (HDGF), the nuclear factor kB (NFkB), the c-Met pathway, and HNF4α[alpha] were all described during the phases of liver growing [11, 12].
24.3 Anatomy and Histology of the Liver
In adults, the liver accounts for approximately the 2 % of the total body weight, with a mean weight of 1400–1600 g [3]. The liver is located in the right upper quadrant of the abdomen, completely covered by the ribs in nonpathological conditions. The canonical anatomic division of the liver includes a right and a left lobe, respectively, lateral and medial to the falciform ligament, as well as the smaller quadrate and caudate lobes. This topographical division has been progressively replaced by the more recent division in eight functional segments: each segment depends on specific branches of the hepatic artery, the portal vein, and the biliary tree. Thus, this division follows the anatomy of the vascular and biliary branching and not the gross anatomy of the organ. Therefore, it is important for surgical reasons, especially when small portions of the liver parenchyma must be resected. According to the segmental division, the liver is not divided in left and right lobes (with the falciform ligament as mark), but in left and right hemiliver, according to the first branching of the portal vein at hilum. The left hemiliver incorporates segments II, III, and IV, the right hemiliver the V, VI, VII, and VIII; caudate lobe is segment I. According to some authors, the quadrate lobe should be regarded as a separate segment [1, 13].
Histologically, the liver lobule was described as the fundamental unit of the liver since seventeenth century [1]: the canonical lobule is conceived as an hexagon with portal tracts in each angle—each made by a terminal branch of the liver artery, a terminal branch of the portal vein, and a bile duct—and a central vein. The modern acinar concept was introduced by Rappaport in the middle-twentieth century: the “Rappaport acinus” is seen as a triangle, with two portal tracts in two angles and the hepatic vein (called “terminal vein”) in the third (Fig. 24.2). This approach is more functional, since it is based on the blood flow (and the hepatocyte oxygenation), which goes from the portal tracts to the terminal vein dividing the hepatocytes layers into three zones, from “zone 1” (closer to the portal tract and more oxygenated) to “zone 3” (closer to the vein and less oxygenated) [14]. Although many other systems have been proposed for the classification of the liver functional unit, the “Rappaport acinus” still represents the most utilized since it provides a strong link between liver microanatomy and liver pathology (e.g., fibrosis progression, toxic and ischemic damage, etc.) [1].


Fig. 24.2
The “Rappaport acinus” in a liver with normal architecture is represented as a triangle, with two portal tracts in two angles and the terminal vein in the third, according to the blood flow. Haematoxylin–eosin stain
In adults, hepatocytes are large polygonal cells, with wide eosinophilic cytoplasms, disposed in one-cell thick layers. Each heaptocyte has vascular and biliary “poles” that are properly shaped to create the sinusoids and the terminal bile ductules when the cells are juxta posed in layers. The sinusoids form a vascular network between these hepatocyte plates, surrounding them with a very specialized endothelium, with sieve-like cytoplasm and without a continuous basal membrane, that leads the blood flow toward the terminal veins. The biliary spaces created between two hepatocytes bring the bile toward the portal tracts where it flows into the bile ductules [3]. All these microanatomical features warrant the interrelations among hepatocytes portal blood flow and bile flow.
24.4 Histopathology of Precursor Lesions: Regenerative Nodules and Dysplastic Nodules
Liver cirrhosis represents the main clinical condition leading to hepatocellular carcinoma (HCC) in developed countries (Fig. 24.3). Foci of cells with atypical features can be present in cirrhotic regenerative nodules (RNs). Although these hepatocytes may be enlarged with prominent nuclei, their nucleus-to-cytoplasm (NC) ratio is generally preserved with only a little reduction of the amount of cytoplasm present. These features can be ascribed to hepatocyte degenerative and/or regenerative changes rather than to true cell dysplasia [15]. Therefore, the diagnosis of dysplasia is recommended only when these changes involve an entire liver nodule or at least a large focus within a nodule [16].


Fig. 24.3
Gross features of a cirrhotic liver: the parenchyma is replaced by regenerative nodules, with evidence of a major HCC nodule (orange arrow); a distant neoplastic thrombosis is visible as well (red arrow)
The distinction between a large RN and a low-grade dysplastic nodule (LGDN) is not achievable at the clinical or histopathological level, since the only significant difference is that an LGDN is clonal while an RN is not. Because clonality assays are expensive and time-consuming, and since an LGDN is considered a lesion with very low risk of progression to HCC, the distinction between an RN and an LGDN is considered of little clinical relevance. These lesions are by definition bigger than 1 cm in size (alternatively, they must be clearly identifiable in the cirrhotic parenchyma regardless of size), and should lack the histological and architectural characteristics of a high-grade dysplastic nodule (HGDN) [17, 18]. LGDNs still display hepatocyte plates no more than two-cell thick, without trabeculae or acinar structures and surrounded by a preserved reticulin network. The portal structures are readily recognizable within the nodules.
HGDNs are macroscopically similar to RNs and LGDNs, but they are characterized by dysplastic cytology. Histologically, these nodules are often hypercellular [19]. HGDNs may focally loose the reticulin network, with hepatocellular plates comprised of three or more cells in thickness. Unpaired arteries can be seen associated with an increased sinusoidal endothelialization [20]. These subtle features may not always be visible a small biopsy, making diagnosis challenging when diagnostic specimen are limited. The most important differential diagnosis of a HGDN is with early HCC (eHCC). This will be discussed below.
24.4.1 Molecular Pathology of Precursor Lesions
The process of tumor initiation and progression in cirrhosis is well established. Progressive accumulation of genetic and epigenetic abnormalities marks the temporal steps of hepatocarcinogenesis [1]. In the early stages of hepatocarcinogenesis the epigenetic mechanisms of gene expression upregulation prevail, involving particularly the transforming growth factor-α[alpha] (TGF α[alpha]) and the insulin-like growth factor-2 (IGF-2). Chronic hepatitis (both viral and nonviral) and cirrhosis are considered predisposing situations, since the combined action of inflammatory cytokines, viral transactivation, and regenerative response can determine changes in telomerase length, gene methylation, microsatellite instability, and fraction of alleles that were observed both in hepatocytes during chronic inflammation and cirrhosis, and in dysplastic nodules as well as HCC [21]. In these inflammatory situations there is an increased expression of DNA methyltransferases (DNMTs), that catalyze the methylation and demethylation of CpG groups, and both DNMT1 and DNMT3a are upregulated in HCC [21].
Loss of heterozygosity (LOH) and microsatellite instability (MSI) are detectable in preneoplastic liver lesions, with the most frequent deletion occurring at 8p, both in HGDNs and HCC. Similarly, RNs, dysplastic nodules, and adjacent HCC may share an MSI phenotype at identical loci and similar allelic deletions or gene mutations. Oncogene activation (v-akt homolog 2), loss of tumor suppressor genes (WT1, RB1), and DNA repair genes are deregulated in HGDN. Similarly, the expression at the protein level of growth factors (EGFR, IGFBP3) cytokines, adhesion proteins, signal transduction proteins, transcription factors, and housekeeping genes is altered in precursor and neoplastic hepatocellular lesions [1, 21–23]. The AKT-mTORC1 signaling pathway is activated in cirrhotic liver with and without HCC. As a result, increased expression of phospho-S6 (an AKT effector) was observed, while PTEN staining was negative in 24 % of HCC cases [23].
Allelic deletions are rare in chronic hepatitis and cirrhosis, but their number steadily increases in dysplastic nodules and in HCC and that suggests that HCCs often arise as clonal outgrowths of cirrhotic (dysplastic) nodules. Although several of the these structural alterations in preneoplastic cell populations differ from those found in adjacent HCCs, suggesting that most of the cells harboring the early genomic aberrations do not evolve into the malignant phenotype [21].
Hepatocyte dysplasia, especially the “large-cell” HGDN, is often accompanied by alterations that can also be found in HCC, such as telomerase shortening, microsatellite instability, inactivation of cell cycle checkpoints CDKN2A and CDKN1A, resulting in increased proliferation index [22]. However, the most common genetic alterations in HCC (i.e., β[beta]-catenin or TP53 mutations) have never been described in preneoplastic liver lesions [24].
24.5 Hepatocellular Carcinoma
24.5.1 Gross Appearance
The macroscopic features of HCC largely depend on the status of the surrounding liver. In cirrhotic livers, HCC is seen as a single lesion or as multiple lesions, and is encapsulated, resembling larger cirrhotic nodules (Fig. 24.3). The neoplastic nodules are soft, with a grayish-white to greenish color, according to the amount of bile content. The macroscopic diagnosis of HCC in a cirrhotic background is not always easy, especially in eHCC < 2 cm in size. HCC in noncirrhotic livers is usually a single large mass, with irregular, poorly defined borders, sometimes with satellite lesions and grossly visible vascular invasion. Vascular invasion is a common feature of any HCC at the time of diagnosis, involving the portal and the hepatic veins, or the vena cava [15].
24.5.2 Histopathology of “Early HCC”
Small nodules (≤2 cm) within a cirrhotic background with the architectural and cytologic atypical of a HGDN, but affecting 100 % of the cells, is classified as (eHCC) [19]. eHCC is by definition grade 1 according to the Edmondson and Steiner grading system (see below). At a histological level, hypercellularity, loss of reticulin staining, and the presence of hepatocellular trabeculae more than two-cell thick are helpful diagnostic criteria if present throughout. In addition, no portal tracts are visible within the lesion. Nearly half of eHCCs show fatty changes (macrovescicular steatosis) [24]. An important diagnostic feature is the occurrence of stromal invasion, defined as the presence of HCC cells in the stroma of the surrounding portal tracts (or in fibrous septa) [25]. Although stromal invasion is the most specific histological feature for the diagnosis of eHCC, it is not always recognizable on needle biopsies, and even when present, it is difficult to distinguish from non-neoplastic hepatocytes entrapped within the fibrosis.
24.5.3 Histopathology of Advanced HCC
Unlike many other solid tumors the neoplastic cells in HCC are quite similar to the non-neoplastic hepatocytes, i.e., they are polygonal cells with a variable amount of eosinophilic granular cytoplasm and large polymorphic vesicular nuclei, with open chromatin and one or more prominent nucleoli. Many cell features seen in not-neoplastic hepatocytes are common also in HCC, such as Mallory bodies, hyaline bodies, glycogen inclusions, fatty changes, bile production, and nuclear inclusions [15].
HCCs are routinely graded according to the original Edmondson and Steiner system of 1954 [26]. Like the grading systems of other cancers, Edmondson and Steiner’s is based on the dedifferentiation of the neoplastic hepatocytes, i.e., their progressive loss of resemblance with the normal hepatocyte (Fig. 24.4). More practically, the grade of HCC is based on the nuclear-to-cytoplasmic ration, nuclear hyperchromasia, and cell polymorphism. Grade 1 HCCs are characterized by neoplastic hepatocytes with preserved eosinophilic cytoplasms, mild (or no) atypia, while in grade 2 and 3 HCCs a progression of the above-mentioned features is observed. Grade 4 HCCs include anaplastic, small-cell and giant-cell tumors.


Fig. 24.4
Examples of HCCs with progressive Edmonson and Steiner grade. G1 HCCs (a) are characterized by hepatocytes with low atypia and low nuclear-to-cytoplasmic ratio; early HCC often show steatosis. G2 (b) and G3 (c) HCCs show a progressive increase in atypia, nuclear-to-cytoplasmic ratio, and polymorphism. Haematoxylin–eosin stain
The architectural appearance of HCC is variable, with different, often intermingled patterns. The trabecular, acinar, and solid growth patterns are recognized. The most common pattern, particularly in well-to-moderately differentiated HCC, is the trabecular pattern, characterized by cords of neoplastic cells surrounded by vascular sinusoids [15]. These cords resemble the normal hepatic trabeculae, but are generally more than two neoplastic cell thick, with a high N/C ratio and nuclear overcrowding. With tumor progression, the neoplastic trabeculae can become distorted, creating a pseudoglandular pattern. In the acinar growth pattern, the neoplastic hepatocytes form luminal structures resembling bile canaliculi. Interestingly, bile production is detectable in nearly half of the cases simply by light microscopy [1]. These acini are not true glands, hence the term pseudoglandular, and may contain bile. Their biliary derivation can be demonstrated by electron microscopy or by the immunoreaction for CEA. The acini may contain PAS-positive proteinaceous fluid as well. The solid pattern is the least frequent, and occurs in poorly differentiated HCC, generally in association with at least one of the other architectural patterns. In the solid pattern the tumor cells are densely aggregated, without visible trabeculae or sinusoids. Immunostaining for CD34, however, often reveals compressed sinusoids within the lesion [22]. Other histological variants of HCC include: fibrolamellar HCC, scirrhous HCC, sarcomatoid HCC, clear-cell HCC, giant-cell HCC, HCC with a predominant inflammatory infiltrate, HCC with predominant hematopoiesis [1].
24.5.4 Diagnostic Markers
Due to the lack of strong diagnostic criteria, the differential diagnosis between HGDN and eHCC is challenging, and often requires immunohistochemistry (IHC). The American Association for the Study of Liver Diseases (AASLD) and the European Association for the Study of the Liver/European Organization for Research and Treatment of Cancer EASL/EORTC guidelines recommend the use of the three markers: Glutamine Synthase (GS), Glypican-3 and heat shock protein-70 (HSP70) [17, 18]. The combination of these three markers is considered sensitive and specific for the diagnosis of HCC in small liver lesions, while their diagnostic value in biopsy samples is less valuable (Fig. 24.5a–l). Although additional markers, such as EZH2 (Fig. 24.5m–o) [27] and Agrin [28] have been proposed, the differential diagnosis between a HGDN and eHCC in needle biopsies still represents a major challenge for the pathologist. A new promising class of biomarkers is represented by the cleavage products of the endoplasmatic reticulum protein, in particular calreticulin, PDIA3, PDI, and GRP78 [23]. A role as potential biomarker for HCC was postulated for the ubiquitin-conjugating enzyme E2C (UBE2C) due to its overexpression in human HCC at significantly higher levels compared to normal hepatocytes. In addition, high-UBE2C levels were associated with significantly lower disease-free survival rates [23].


Fig. 24.5
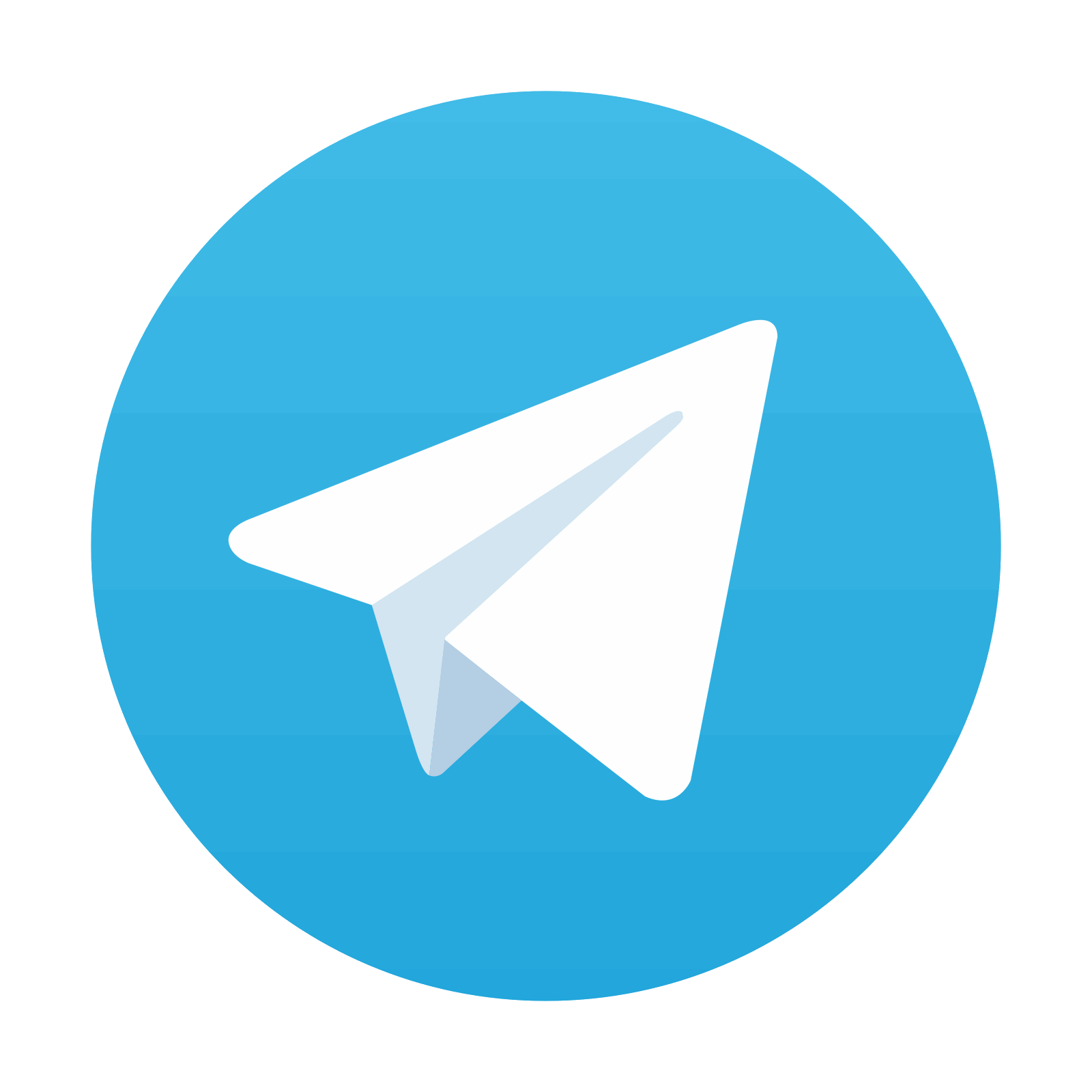
Histological appearance at haematoxylin–eosin of a (a) Regenerative Nodule (RN), a (b) High-Grade Displastic Nodule (HGDN), and a (c) Hepatocellular Carcinoma (HCC), and expected immunohistochemistry pattern. Glutamine Synthase shows positive reaction only in periportale, perivascular, and perifibrotic areas in RN (d) and HGDN (e), while it is diffusely positive in HCC (f). Glypican-3 is completely negative in RN (g) and HGDN (h) and diffusely positive in HCC (i). Heat shock protein-70 is completely negative in RN (j) and HGDN (k) and variably positive in HCC l. EZH2 is completely negative in RN (m) and HGDN (n) and shows variable nuclear positivity in HCC (o)
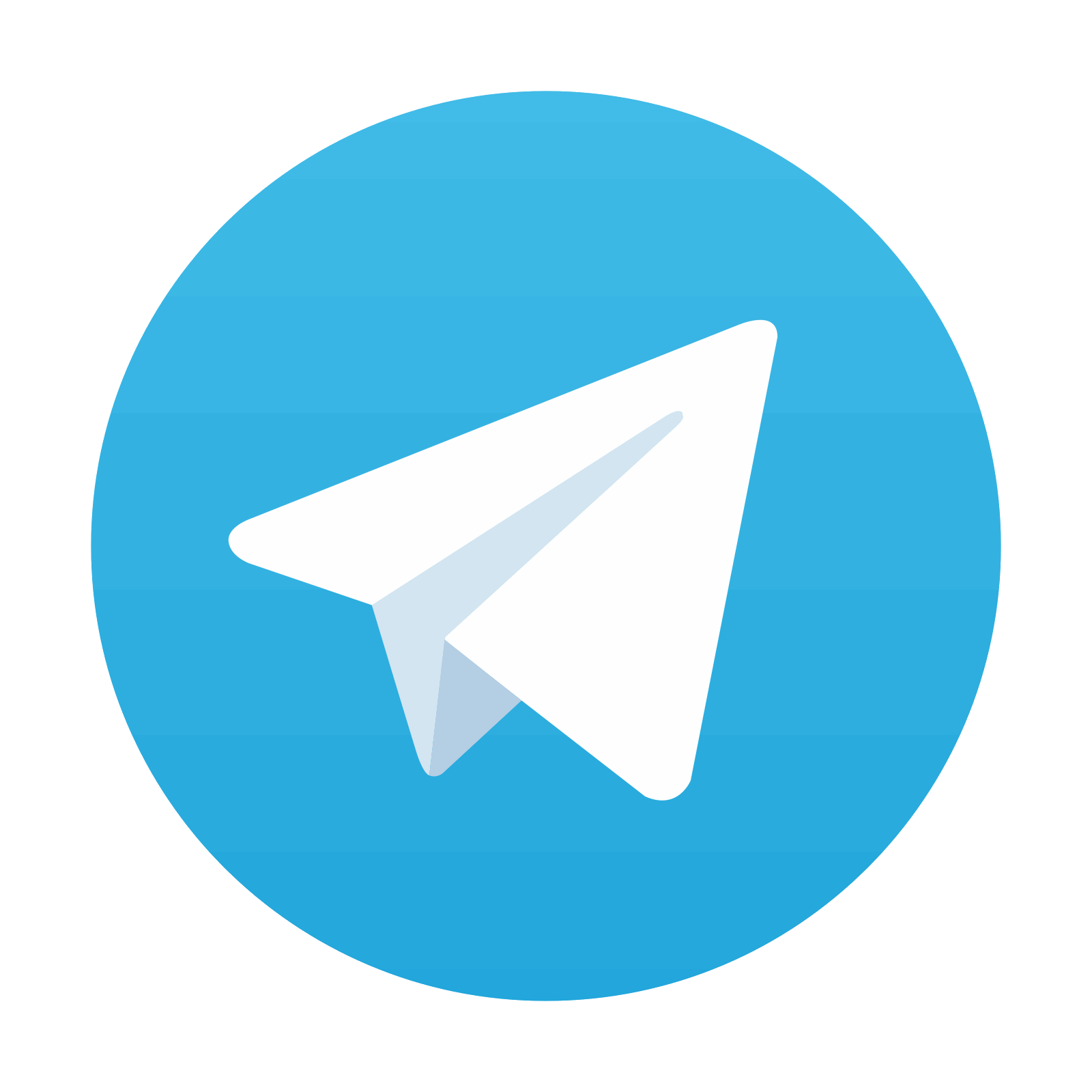
Stay updated, free articles. Join our Telegram channel

Full access? Get Clinical Tree
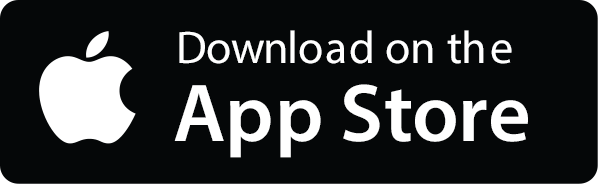
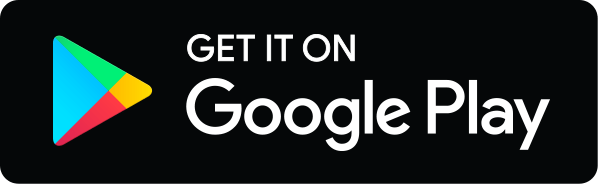
