Stereotactic Irradiation of Tumors Outside the Central Nervous System
Departing from the established traditions of conventionally fractionated external beam radiotherapy, in the late 1980s and early 1990s investigators in the United States, Sweden, and Japan began to explore the use of extremely brief hypofractionated radiation treatment regimens for spine, lung, liver, and selected other malignant extracranial tumors.1–3 In essence these clinical researchers were modifying techniques proven clinically valuable in the context of cranial and spine stereotactic radiosurgery in an effort to exploit the efficiency and biological potency of high–dose-per-fraction irradiation.4 This idea was soon appreciated for its clinical promise by other researchers in numerous countries across the world.
Pioneers in the field initially used customized ancillary equipment constructed in their own institutions to immobilize patients and to adapt ordinary linear accelerators for the task of precise internal tumor targeting. Now, however, the administration of high-dose, tightly focused external beam radiation therapy is greatly facilitated by a wide assortment of commercially available systems that immobilize patients, address the problem of respiratory motion during treatment, and ensure accurate treatment with the use of image guidance. The newest generation of linear accelerators from several manufacturers is either exclusively dedicated to cranial or extracranial stereotactic radiotherapy or is equipped with a built-in package of features that provide an easy means of administering this type of treatment.
Stereotactic body radiation therapy (SBRT) is the term applied in the United States by the American Society of Therapeutic Radiology and Oncology (ASTRO) for the management and delivery of image-guided high-dose radiation therapy with tumor-ablative intent within a course of treatment that does not exceed 5 fractions.5 Other descriptive terms have been occasionally applied to describe what is officially called SBRT, including the acronym SABR, an abbreviation for stereotactic ablative radiotherapy.6
BIOLOGICAL AND ONCOLOGICAL RATIONALE FOR SBRT
The appeal of SBRT is based on the nonlinear relation between radiation dose and cytotoxic effect, whereby one or a few large individual doses of radiation therapy have substantially more cell-killing effect than the same dose of radiation given in smaller individual doses. Traditionally, the expected relation between radiation dose and tumor cell kill has been commonly estimated by the well-known linear-quadratic (LQ) model of radiation dose response, often relied on for the purpose of comparing the biological potency of different schedules of conventionally fractionated radiation therapy. In the range of dose per fraction used in SBRT, however, there has been an emerging appreciation that the LQ model overestimates the potency of fraction sizes on the order of 8 to 10 Gy or higher.
A variety of alternative mathematical models have been proposed to account for the observed inaccuracy of the LQ model for doses in this range. For example, Guerrero and Li7 have proposed a modification of Curtis’s8 lethal-potentially lethal model that accounts for ongoing repair processes occurring during the time of an individual radiation treatment, thus predicting lower cytotoxicity from high doses administered over time intervals resembling typical clinical treatment times. Experimental data modeling cranial radiosurgery offer support for this concept.9 Alternatively, Park et al.10 have offered the universal survival curve formalism, which combines the LQ model for doses in the range used in conventional fractionation with a multitarget model for doses in the range used for SBRT. This piecewise function effectively achieves the same key mathematical result as other departures from the LQ model applied to SBRT, namely a more linear slope in the relation between dose and log cell kill in the high dose region.
In the special case of prostate cancer, the rationale for evaluating SBRT for prostate cancer also includes a fundamentally different consideration. Here, applying the LQ model-based assumptions and interpretation, the α/β ratio for prostate cancer has been estimated to be very low, likely in the range of 1.5 to 3.0 Gy.11,12 If this estimate of α/β ratio for prostate cancer is correct, then higher doses per fraction should provide a more favorable therapeutic ratio than a conventionally fractionated regimen. Accumulating evidence from randomized clinical trials comparing conventional daily fractions of 2 Gy with hypofractionated regimens using fractions sized on the order of 3 Gy per day offer strong support for this hypothesis and the safety of using this approach.13–15 Clinical reports involving SBRT for prostate cancer have involved doses that are higher than 3 Gy and are discussed later in the chapter.
None of the aforementioned models of high–dose-per-fraction tumor cell–killing effects explicitly incorporate a mechanism of tumor cell kill that might be of equal or greater importance than tumor DNA damage-based injury, namely the antiangiogenic effect of endothelial cell apoptosis occurring above an apparent threshold dose on the order of 8 to 10 Gy. First observed preclinically and reported by Garcia-Barros et al.,16 clinical evidence indirectly supporting the importance of this mechanism includes measures of an increase in serum markers of apoptosis post-SBRT.17 Other similar threshold effects, which do not occur until the dose per fraction exceeds a minimum “unconventional” level, have been observed, suggesting new ways in which SBRT might be further exploited.18–20
Beyond its uses as primary therapy for selected early-stage cancers, SBRT has also been used as a noninvasive and efficient means of eradicating discrete metastatic tumors, and the argument for this application can be built on numerous overlapping lines of evidence or conceptual theories of cancer growth and dissemination or cytotoxic abscopal effects: (a) the empiric or phenomenological, (b) the patterns-of-failure concept, (c) the theory of oligometastases, (d) a lethal burden variation of the Norton-Simon hypothesis, or (e) immunological enhancement.21
The most straightforward argument for SBRT in the setting of isolated sites of metastatic disease is what might be termed an empiric or phenomenological rationale. There have been numerous reports of patients enjoying a high rate of 3- to 5-year survival following various forms of aggressive local treatment (e.g., surgical resection, radiofrequency ablation, cryotherapy) for limited metastases in the liver or lung from an assortment of solid tumor types. On one level, then, SBRT is a valid, noninvasive substitute for other local modalities if it provides similar efficacy and the same or less toxicity.
The indications for SBRT in the setting of metastatic disease might be alternatively couched in terms of a patterns-of-failure model. A traditional example of a patterns-of-failure approach would be combined modality therapy for lymphomas, where systemic treatment with chemotherapy is combined with involved field radiotherapy to the sites of disease that were grossly evident at the time of diagnosis, indicating that they contain the highest number of clonogenic cells and are thus least likely to be completely eliminated by the chemotherapy. A patterns-of-failure analysis by Rusthoven et al.,22 involving patients who received chemotherapy for metastatic non–small cell lung cancer, reveals a similar result: patients are most likely to manifest tumor recurrence in sites initially involved prior to chemotherapy, opening an opportunity for SBRT to sites of residual disease following some form of systemic therapy to be given with this goal in mind. An earlier analysis by Mehta et al.23 yielded very similar observations.
Yet another argument that has been advanced in support SBRT in this setting is the theory of oligometastases. As articulated by Hellman and Weichselbaum,24 this viewpoint considers that there seems to be a subgroup of patients with metastatic disease that is intermediate between completely absent and widely metastatic. For such patients the entire systemic disease burden is then entirely contained within the finite number of individual sites of gross disease recognized by the pertinent imaging studies. This condition would reflect an intermediate point in the natural history of that individual’s cancer; therefore, these patients might be cured if their limited numbers of metastatic sites are eradicated. Among the many studies lending support to this theory would be the report by Stinauer et al.25 involving patients treated with SBRT for metastases from melanoma or renal cell carcinoma, where there was significantly longer survival for patients with oligometastatic disease, there defined as three or fewer sites, than for patients with more extensive disease.
The theory of oligometastases may be extended beyond subdividing patients according to the integer number of detectable lesions present and, instead, toward characterizing prognosis according to a continuous rather than discrete metric of disease burden. In one sense this approach is a variation of the Norton-Simon hypothesis, as applied to the whole host rather than simply tumor cell kinetics. This conjecture arose from experimental observations in animal models where the number of cancer cells within the host increases from beneath the threshold of detectability, through a phase of rapid growth, and onward toward a plateau level that is lethal to the host.26 This observation was translated into clinical trials by postulating that traditional DNA-targeted chemotherapy is expected to render the greatest degree of cytotoxicity to cancer cells in the rapid phase of growth, when there is higher mitotic activity that renders DNA more vulnerable. Trials testing dose-dense chemotherapy were designed to exploit the enhanced chemosensitivity of rapidly growing cancer cells with the use of frequent dosing schedules that capture tumors repeatedly at relatively smaller volume, without allowing time for regrowth into relatively less sensitive, near-lethal tumor volumes.27
An example of evidence in support of the concept of a clinical lethal burden of disease, as measured by a continuous rather than discrete metric, includes the observations of Lee et al.,28 who used a positron emission tomography scan-based method of quantifying the metabolically active tumor burden in patients with lung cancer and observed that it independently predicted overall survival. SBRT given to a patient with metastatic disease could, in principle, have favorable effects that align with the tenets of the Norton-Simon hypothesis. First, the SBRT might reduce the patient’s total burden of disease in such a way that the remaining cancer within the patient’s body enters into a state of relatively higher growth fraction and is thus more susceptible to cytotoxic systemic agents. Second, the SBRT could prevent or delay the condition of lethal systemic tumor burden that is fatal to the patient.29
A fifth consideration that has emerged in recent years is the possibility that high–dose-per-fraction radiation therapy influences immune system responses in a manner that can be exploited for favorable therapeutic effect. Preclinical studies have demonstrated that high–dose-per-fraction ionizing radiation can induce antigen presentation within the tumor stroma.30 Furthermore, antibody-mediated induction of T cell activity can be combined with high–dose-per-fraction ionizing radiation to enhance not only the effect on the irradiated tumor but also to create an abscopal effect whereby tumor implants remote from the irradiated site regress.31 Findings such as these suggest ideas for new investigations into the combination of SBRT and immunomodulatory agents for patients with metastatic disease.
There is overlap among all of these perspectives, and it would be impossible to prove the exclusive validity of any one above another. Improved disease-free and overall survival following the use of SBRT to metastatic sites of disease would support any of these theories. Nevertheless, it is very important to consider the larger context of cancer treatment in which SBRT is applied for metastatic disease, and these theories can serve to frame the clinical or investigational objectives when SBRT is applied as a treatment for metastatic disease, either alone or in combination with a systemic agent.
STEREOTACTIC BODY RADIATION THERAPY GUIDELINES, PHYSICS OVERVIEW, AND SAFETY CONSIDERATIONS
ASTRO and the American College of Radiology (ACR) have published guidelines that characterize the personnel qualifications and responsibilities, documentation, quality control, and clinical operations recommended for the safe and proper administration of SBRT and follow-up care for patients treated.5 The ASTRO-ACR guidelines advise that the following components should be in place within and institution’s SBRT program:
1. Qualified personnel:
a. Board-certified radiation oncologist
b. Qualified medical physicist
c. Licensed radiation therapist
d. Other support staff as indicated (dosimetrists, oncology nurses, and so forth);
2. Ongoing machine quality assurance program;
3. Documentation in accordance with the ACR Practice Guideline for Communication: Radiation Oncology;
4. Quality control of treatment accessories;
5. Quality control of planning and treatment images;
6. Quality control of treatment planning system;
7. Simulation and treatment systems that account for systematic and random errors associated with setup and target motion in a manner that is based on actual measurement of organ motion and setup uncertainty.
The American Association of Physicist in Medicine Task Group 101 (TG101) moved forward from the ASTRO-ACR guidelines to generate a report that considers additional important nuances in the planning and treatment delivery of SBRT.32 Included within the TG101 report are discussions of potential imaging artifacts and their impact on treatment planning, the challenges of small field dosimetry, and the importance of using an acceptable dose calculation algorithm, among other issues.
Proper patient repositioning, target localization, and management of breathing-related motion are essential for SBRT. A variety of patient immobilization devices are available, including several types of body frames with external fiducial markers. So-called frameless systems incorporate ultrasound, kilovolt-range imaging, or near real-time computed tomography (CT) scanning to verify the location of internal targets relative to the beams to be used. Because SBRT treatment sessions are lengthier than conventional external beam treatments, patient comfort is an important issue.
Breathing-related motion control devices and systems fall into three general categories: (a) dampening, (b) gating, and (c) tracking or “chasing.” Respiratory dampening techniques include systems of abdominal compression intended to diminish one of the largest contributors to breathing-related motion, namely diaphragmatic excursion, by obliging the inspiratory–expiratory lung motion pattern to involve more intracostal expansion and shallower breathing overall. Also included in this category are the systems employing breath-holding maneuvers to stabilize the tumor in a reproducible stage of the respiratory cycle (e.g., deep inspiration). Gating systems for SBRT, as for any radiotherapy application, follow the respiratory cycle using a surrogate indicator for respiratory motion, for example, chest wall motion, and employ an electronic beam activation trigger allowing irradiation to occur only during a specified range of expected tumor locations. Tracking or “chasing” systems move the radiation beam or patient to follow the movement of the tumor.
Regardless of the system employed, the procedure of treatment planning must include the same consideration for respiratory motion management to be used during treatment. Despite available motion control equipment, some positional uncertainty will remain. The planning target volume (PTV) margins used to account for this residual motion of the gross tumor volume (GTV) will typically range from 5 to 10 mm.
The word stereotactic has heretofore usually implied that some sort of external reference markers indexed to internal structures facilitate internal target relocalization, but the definition has expanded to include systems of image-guided radiation therapy (IGRT) that relate the position of internal targets to a three-dimensional coordinate geometry registered to the treatment machine without the use of external markers on the patient. SBRT always involves some form of IGRT to guide for treatment delivery.
Most reports describing SBRT published to date have employed high-energy photons (x-rays) as the source of therapeutic radiation, although other particles can also be used. There is no absolute standard for the combination of beam or arc angles ideal for any given clinical situation, and each case can present unique challenges. In general to achieve a tightly focused high-dose distribution within the PTV and rapid dose falloff outside the PTV, a combination of multiple (often 10 or more) noncoplanar beams or multiple arcs are required. Intensity modulation across the individual beams or arc segments can be incorporated within SBRT.
As part of ASTRO’s Target Safely campaign, the Multidisciplinary Quality Assurance Subcommittee of the Clinical Affairs and Quality Committee of ASTRO commissioned a white paper on the topic of cranial radiosurgery and SBRT titled “Quality and Safety Considerations in Stereotactic Radiosurgery and Stereotactic Body Radiation Therapy.”33 Of particular importance within the white paper are sections emphasizing the importance of creating a proactive culture of safety with procedural checkpoints and error analysis mechanisms.
CLINICAL EXPERIENCE WITH STEREOTACTIC BODY RADIATION THERAPY IN SELECTED SITES
Liver
The two major reasons for considering SBRT for hepatocellular cancer (HCC) is that underlying severe liver disease often renders patients medically inoperable and that other nonsurgical therapies have generally achieved at best rather modest success in that setting. The natural history of untreated HCC has been reported to involve a median survival in the range of 3 to 8 months,34–36 and so a safe and effective therapy is needed in this setting.
The earliest observations following SBRT for HCC were reported by a group at Karolinska Hospital,2 and more recent formal prospective studies have followed. The Princess Margaret Hospital group utilized a 6-fraction regimen in a prospective phase I study in patients with HCC (n = 31) or intrahepatic cholangiocarcinoma (n = 10).37 Prescription doses were selected according to a normal tissue complication probability (NTCP) model based on conventionally fractionated radiotherapy to the liver. The median dose to the tumor was 36 Gy (range, 24 to 54 Gy). The NTCP model overestimated the chance of radiation-induced liver disease, and it is possible that the dose could have been escalated to a higher level in most cases. Nevertheless, in this group of heavily pretreated patients (over 60% had had at least one prior therapy for HCC), a remarkable median survival of 12 months was observed.
Méndez Romero et al.38 at Erasmus University Medical Center treated eight patients with 11 separate lesions of HCC in 3 to 5 fractions to a dose of 25.0 to 37.5 Gy. A 1-year survival of 75% was observed. Choi et al.39 at the Catholic University of Korea treated 23 patients with 32 individual lesions in a 3-fraction regimen to a median dose of 36 Gy (range, 30 to 39 Gy). No patient experienced severe toxicity, although follow-up was limited (median 11 months). Interestingly, in a subsequent analysis of predictors for decline in liver function after SBRT, on multivariate analysis the only predictor for a decrease in Child-Pugh classification (CPC) from baseline level was the volume of normal liver receiving 18 Gy or more (V18). The rate of negative impact on CPC rose sharply when the V18 exceeded 800 cc.40 This latter observation favors a model of SBRT effect on normal liver in line with a critical volume model, whereby it is important to preserve a certain minimum level of function by sparing an adequate volume of normal liver from receiving a dose above a certain threshold.
The group from the Korea Institute of Radiological and Medical Sciences treated a prospectively registered cohort of 38 patients with inoperable HCC with SBRT, all of whom had failed prior transarterial chemoembolization.41 The median tumor volume was 40.5 cc (range, 11 to 464). The SBRT dose was 33 to 57 Gy in 3 or 4 fractions. Minimal grade 3 toxicity (<3%) was observed, and the 2-year overall survival was 61%.
Cardenes et al.42 from Indiana University and the University of Colorado reported a multi-institutional phase I dose escalation study of SBRT given in 3 fractions for HCC. Eligibility requirements were CPC-A or -B, medical or technical inoperability, and three or fewer lesions of cumulative tumor diameter 6 cm or less. The study enrolled 17 patients with 25 individual lesions. Dose was escalated from 36 to 48 Gy (16 Gy per fraction) in CPC-A patients without dose-limiting toxicity; however, two patients with CPC-B status at baseline developed grade 3 hepatic toxicity at the 42 Gy (14 Gy per fraction) dose level. Consequently, the dose for CPC-B patients was reduced to 40 Gy in 5 fractions. There were no local failures within the treated volume, and six patients proceeded to liver transplantation. The 2-year overall survival for the entire group was 60%.
The Indiana University group has separately reported their single institution experience involving a total of 60 patients: 34 CPC-A, 25 CPC-B, and 1 CPC-C.43 The median number of fractions, dose per fraction, and total dose, was 3, 14 Gy, and 44 Gy, respectively, for those with CPC-A cirrhosis and 5, 8 Gy, and 40 Gy, respectively, for those with CPC-B. With a median follow-up time of 27 months, the 2-year local control rate was 90%. Two-year overall survival was 67%. SBRT served as a bridge to liver transplant for 23 patients who underwent transplant at a median time of 7 months following SBRT. A progression in CPC was observed in 20% of patients within 3 months of treatment.
Outcomes following SBRT for liver metastases have also been reported by numerous groups44–51 and are summarized in Table 17.1. Regimens of 1 to 5 fractions have been employed, and total doses up to 60 Gy to the planning target volume have been administered. Taken together, the results show good survival outcomes achieved in heavily pretreated individuals and a trend toward improved local control with increasing dose.
Chang et al.52 reported a pooled analysis from three institutions with the use of liver SBRT for liver metastases from colorectal primary cancers. The combined experience from Stanford, Princess Margaret Hospital, and the University of Colorado included 65 patients with a total of 102 individual liver metastases from colorectal cancer treated. More than half of the patients had had at least one prior systemic therapy regimen, and over 40% of the patients had had two or more prior systemic regimens. The analysis indicated that to achieve durable local control of treated lesion, a 3-fraction total dose on the order of 48 Gy is needed. Sustained local control after SBRT was closely associated with improved survival on multivariate analysis (P = .06).
Technical issues and posttreatment imaging follow-up considerations unique to liver SBRT have been included in these reports and in review papers.53,54 Briefly, target delineation and image guidance can be difficult, because liver metastases are not well visualized on CT scans or in-room volumetric imaging used for IGRT. In many centers, radiopaque fiducial markers are place in or near the metastases to facilitate IGRT. In all centers, the planning target volume is an expansion of the gross tumor volume in consideration of the setup and intrafraction motion to be expected with the particular setup and delivery system employed. Typically the margin used to expand a GTV into a PTV is on the order of 5 mm axially and 5 to 10 mm craniocaudally.
The Colorado group first applied the critical volume approach to normal liver dose constraints in their initial phase I study55 and subsequent phase II study. Noted above in relation to the observations of the Catholic University of Korea in their treatment of primary liver tumors, the critical volume model liver SBRT is an adaptation of the early work of Yeas and Kalend.56 Applicable for organs of radiobiologically parallel structure, the crux of this application is to work backward, in a sense, from an estimate of how much volume of the organ is essential and must be protected from functional ablation. The estimate for liver that at least 700 cm3 should receive less than 15 Gy during a 3-fraction SBRT course was derived from a combination of prior reports of outcomes after partial hepatectomy documenting approximate minimum volumes required and estimates of the effects of that dose of radiation extrapolated from prior reports of conventionally fractionated treatment.
One feature of the normal tissue effect of liver SBRT consistently observed within the first few months after SBRT is a zone of hypodensity observed on follow-up CT scans corresponding to the volume that received approximately 30 Gy.55 This phenomenon, first described by Herfarth et al.57 following single-dose liver SBRT, is likely related to local veno-occlusive effects.58 There is no known clinical consequence associated with the finding, but it can cloud the assessment of tumor response within the first few months after liver SBRT.
TABLE 17.1 STEREOTACTIC BODY RADIATION THERAPY FOR LIVER METASTASES
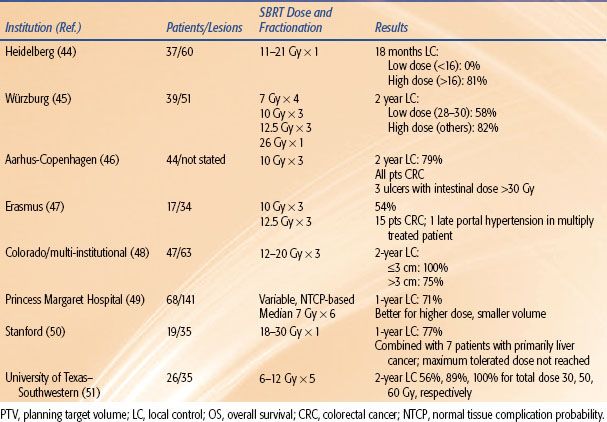
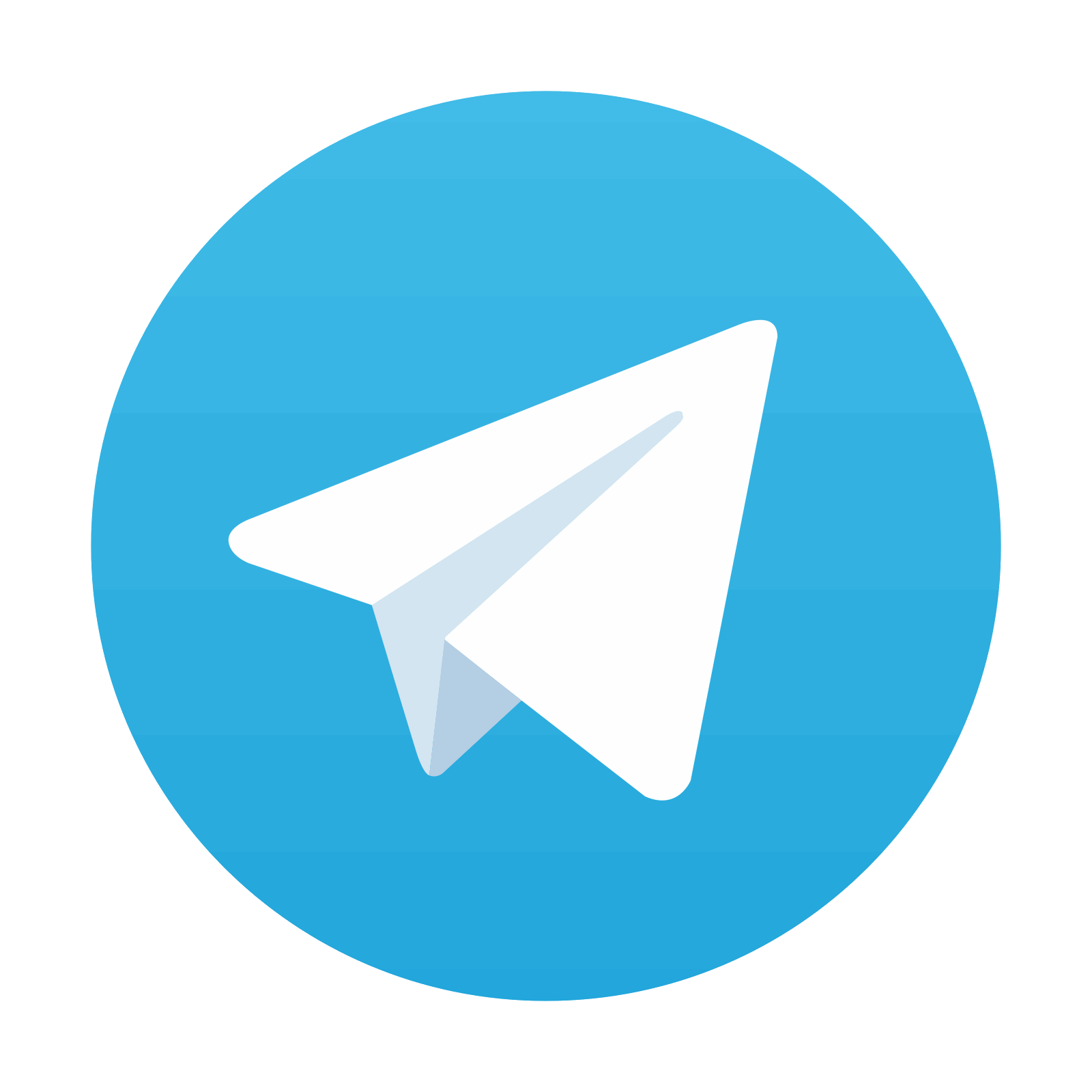