Authors
Years
Locations
Study methods
N
(F, M)
Age at enroll (years)
Fracture sites
RR [OR]a
Associations, impact of comorbidities
CGC
Zhukouskaya [51]
2013
Belarus
Case–control
82/82
F + M
20–55
Vertebral
4
NRb
No
Neumann [30]
2011
Germany
Case–control
128/77
F + M
20–70
Any
[2.6] (F)
Not associated with microvascular complications
Yes
[1.9] (M)
Danielson [32]
2009
USA (WI)
Case–control
75/75
F
18–50
Any
[2.3]
Poor control associated with low BMD
NR
Vestergaard [22]
2007
Mixed
Meta-analysis
5 studies
F + M
–
Hip
6.9
–
NR
Janghorbani [175]
2007
Mixed
Meta-analysis
6 studies
F + M
–
Hip
6.3
–
NR
Ahmed [55]
2006
Norway
Prospective cohort—6 years
27,159 (T1D = 81)
F + M
25–98
All non-vert. hip
3.1 (M) 8.9 (F) 17.8 (M)
–
NR
Strotmeyer [40]
2006
USA (PA)
Case–control
67/237
F
35–55
Any
[1.9]
–
NR
Janghorbani [176]
2006
USA
Nurses’ health study cohort
101,343 (T1D = 292)
F
30–55
Hip
6.4
–
NR
Vestergaard [54]
2005
Denmark
Case–control
1745/2618
F + M
43 ± 27
Hip
[1.7]
Hypoglycemia
NR
Spine
[2.5]
Miao [58]
2005
Sweden
Prospective cohort—10 years
24,605 (all T1D)
F + M
20.7 ± 10.9
Hip
9.8 (F) 7.6 (M)
Microvascular, Cardiovascular complications
NR
Nicodemus [177]
2001
USA (Iowa)
Prospective cohort—11 years
32,089 (T1D = 47)
F
55–69
Hip
12.25
–
NR
Forsen [178]
1999
Norway
Prospective cohort—9 years
35,444 (T1D = 54)
F + M
≥50
Hip
6.9 (F)
Impaired vision, stroke, motor deficits
No
Though only a very few studies have examined fracture risk at other skeletal sites [51, 54], an increased risk for vertebral fracture is also a consistent finding in studies that have quantified this. This includes the elevated prevalence of asymptomatic vertebral fractures in T1D, independent of BMD [51]. And, in one study, an approximate threefold increase in risk for all non-vertebral fractures was reported in men with T1D [55]. Even in case–control studies comparing lifetime fracture history at any site, an increased frequency of fracture is noted in persons with T1D (odds ratios of ~2) [30, 32]. And, as in other conditions, a more common site for osteoporotic fracture in adults with T1D remains limb fractures, related to accident or falls [44, 47, 56].
In diabetes, an increased risk for fracture has been associated with longer duration of disease [44]; then again, few studies of fracture risk in pediatric patients with T1D have been conducted. However, from one study with relatively small sample size, when comparing the history of any fracture in pediatric T1D patients ≤13 years of age to age-matched control subjects, no significant increase in fracture occurrence was yet seen in these early years [57].
Whether fracture risk is influenced by long-term glycemic control remains unclear, as studies exist to both support and refute this idea (Table 1.1). Nevertheless, in T1D, the presence of diabetic microvascular complications [58], and specifically including retinopathy [44], and nephropathy [59], are associated with a higher risk for fracture, though this increased fracture risk might relate, in part, to the health consequences of these comorbidities (decreased visual acuity or kidney function). Certainly, in persons with long-standing T1D, fracture risk is amplified by an increased fall risk, as might result from hypoglycemic events, visual deficits, peripheral neuropathy, or physical disability [60].
Recognizing that diabetic bone has a greater propensity for fracture than is predicted by BMD, attributes of diabetic bone quality have been examined. A role for the skeletal accumulation of advanced glycation end products (AGEs; see section “Advanced Glycosylation End Products,” below) [61, 62], chronic hyperglycemia [30], oxidative stress [63], and microarchitectural bone defects [64] have all been proposed, and it is expected that the pathological mechanisms leading to bone fragility in T1D are multifactorial [65]. To date, however, most information concerning diabetic bone quality is provided by data from animal models. This topic is reviewed in greater detail in other chapters (see Chaps. 9 and 10).
Beyond fragility fractures, other skeletal complications also occur disproportionately in persons with T1D, including fracture-healing complications (nonunion, malunion) [66], Charcot osteoarthropathy [67], osteomyelitis, and diabetic foot syndrome. These orthopedic complications are discussed in Chap. 4.
Onset of Skeletal Pathology: Studies in Children and Adolescents
When focused on pediatric patients with T1D, measurements of bone mineral density by dual X-ray absorptiometry (DXA) alone have provided conflicting results [20], with specific reports demonstrating global reductions in BMD [68], site-specific reductions in BMD [69, 70], or minimal to no effect on bone density [71, 72]. By providing only a two-dimensional estimate of BMD, however, DXA neglects parameters of bone size, bone geometry and bone compartment, which are critical components of the integrity of the growing skeleton. Other investigations using QUS or peripheral quantitative computed tomography (pQCT) provide additional information on bone mineral content, bone size, and bone structure. Collectively, these studies do suggest that cumulative changes in bone architecture are beginning early in childhood, particularly in those diagnosed with T1D at very young ages [73]. Compared with nondiabetic children, reductions in BMD [68, 74–78] and bone size, specifically total cross-sectional area (CSA) [73, 79] and cortical area [15, 80], are relatively consistent findings. Consequently, total bone mineral content of these generally smaller bones is also reduced in pediatric patients with T1D [80].
A mild delay in skeletal maturation, acquired after diagnosis, has been reported in some studies [81, 82]. In addition, the age of peak bone mass acquisition may be delayed in individuals with T1D [68, 72] and chronically poor glycemic control may negatively impact growth velocity [83]. However, unlike historical reports of short stature (i.e., Mauriac syndrome) in poorly controlled T1D [84], in the modern era of tighter glycemic management the impact of T1D on final height is minimal [73, 82, 85].
As with adults, the impact of T1D on the pediatric skeleton may again be gender-discrepant. Several studies report a more significant impact in young males [80]. Additionally, in a study of females, 13–19 years of age, no differences in BMD, measured by DXA, were seen when comparing T1D with control subjects [72]. Other comorbidities, including the diagnosis of associated autoimmune disorders during childhood [86], may also exacerbate the impact of DBD on the growing skeleton (see section “Effects of Ancillary Diagnoses: Celiac Disease, Autoimmune Thyroid Disease, Addison’s Disease”). Possible pediatric risk factors for DBD are listed in Table 1.2. Evidence to date would suggest that in patients with one or more of these risk factors, earlier screening for DBD may be warranted.
Table 1.2
Pediatric risk factors for diabetic bone disease
Possible pediatric risk factors for DBD in adulthood | References |
---|---|
Younger age at diagnosis | [73] |
Longer duration of disease | |
Longer duration of poor glycemic control | |
Lower BMI | |
Delayed skeletal maturation | [81] |
Male gender | [80] |
Accompanying celiac disease | |
Vitamin D deficiency |
Contributing Pathological Mechanisms
Decreased Bone Formation
Analysis of bone turnover markers in persons with T1D suggest that bone homeostasis is altered so as to create, predominantly, a state of lowered bone formation, osteoblast dysfunction, and low bone turnover. Numerous studies have demonstrated a decrease in serum osteocalcin (OC) concentration, a marker of bone formation, in children and adolescents [87–90], young adults [31], and middle-aged adults [32, 90–92] with T1D. Even at the time of diagnosis, OC levels in children with T1D appear to be lower than in healthy children [93]. Additionally, lower OC concentrations, typically, are associated with indices of poorer glycemic control [32, 88, 91, 94–96] implying a disease-specific effect. A decrease in serum concentrations of insulin-like growth factor-I (IGF-I), an anabolic regulator of osteoblast function, is also very common in patients of all ages with T1D [31, 97–99], and IGF-I levels correlate with residual beta-cell function, again implying a potential impact of disease severity on bone health in T1D [100]. Similarly, a decrease in serum levels of bone-specific alkaline phosphatase (bALP), a marker of active bone formation and osteoblast activity, has been reported in adults with T1D [84]. However, somewhat contrary to expectations for a state of low bone turnover, an increase in bALP has been reported by others [91, 98] although this has been postulated to reflect impaired osteoblast differentiation. Finally, serum levels of sclerostin, an osteocyte product that antagonizes the Wnt signaling pathway and hence inhibits bone formation, are increased in T1D [101] perhaps more-so in women [102]. As a whole, these studies suggest that systemic markers of bone formation in T1D are generally indicative of a condition in which bone formation is reduced.
In T1D, an uncoupling of bone formation and bone resorption is also apparent. In young [31] and middle-aged [32] women and in men [103] markers of bone resorption, including urine N-terminal telopeptides (NTx), are comparable in concentration between T1D cases and controls. Similarly, urine deoxypyridinoline (DPD) [91, 104] and C-terminal telopeptide (CTX) [21, 104] values are comparable between patients and healthy controls. Osteoprotegerin (OPG), an inhibitor of osteoclast formation, and subsequently of bone resorption, has also been assessed in T1D. Plasma OPG concentrations and OPG mRNA expression [88] are typically, but not unequivocally [105], higher in persons with T1D. This has been demonstrated both in pediatric [57, 88] and in adult populations [21]. Whether this finding suggests a state of constrained bone resorption, however, is unclear since OPG levels are also increased in periodontal disease [106] and can be indicative of vascular pathology or endothelial dysfunction. Finally, average serum PTH concentrations in T1D are often elevated relative to a matched control population, but frequently not above normal ranges in patients without renal dysfunction or vitamin D deficiency [25, 107]. Taken together, it would appear that T1D is characterized best as a state of inappropriately lowered bone turnover which exists in conjunction with relative osteoblast dysfunction [90] and, hence, low bone formation [103].
Vitamin D Deficiency
Hypovitaminosis D is an important risk factor for decreased bone mineralization [108, 109]. Hence, studies have examined the prevalence of vitamin D deficiency or insufficiency among individuals with T1D, both in childhood and adulthood, and across a variety of geographic locations; many suggest that vitamin D insufficiency/deficiency is more common in T1D compared with the general population [110–112]. Additionally, circulating levels of 25OHD are very often found to be significantly lower in case–control comparisons of T1D with the general population [107, 112–114], even when absolute levels do not meet diagnostic cut-points for deficiency. Even so, a 13-year Danish study of 25OHD levels in 907 children with newly diagnosed T1D, compared with 896 nondiabetic siblings, a study intended to eliminate genetic and environmental confounding, showed no difference in vitamin D levels [95]. Hence, the nature of this relationship between vitamin D deficiency and T1D, as being a preexisting condition or an acquired condition, remains controversial.
Several mechanisms could contribute to vitamin D deficiency in T1D. By cross-sectional analysis, data from National Health and Nutrition Examination Surveys (NHANES III and NHANES 2001–2006) identified an association between vitamin D deficiency and/or insufficiency and albuminuria [115, 116]. In addition, excess urinary loss of vitamin D binding protein (DBP) in T1D, particularly in persons with albuminuria, might contribute mechanistically to vitamin D deficiency. Consistent with this hypothesis, we have demonstrated a significant increase in the urinary excretion of DBP in persons with T1D compared with controls; additionally, urine DBP concentrations correlated with urinary albumin excretion, and vitamin D deficiency or insufficiency was again more prevalent in diabetic subjects with albuminuria [107]. Decreased serum DBP levels in T1D have also been reported [117]. Finally, population-specific genetic variation in the vitamin D axis has been proposed. Vitamin D receptor (VDR) gene polymorphisms (FokI), for instance, have been associated with vitamin D deficiency [118]. However, while polymorphisms in the VDR gene (e.g., FokI, BsmI, ApaI, TaqI) have inconsistently been linked to possible susceptibility for T1D in certain populations, VDR genotypes have not been associated with differences in bone turnover markers seen in T1D [119, 120] or with measurements or bone mineral density [120, 121].
While numerous studies have examined the prevalence of vitamin D insufficiency/deficiency in various T1D populations, clinical data examining a direct role for vitamin D in the pathogenesis of diabetic bone disease are scarce, and largely historical. In 1981, a study of 45 Caucasian children with T1D (age 7–18 years), reported no difference in circulating 25OHD levels between non-osteopenic vs. osteopenic diabetic patients (cortical thickness >2SD below the mean normal value) [122], though much less stringent glucometabolic control would have been standard-of-care at the time. However, a more recent study of 58 children with T1D, ages 9–19 years, found that children with vitamin D deficiency and T1D did have lower lumbar spine BMD Z-score [89]. Additionally, serum 25OHD levels have been shown to negatively correlate with serum collagen type 1 C-terminal propeptide [123], yet positively correlate with GLA-carboxylated osteocalcin [124], perhaps inferring a positive effect of 25OHD on bone quality in T1D. Finally, some studies suggest that vitamin D insufficiency may also increase the risk for insulin resistance in T1D [111, 125], hence perhaps impeding the anabolic benefits of insulin signaling in bone [20] in these patients.
Advanced Glycosylation End Products
In the presence of hyperglycemia, the non-enzymatic addition of reduced sugar moieties to amine groups on both tissue-specific and circulating proteins occurs. The generation of one subtype, the advanced glycosylation end products (AGEs), in the milieu of chronic hyperglycemia and the interaction of AGEs with their receptors (RAGEs) are thought to play a significant role in the pathogenesis of diabetic complications [126] including diabetic cardiovascular disease [127], diabetic nephropathy [128], and diabetic retinopathy [129], via activation of pro-inflammatory pathways. While enzymatic cross-link formation between bone collagen molecules is an important element of the mechanical strength and material properties of healthy bone, the dysregulated accumulation of AGEs in bone is thought to negatively impact the integrity of skeletal tissues [130] possibly by causing micro-damage, hindering osteoblast function and differentiation [131], reducing bone turnover, and/or by competitively inhibiting normal enzymatic cross-link formation [132]. For example, evidence exists to suggest that AGE accumulation is operative in age-related bone fragility [93].
Because diabetic bone has a greater propensity for fracture than is predicted by BMD deficits alone, reduced bone quality secondary to AGE accumulation [133] has also been hypothesized. However, in T1D this mechanism has not yet been established. Measurements of urine and serum pentosidine have been used as biomarkers for AGE accumulation in bone, particularly trabecular bone [134]. Adults with diabetes have increased levels of tissue AGEs compared with chronologically age-matched nondiabetics [96]; and, in older adults with type 2 diabetes, higher urine [135], and serum pentosidine [136] levels correlate with increased vertebral fracture prevalence. Similar data linking serum pentosidine levels and fracture risk in T1D have only recently been reported [137]. Nevertheless, serum AGE concentrations are clearly elevated in T1D during childhood [138], even during preschool and prepubertal years [139]. In addition, skin AGEs, estimated from measurements of skin intrinsic florescence (SIF), are increased in children with both T1D and T2D, to the extent that “approximately 4–6 years of diabetes exposure in some children may be sufficient to increase skin AGEs to levels that would naturally accumulate only after ~25 years of chronological aging” [140]. A similar elevation in SIF is observed in adults with T1D, and is clearly related to long-term glycemic control [141–143]. Gingival AGEs are also increased in T1D-associated periodontitis [144]. Even so, while studies to date suggest an association of AGEs with micro- and macrovascular complications of diabetes, future research will be required to establish a link between bone AGE biomarkers and skeletal phenotype in T1D-associated diabetic bone disease.
Effects of Ancillary Diagnoses: Celiac Disease, Autoimmune Thyroid Disease, Addison’s Disease
The relationships between autoimmune disease and bone loss are multifactorial, with outcomes dictated by: (1) direct immune cell regulation of bone homeostasis; (2) disease-specific systemic or local inflammation (e.g., rheumatoid arthritis); (3) disease-specific systemic hormone alterations (e.g., hypothyroidism/hyperthyroidism); (4) ancillary organ damage; or (5) negative skeletal consequences of therapy (i.e., corticosteroids) (For Review, [145]). Certain autoimmune disorders in particular, including autoimmune thyroid disease (ATD), celiac disease (CD), and Addison’s disease (AD) are more prevalent in persons with T1D, and all can independently confer detrimental effects on skeletal homeostasis, compounding the impact of diabetic bone disease. The prevalence of celiac autoimmunity or CD in persons with T1D has been estimated at between 4 and 12 % [86, 146–149] and osteoporosis, along with an increase in the risk of bone fractures, is present in the majority of celiac patients [150]. It is interesting, therefore, that in children with T1D, high-titer seropositivity to celiac antigens in otherwise asymptomatic children is associated with lower bone mineralization [151]. Similarly, in studies of BMD in adults with T1D, a greater reduction in BMD, as well as a higher incidence of fractures [149], is seen among the subset of T1D patients with concurrent celiac autoimmunity [149, 152, 153]. In patients with T1D and active CD, a significant increase in the prevalence of osteopenia (compared to T1D alone) is seen in those with seropositivity and non-adherence to dietary gluten restrictions [154]. Finally, autoantibodies against osteoprotegerin have been reported in a few patients with celiac disease, possibly further contributing to osteoporosis [155]. Hence, in persons with a long-standing coexistence of T1D and CD, the risk for poor bone health is almost undoubtedly higher.
Autoimmune thyroid disease (ATD), either Hashimoto’s disease or Grave’s disease can occur in 12–30 % of persons with T1D [86, 147], likely related to a common genetic susceptibility for the two disorders [156]. Whether related to the primary hormonal disruption, or to the antithyroid autoimmunity [157], these thyroid disorders can independently contribute to a decrement in BMD. Prevalence rates for adrenal autoantibodies and/or Addison’s disease (AD) among persons with T1D vary from 0.5 to 1.4 % [86, 147, 158]. While Addison’s disease is a rare condition, AD is also associated with a higher prevalence of osteopenia or osteoporosis [159] and an increased risk of fracture [160], which is unrelated to glucocorticoid replacement therapy. At present, it is not clear whether the diagnosis of ATD or of AD in a person with T1D further increases the risk of osteoporosis or fracture; however, the combination of multiple autoimmune diseases in some persons with T1D may synergistically increase the risk of low bone mineral density [29].
Treatment of Diabetic Bone Disease in T1D
Because diabetic bone disease in type 1 diabetes represents a deficit in osteoblast function and bone formation, antiresorptive therapies for osteoporosis (e.g., bisphosphonates, denosumab) may be ineffective in this form of secondary osteoporosis, other than as combination therapy in conditions of increased bone resorption (i.e., postmenopausal females with T1D) [161]. Calcium and vitamin D supplementation, where indicated, is considered standard-of-care for osteoporosis treatment [162]. Nonetheless, 1 year of calcitriol supplementation in young adults with recent-onset T1D did not significantly change circulating markers of bone turnover, specifically levels of OC or beta-Crosslaps [160]. Moreover, very little information from comparative effectiveness studies is available on the treatment of osteoporosis in T1D. In animal models, intermittent PTH treatment has been shown to improve osteoblast survival and reverse diabetes-associated bone loss [163]. In clinical trials examining the effectiveness of injectable PTH (teriparatide) for treatment of osteoporosis, however, diabetes has typically been an exclusion criteria preventing enrollment, though positive results with off-label use of teriparatide for nonunion fracture repair [164] or Charcot osteoarthropathy provide some early support for this concept. Clearly, additional clinical investigation in this area is needed.
Prevention of Diabetic Bone Disease in T1D
Greater than 90 % of peak adult bone mass is typically achieved by the end of the second decade of life, and ~40 % of this bone mass is acquired during the adolescent growth spurt; hence, adolescence is a period of particular importance for prevention strategies. Optimal T1D disease management in children and adolescents, according to best practice guidelines [3], would seem a prudent approach to minimizing the impact of T1D on skeletal tissues. This would include effectively managing blood glucose to achieve age-appropriate treatment goals for plasma glucose and HbA1c, as published [3]; additionally, adequate insulin replacement may, in and of itself, have beneficial anabolic effects on bone [20]. Routine screening for associated autoimmune disorders, such as celiac disease and hypothyroidism, as is recommended, is also a necessary component to prevention [3].
Optimal bone mineralization during puberty should also become an important objective for the pediatric clinician, utilizing the following general guidelines:
Weight-bearing exercise during childhood and adolescence is an important determinant of bone mineral density [165, 166]. Regular physical exercise and sustained physical activity should be encouraged for all adolescents, in accordance with physical activity recommendations of the Centers for Disease Control [167], and as further delineated for persons with T1D by the American Diabetes Association [3]; specifically, it is recommended that children and adolescents perform 60 min or more of physical activity daily. Demonstrating the importance of exercise, a 9-month weight bearing physical activity program comparing 27 children with T1D and 32 healthy children demonstrated improved bone mineral accretion in T1D children, comparable in magnitude to the improvements seen in healthy children [160].
Adequate daily intake of calcium is important. During puberty, normal skeletal mineralization is dependent upon calcium accrual; calcium acquisition/retention rates vary by gender and race, but are estimated at ~160–500 mg/day [168–171]. To achieve this, the most recent Institute of Medicine dietary guidelines for the Recommended Dietary Allowance for calcium for adolescents is 1300 mg per day [172]. Recognizing that urinary calcium excretion is increased as a consequence of osmotic diuresis in T1D, recommendations for daily calcium intake in the adolescent with T1D may, in fact, be greater.
Recommended Dietary Allowance (RDA) for vitamin D in children and adolescents 1–18 years of age is 600 IU daily [172]. Because vitamin D insufficiency is more common in persons with T1D, the periodic assessment of vitamin D status along with the diagnosis and treatment of vitamin D deficiency in this population are also important goals.
Consensus Recommendations
The FRAX® tool, or World Health Organization Fracture Risk Assessment tool, was developed to integrate BMD measurements and patient-specific clinical risk factors in determining an individual’s 10-year probability of fracture. Current American Diabetes Association (ADA) Standards of Medical Care for fracture assessment are largely derived from studies of fracture risk in type 2 diabetes, which suggest that for a given FRAX® score, the risk of fracture is higher in persons with diabetes [3, 173]. ADA guidelines state that “it is appropriate to assess fracture history and risk factors in older patients with diabetes and recommend BMD testing if appropriate for the patient’s age and sex” [3]. Additionally, the fracture risk prediction algorithm used in the United Kingdom (QFracture algorithm) has been recently updated to incorporate type 1 diabetes as a specific variable in their hazard assessment [174]. Guidelines for BMD testing in children and adolescents with T1D, however, do not currently exist.
Summary
Persons with type 1 diabetes, particularly those with long-standing disease, are at significantly increased risk for osteopenia or osteoporosis, fractures, and poor bone healing, collectively now termed diabetic bone disease. Many factors contribute to these deficits in skeletal integrity, including: (1) a disease-specific reduction in new bone formation; (2) an increased occurrence of vitamin D insufficiency; (3) a variety of bone-adverse comorbid conditions, including diabetic microvascular complications and associated autoimmune diseases; and, very possibly (4) glucose-related alterations in the material properties and mechanical strength of diabetic bone. Because T1D is most commonly diagnosed in childhood and adolescence, the early assessment of bone health and skeletal risk factors in youth with T1D will be important, in an effort to maximize the acquisition of peak bone mass in these patients, and to minimize the impact of T1D on the skeleton going forward.
References
1.
Egro FM. Why is type 1 diabetes increasing? J Mol Endocrinol. 2013;51(1):R1–13. doi:10.1530/JME-13-0067.PubMed
2.
Atkinson MA, Eisenbarth GS, Michels AW. Type 1 diabetes. Lancet. 2014;383(9911):69–82. doi:10.1016/S0140-6736(13)60591-7.PubMedCentralPubMed
3.
American DA. Standards of medical care in diabetes—2014. Diabetes Care. 2014;37 Suppl 1:S14–80. doi:10.2337/dc14-S014.
4.
Pettitt DJ, Talton J, Dabelea D, Divers J, Imperatore G, Lawrence JM, et al. Prevalence of diabetes in U.S. Youth in 2009: The SEARCH for diabetes in youth study. Diabetes Care. 2014;37(2):402–8. doi:10.2337/dc13-1838.PubMedCentralPubMed
5.
Kim HS, Shin JA, Chang JS, Cho JH, Son HY, Yoon KH. Continuous glucose monitoring: current clinical use. Diabetes Metab Res Rev. 2012;28 Suppl 2:73–8. doi:10.1002/dmrr.2346. Epub 2013/01/04.PubMed
6.
Shalitin S, Peter CH. Diabetes technology and treatments in the paediatric age group. Int J Clin Pract Suppl. 2011;170:76–82. doi:10.1111/j.1742-1241.2010.02582.x. Epub 2011/11/10.PubMed
7.
Ludvigsson J. Novel therapies in the management of type I diabetes mellitus. Panminerva Med. 2012;54(4):257–70. Epub 2012/11/06.PubMed
8.
Nathan DM, Bayless M, Cleary P, Genuth S, Gubitosi-Klug R, Lachin JM, et al. Diabetes control and complications trial/epidemiology of diabetes interventions and complications study at 30 years: advances and contributions. Diabetes. 2013;62(12):3976–86. doi:10.2337/db13-1093.PubMedCentralPubMed
9.
Golden SH, Sapir T. Methods for insulin delivery and glucose monitoring in diabetes: summary of a comparative effectiveness review. J Manag Care Pharm. 2012;18(6 Suppl):S1–17. Epub 2012/09/26.PubMed
10.
Mathiassen B, Nielsen S, Johansen JS, Hartwell D, Ditzel J, Rodbro P, et al. Long-term bone loss in insulin-dependent diabetic patients with microvascular complications. J Diabet Complications. 1990;4(4):145–9.PubMed
11.
Hui SL, Epstein S, Johnston Jr CC. A prospective study of bone mass in patients with type I diabetes. J Clin Endocrinol Metab. 1985;60(1):74–80. doi:10.1210/jcem-60-1-74.PubMed
13.
McNair P, Christiansen C, Christensen MS, Madsbad S, Faber OK, Binder C, et al. Development of bone mineral loss in insulin-treated diabetes: a 1 1/2 years follow-up study in sixty patients. Eur J Clin Invest. 1981;11(1):55–9.PubMed
14.
Miazgowski T, Czekalski S. A 2-year follow-up study on bone mineral density and markers of bone turnover in patients with long-standing insulin-dependent diabetes mellitus. Osteoporosis Int. 1998;8(5):399–403.
15.
Santiago JV, McAlister WH, Ratzan SK, Bussman Y, Haymond MW, Shackelford G, et al. Decreased cortical thickness & osteopenia in children with diabetes mellitus. J Clin Endocrinol Metab. 1977;45(4):845–8. doi:10.1210/jcem-45-4-845.PubMed
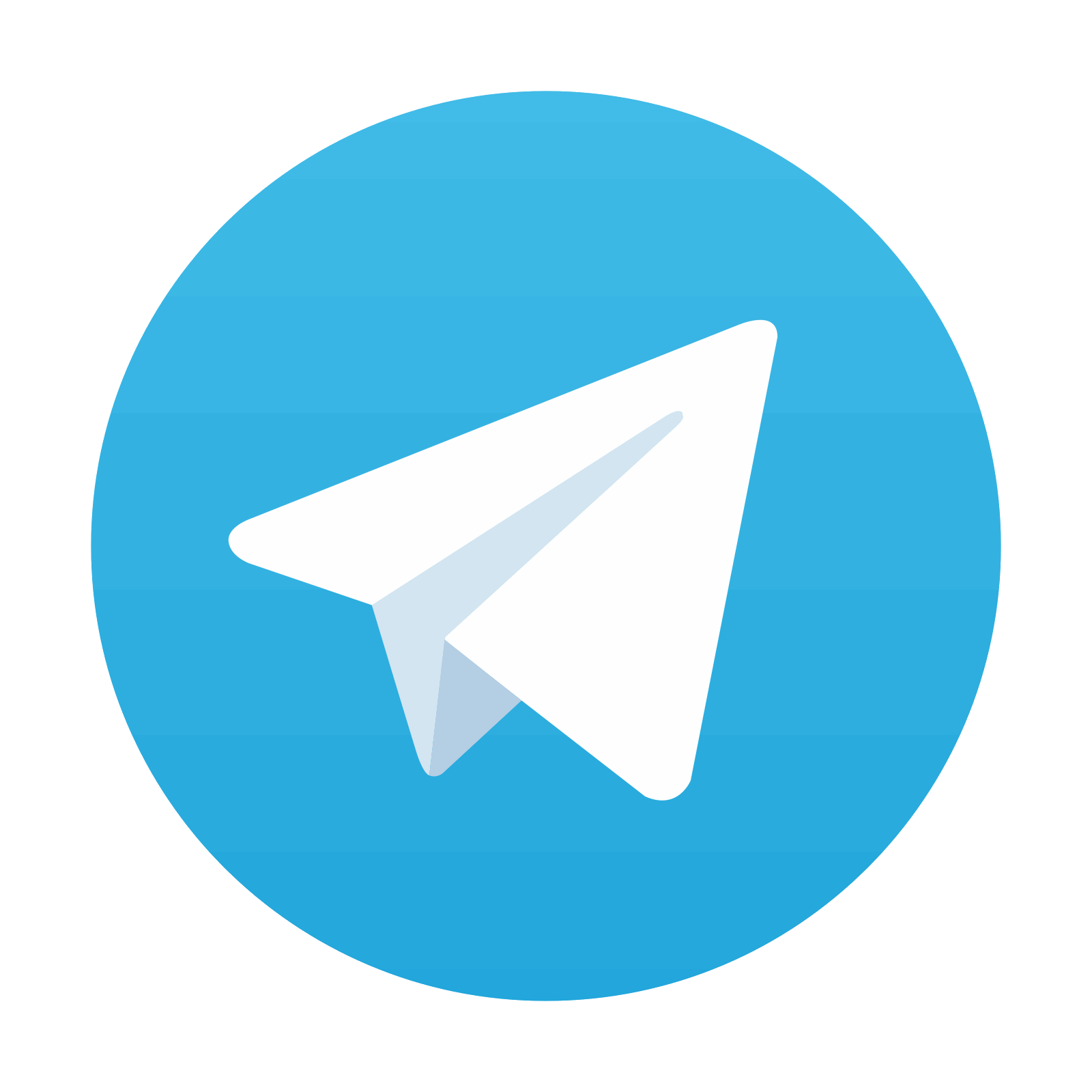
Stay updated, free articles. Join our Telegram channel

Full access? Get Clinical Tree
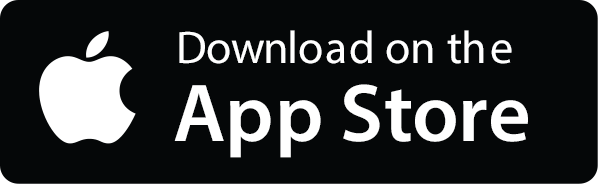
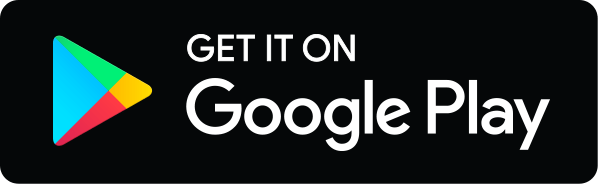