Retroperitoneal Cancer
Primary tumors of the retroperitoneum present in unselected series with a frequency of 0.1% to 0.2%.1–3 They are notable for their widely disparate histologies and presentations. Morgagni published the first report of a retroperitoneal lesion in 1761 after an autopsy.4 However, the most comprehensive work on retroperitoneal lesions was done in 1954 by Pack and Tabah.3 They added 120 cases from the Memorial Cancer Center experience to a survey of the world’s literature, which contained 750 prior reports. Recent series are histology specific (e.g., sarcomas). Older series, based on clinical presentations, are composed of a variety of tumor types and because of this continue to provide the most valuable data on presenting signs and symptoms. However, because of the tremendous variations in histologies, widely disparate therapeutic recommendations came about. Radiographic interpretation of these lesions has been aided by more recent data and ever improving technology. The focus on this chapter is on lesions that are primary to the retroperitoneum rather than metastatic to it.
FIGURE 75.1. Sagittal view of trunk showing the retroperitoneal space (shaded area). The kidney is outlined by dots. (From Wasserman TH, Tepper JE. Retroperitoneum. In: Perez CA, Brady LW, eds. Principles and practice of radiation oncology, 3rd ed. Philadelphia, PA: Lippincott-Raven, 1997:1943–1956.)
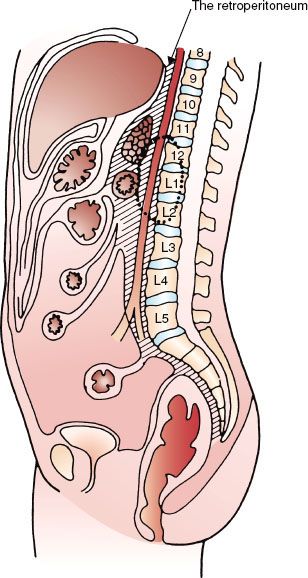
ANATOMY
The retroperitoneal space is the potential space posterior to the peritoneum or abdominopelvic cavity (Fig. 75.1). The superior border of this space is the diaphragm, and its inferior border is the superior aspect of the pelvic diaphragm (the levator ani and the coccygeus muscles). In terms of vertebral levels, the retroperitoneum stretches from the 12th thoracic vertebral body to the distal coccyx. Bilaterally, the border was classically considered to be at the lateral edge of the quadratus lumborum. Pack and Tabah3 consider the lateral extent of the 12th rib to be more valuable because this is the origin of the transversus abdominis aponeurosis as well as the mid-iliac crest. The muscles of the posterior abdominal wall, the psoas and quadratus lumborum muscles, form the posterior border of the retroperitoneum. The renal fascia forms a cone that helps to protect the pelvis from perirenal disease.5,6
Most of the tissue in this space consists of lymphatics and loose connective tissue. Retroperitoneal organs, either partially or fully contained, include urinary organs (adrenals, kidneys, ureters, and bladder), vascular organs (aorta and inferior vena cava), alimentary canal organs (esophagus and the upper two-thirds of the rectum), and many nerves. Organs that moved from within the peritoneum to outside of it during organogenesis include parts of the pancreas (head, neck, and body), the nonproximal duodenum, and the ascending and descending portions of the colon. The tail of the pancreas is within the splenorenal ligament and is thus intraperitoneal.
Nethercliffe et al.7 described the surgical anatomy of the retroperitoneum in their review of retroperitoneal surgical techniques. The most common surgical approaches described include the following: (a) subcostal (below the 12th rib), (b) supracostal (above the 12th rib), (c) transcostal (removing the 11th or 12th rib), and (d) thoracoabdominal.7
Laparoscopic and other minimally invasive techniques that cause less postoperative morbidity often defer these approaches.
FIGURE 75.2. Age at diagnosis of malignant retroperitoneal tumors (pooled data from multiple series).
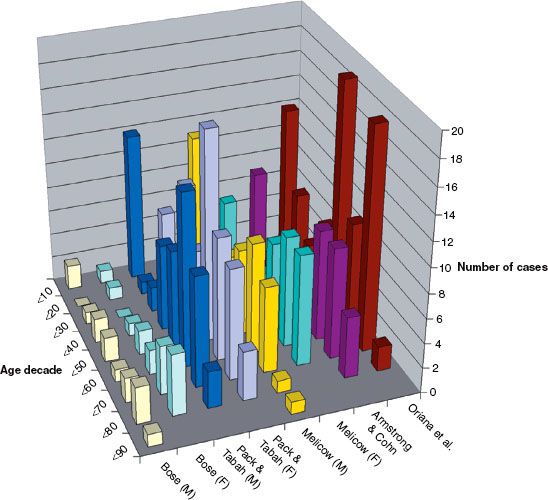
FIGURE 75.3. Percentage of retroperitoneal lesions by histology (pooled data from multiple published series).
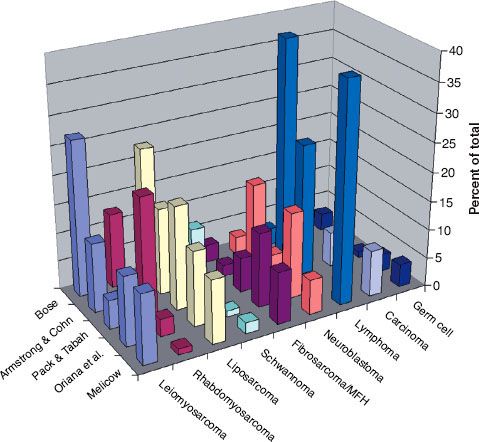
EPIDEMIOLOGY
The population frequency for retroperitoneal tumors has been quoted as three per million persons in two population-based studies.1,2 The age range of patients varied between 3 and 83 years. Fifty percent of patients are diagnosed between 60 and 80 years of age. Males and females are equally likely to develop lesions of the retroperitoneum.2
Figure 75.2 graphs the age at diagnosis of several large series of patients with primary retroperitoneal tumors. There are two peak age periods. The first peak occurs during the first decade and is generally caused by neuroblastoma or germ cell tumors. The second peak occurs during the sixth decade of life and is generally caused by mesenchymal lesions. These histologies are outlined in Figure 75.3.
TABLE 75.1 PRESENTING COMPLAINTS (%) OF PATIENTS WITH RETROPERITONEAL LESIONS (POOLED FROM LITERATURE SOURCES)
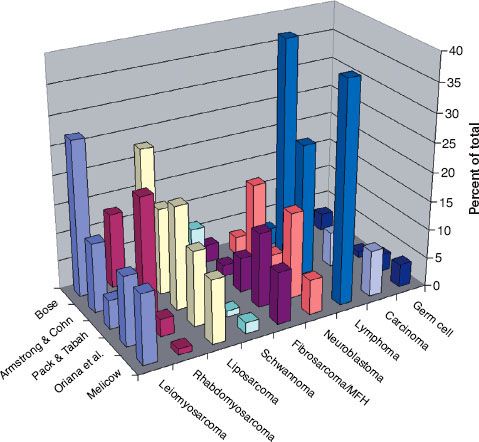
NATURAL HISTORY
The natural history of these lesions varies by histology. Lesions of the peritoneum often present having achieved a substantial size owing to the anatomic properties of the region.
CLINICAL PRESENTATION
Table 75.1 shows the summary of presenting symptoms and signs of tumors in the retroperitoneum at presentation.
DIAGNOSTIC WORKUP
Melicow8 considered the ureter to be “the ‘weather vane’ of the retroperitoneum” because on intravenous urography it is often displaced by tumor. Hydroureter and hydronephrosis are relative late events caused by retroperitoneal pathology given that the ureter and kidney at baseline are highly mobile. Bose2 published that approximately 80% of these patients had such findings that were suggestive of a mass.
Two series demonstrated that the vascular bed is abnormal in patients with retroperitoneal masses. In the first of these series, which was made up of angiographic studies performed in 20 patients by Oriana et al.,9 aberrant circulation was noted in 75% and mass effect in 85%. All patients undergoing angiography reported by Bose2 had abnormal studies.
Neville and Herts10 have published a comprehensive review of computed tomography (CT) appearance of primary retroperitoneal lesions. CT can differentiate between well-differentiated and dedifferentiated retroperitoneal liposarcoma. A focal nodular/water density area has been found to be a very sensitive marker of dedifferentiated tumor (sensitivity, 97.8%). CT scan can identify most dedifferentiated tumors accurately.11 Liposarcomas frequently are large lesions with fatty components causing mass effect. Teratomas can have numerous types of tissue imaging characteristics, reflecting their capacity to be made up of differing stromal types. Nerve sheath tumors and other tumors of the nerves are commonly paraspinal. CT characteristics of retroperitoneal tumors have been used in pediatric patients as well.12
Magnetic resonance imaging (MRI) often demonstrates additional imaging details to further classify primary lesions of the retroperitoneum, as reported by two groups.13,14 CT-guided needle biopsy may be performed to obtain initial tissue for histologic diagnosis when an open or laparoscopic biopsy is not possible. Positron emission tomography (PET) scans can be helpful in evaluating the extent of distant metastases during the staging workup. Fluorodeoxyglucose (FDG)-PET can complement CT and MRI for detecting high- and intermediate-grade local recurrence of retroperitoneal soft tissue sarcoma (STS).15
CONTEMPORARY POPULATION-BASED DEMOGRAPHICS
A comprehensive review of the Surveillance Epidemiology and End Results (SEER) public use registry focused on retroperitoneal tumors was performed by Lawenda and Johnstone.16 The SEER program is made up of 15 regions designed to collect information on cancer incidence, prevalence, and survival on a significant portion of the U.S. population. Table 75.2 shows the results of this review.
GENERAL MANAGEMENT OF RETROPERITONEAL SARCOMA
Gross total resection is the primary treatment for retroperitoneal sarcoma (RPS) and is attempted when possible. Aggressive surgical management is the key for long-term survival for patients with retroperitoneal STS.17,18,19 Criteria for unresectable tumors may include spinal cord or major vessel involvement.20 Recent series report that the rate of resectability ranges from 65% to 85%.21–23 En bloc resection of the primary tumor has been suggested as necessary for tumor control in the retroperitoneum.23,24
Surgical resection is often classified by the final margin status or R status as follows:
R0—gross or macroscopically complete tumor resection with microscopically negative margins,
R1—gross or macroscopically complete tumor resection with microscopically positive margins, and
R2—incomplete or partial tumor resection with gross tumor residual.
Achieving an R1 or macroscopically negative margin is a significant prognostic factor in local control and survival; however, the significance of a microscopically positive margin is less clear.22,25 Even with an R0 resection, the long-term outcomes of tumors of the retroperitoneum are poor: 33% to 77% (local recurrence) and 35% to 63% (5-year overall survival).22,23,26 Local recurrence is the primary cause of death from RPS.23,24 Local disease in the absence of metastatic disease was associated with death in 75% of patients with primary RPS in one series.22
No prospective data exist suggesting that postoperative radiation after surgery is superior to surgery alone for local control; multiple retrospective series support adjuvant radiation therapy.23,26 The effect of adjuvant radiation on overall survival is less certain. Preoperative or postoperative radiation therapy can be employed. Other modalities such as hyperthermia have shown early promise and can be of use for increased local control.20
Postoperative radiation is felt to be more toxic than preoperative radiation therapy because the clinical target volume (CTV) may be larger and typical contains a larger amount of normal tissue. Preoperative radiation therapy may be less toxic because fields are smaller, there is less normal tissue in fields as the tumor displaces these tissues “out of the way,” the tumor may be more easily resectable owing to a “rind” forming, and oxygenation may be better with an intact vasculature.
Lower dose to the region and better delineation of tumor with preoperative treatment are perhaps the most critical reasons why the trend is moving toward preoperative chemotherapy in Europe and many academic centers in North America. The role of preoperative radiation therapy was studied in the American College of Surgeons Oncology Group (ACOSOG) protocol Z9031, a phase III prospective randomized study of preoperative radiation plus surgery versus surgery alone for patients with RPS. The study, which accrued 370 patients, closed in 2006. Results have not yet been published.
Children’s Oncology Group (COG) protocol ARST0332 allowed both pre- and postoperative radiation therapy as well as significant chemotherapy. It just recently closed at the time of this writing and contains significant cross sections of histologies. Most accrued cases on the study have been treated with preoperative radiation therapy and chemotherapy. It allowed enrollment for patients up to age 30 years with nonrhabdomyosarcoma STS histologies.
Completeness of resection, tumor volume, grade, and subtype are prognostic factors of RPS.27 Preoperative radiotherapy or chemotherapy may be employed in cases of unresectable tumor as a means to attempt to shrink the tumor and make it resectable.28 A 60% local control rate was reported in a series of unresectable RPS patients employing doses of radiation of at least 63 Gy by the Massachusetts General Hospital.29 Patients with locally extensive tumors that are not amenable to complete resection can be offered palliative debulking surgery to help control local symptoms.30
Following aggressive surgery, local recurrence is a key factor determining morbidity and mortality. Local recurrence growth rate >0.9 cm per month has been associated with a poorer outcome.31 The use of higher doses of external-beam radiation therapy (EBRT) or intraoperative radiation therapy (IORT) does not result in significant improvement in outcome in such cases.32 Locally recurrent RPS is potentially surgically salvageable, with longer disease-free interval to recurrence being associated with better prognosis.23 Patients who have not received prior radiation to the tumor bed should be considered for either pre- or postoperative radiation therapy. IORT has been reported to increase local control after resection in several series33–35; however, in a randomized prospective study at the National Institutes of Health (NIH), IORT was shown to not be significantly better than EBRT.36
There is no standard way to manage patients who present or recur with distant metastases. Guidelines presented by the National Comprehensive Cancer Network (NCCN) for sarcoma management (version 2.2011) suggest that the same approach used for localized sarcoma patients be employed to deal with the oligometastatic population.37 In the case of oligometastatic disease, chemotherapy may be considered. With disease that is extensively metastatic, palliative approaches are used so as to focus on quality of life.
A histology-based system identifies RPS into three prognostic groups and can be used in both primary and recurrent RPS. Distinct risk stratification is necessary for specific assessment of prognosis and decisions regarding individualized adjuvant therapy.38 Some authors39 recommend the formulation of a liposarcoma-specific postoperative nomogram based on histologic subtypes, which provides more accurate survival predictions for patients with primary RPS. Well-differentiated and dedifferentiated liposarcoma differ significantly in their biologic behavior and are treated by different surgical approaches at the University of Texas MD Anderson Cancer Center.40 Researchers at the center recommend that the American Joint Committee on Cancer (AJCC) STS staging system needs the incorporation of primary site, histologic subtype, margin status, and recurrence to shed light on prognosis.41 Tumor size was found to have no correlation with survival.42 Patients with dedifferentiated retroperitoneal STS carry the worst prognosis according to a small series from Italy.43
Assessing the response to therapy is challenging and limited to either pathologic review of biopsy or resection specimens, or to serial imaging studies (i.e., CT or MRI with contrast enhancement). Based on the findings of a recently published meta-analysis, FDG-PET imaging may not accurately reflect the response to radiation therapy.44
CHEMOTHERAPY
Adjuvant chemotherapy is controversial in the management of adult patients with macroscopically completely resected retroperitoneal STS. In a National Cancer Institute trial, patients with STS were randomized to chemotherapy or observation following resection; some patients had postoperative irradiation.45 Among patients who were assigned to chemotherapy, survival was favorably affected in those with extremity tumors. However, patients with head and neck and truncal lesions (including RPS) did not benefit; the 5-year survival rate was approximately 40% in both arms. Similar findings were reported in a large meta-analysis of patients with STS.46
The use of neoadjuvant or adjuvant chemotherapy is not standard in the management of nonmetastatic adult retroperitoneal STS. Neoadjuvant chemotherapy has been primarily studied in the setting of high-grade extremity STS. Pisters et al.47 demonstrated feasibility of using preoperative concurrent doxorubicin and EBRT (18 to 50.4 Gy) followed by resection and IORT (15 Gy) in patients with RPS. An R0 or R1 resection was possible in 90% of the patients who went to surgery (83%). Despite these promising results, concurrent neoadjuvant chemoradiotherapy is not recommended outside clinical protocols.48
RADIATION THERAPY TECHNIQUES
Three-dimensional conformal radiation therapy (3DCRT) planning is preferable to conventional planning techniques to more clearly define target and nontarget tissues and to optimize field arrangements. Intensity-modulated radiation therapy and helical tomotherapy, proton beam therapy, and IORT have reported benefits over standard 3DCRT in enhancing the delivery of higher radiation doses to the target volume while minimizing doses to normal tissues.33–35,49–51
We recommend preoperative radiation therapy for the reasons outlined previously. This usually requires that an initial procedure be done (CT-guided core needle biopsy, if adequate tissue can be obtained, or a small operative procedure) to obtain tissue for histologic study. Obtaining a contrast-enhanced CT scan or MRI prior to simulation will help to more clearly define tumor and normal tissues for planning purposes. The use of [F-18]FDG-PET in tumor localization has been reported and may differentiate tumor versus surrounding normal tissues.52 Hybrid FDG-PET/CT is becoming increasingly popular for diagnosis and monitoring of treatment for RPS.52 Most cases of RPS will require irradiating the ipsilateral kidney, except in the case of proton therapy, where some kidney may be spared on a case-by-case basis. Therefore, a renal perfusion study should be ordered as part of the initial radiation planning process to ascertain the degree of functionality of each kidney.
Target volumes are defined as follows (preoperative volumes as per ACOSOG Z9031 as an example):
1. The gross tumor volume (GTV) is the visualized GTV based on preradiotherapy imaging with CT and/or MRI. PET/CT can also be useful.
2. The CTV is defined as the tissues adjacent to the GTV that have a potential for microscopic disease and are not visible on radiographic imaging. The CTV should be contoured at least 1.5 cm outside the GTV but can be less in areas where there is minimal risk of direct invasion (i.e., peritoneum, bone, muscle).
3. The planning target volume (PTV) is an expansion volume outside the CTV that accounts for setup error and patient/organ movement.
This variable is institution dependent as well as patient dependent. A minimum of 0.5 cm expansion outside the CTV should be used. Infraction organ motion of retroperitoneal structures can be significant—that is, average movement between 11 and 19 mm (kidneys, normal breathing) and 18 and 22 mm (pancreas, normal breathing).53 Stereotactic ultrasound-based image-guided targeting, cone-beam CT imaging, active breathing control, and respiratory gating may be useful modalities in decreasing the required PTV expansion owing to organ motion. Four-dimensional (4D) CT simulation may be of value in helping to define a GTV during the breath cycle54 but his needs to be better evaluated on clinical trials as it has been used primarily in the lung.55
Critical normal structures should be contoured (i.e., liver, kidneys, spinal cord, bowel, stomach) and dose-volume histograms calculated. The spinal cord dose should be limited to 45 Gy in standard fractionation over 5 weeks. The current ACOSOG Z9031 trial limits liver doses as follows: no more than 20% of the liver volume should receive >50 Gy, and no more than 50% should receive >25 Gy. At least two-thirds of the volume of one functioning kidney should receive <20 Gy. Stomach and bowel should be limited to a maximum dose of 45 Gy. Volume expansions should be minimized in regions where tolerance doses to critical structures will be reached.
In photon-based therapy, conventional anteroposterior/posteroanterior or slightly oblique fields often provide the best target coverage with the least amount of normal tissue in the beam. The use of lateral fields can result in irradiating a substantial volume of normal tissue (i.e., liver and kidneys) and should be used sparingly.
Preoperative radiation doses of 45 to 50 Gy (in 1.8 to 2 Gy per fraction per day) are recommended. Surgical resection usually is usually delayed until 3 to 8 weeks after completion of the radiation therapy. Radiographic restaging is typically done prior to resection to assess for metastases and response to therapy.
IORT with either brachytherapy or EBRT may be used as a focal boost to deliver additional radiation to areas of concern (i.e., close or positive margins). The addition of an IORT boost has been shown to increase local control rates over resection and EBRT alone.34,56 Although not always possible, care should be taken to avoid including critical structures from the boost (i.e., bowel, ureters, nerves). A postoperative external-beam boost can also be delivered to an area of close or positive margin. We recommend asking the surgeon to place radiopaque markers (i.e., clips, metallic seeds) at the borders of the resection cavity and in the areas where the margin may be close or positive. This will be helpful in defining these areas later if a postoperative boost is given. The boost dose is typically 10 to 15 Gy and is delivered in either a single intraoperative dose (electron beam or brachytherapy) or in a once-daily, fractionated regimen (1.8 to 2 Gy per fraction) postoperatively. Both low and high dose rate brachytherapy have similar efficacy in terms of disease control when used alone or in combination with EBRT. Brachytherapy results in fewer complications compared with combination of brachytherapy and EBRT.57
High dose rate intraoperative brachytherapy has been described in the treatment for primary and locally recurrent RPS. A Harrison-Anderson-Mick applicator (an array of catheters spaced 1 cm apart in a silicone rubber pad), or similar device, can be used to deliver an intraoperative dose of 12 to 15 Gy to the tumor bed, using iridium-192 (192Ir) sources.33 Adjuvant interstitial postoperative brachytherapy provides local control of tumor in both low- and high-grade STS.58
Postoperative radiation therapy is recommended for patients who initially present after resection. These fields frequently are more extensive than preoperative fields; thus, a larger volume of normal tissue is usually included. Normal tissues (i.e., stomach and bowel) that were previously displaced by the tumor mass will subsequently fill the resection cavity after the tumor has been removed, increasing the toxicity of the treatment and limiting the dose that can be delivered. Although not commonly employed, silicone-filled implants have been used to displace bowel and other tissues out of the radiation field.33 Postoperative radiation fields should include the preoperative GTV (as defined on the preoperative CT or MRI) and the entire resection cavity. Defining the tumor bed can be challenging; therefore, the radiation oncologist generally needs to err on the side of treatment that affects more, rather than less, normal tissue in cases that are uncertain. Residual disease may be visible on postoperative scans; however, surgical clips placed at the time of the resection will remove much of the uncertainty of defining these areas. Boost fields can encompass areas of close margins or residual disease. Expansion volumes (CTV and PTV) should be limited in regions where the tumor did not violate fascial or peritoneal boundaries. A postoperative radiation dose of 45 Gy (1.8 Gy per fraction per day) is recommended. Limited boost fields (5.4 to 9 Gy per fraction) can be planned, although careful attention must be paid to the surrounding normal tissue tolerances. Particle therapy may be advantageous in terms of decreasing the integral dose to the abdomen and pelvis and is under study.51,59
RESULTS OF THERAPY
Despite poor local control rates with resection alone, complete surgical resection remains the only curative treatment modality in patients with RPS.25 In patients with nonmetastatic, completely excised RPS, 5-year overall survival is 49% to 70%24,25,60 (Table 75.3). Failure to achieve local control of disease is the major cause of death in patients with RPS.21 In a large, single-institution report of RPS, patients who successfully underwent a gross total resection (n = 185) had a median survival of 103 months versus 18 months (n = 46) for those who underwent an incomplete resection.21 Similarly, Stoeckle et al.22 published actuarial 5-year overall survival rates of 62% versus 26% for patients who had a complete (n = 94) versus incomplete (n = 50) excision, respectively.
TABLE 75.3 COMPLETE RESECTION AND SURVIVAL IN PATIENTS WITH NONMETASTATIC (M0) RETROPERITONEAL SARCOMA AND SURVIVAL

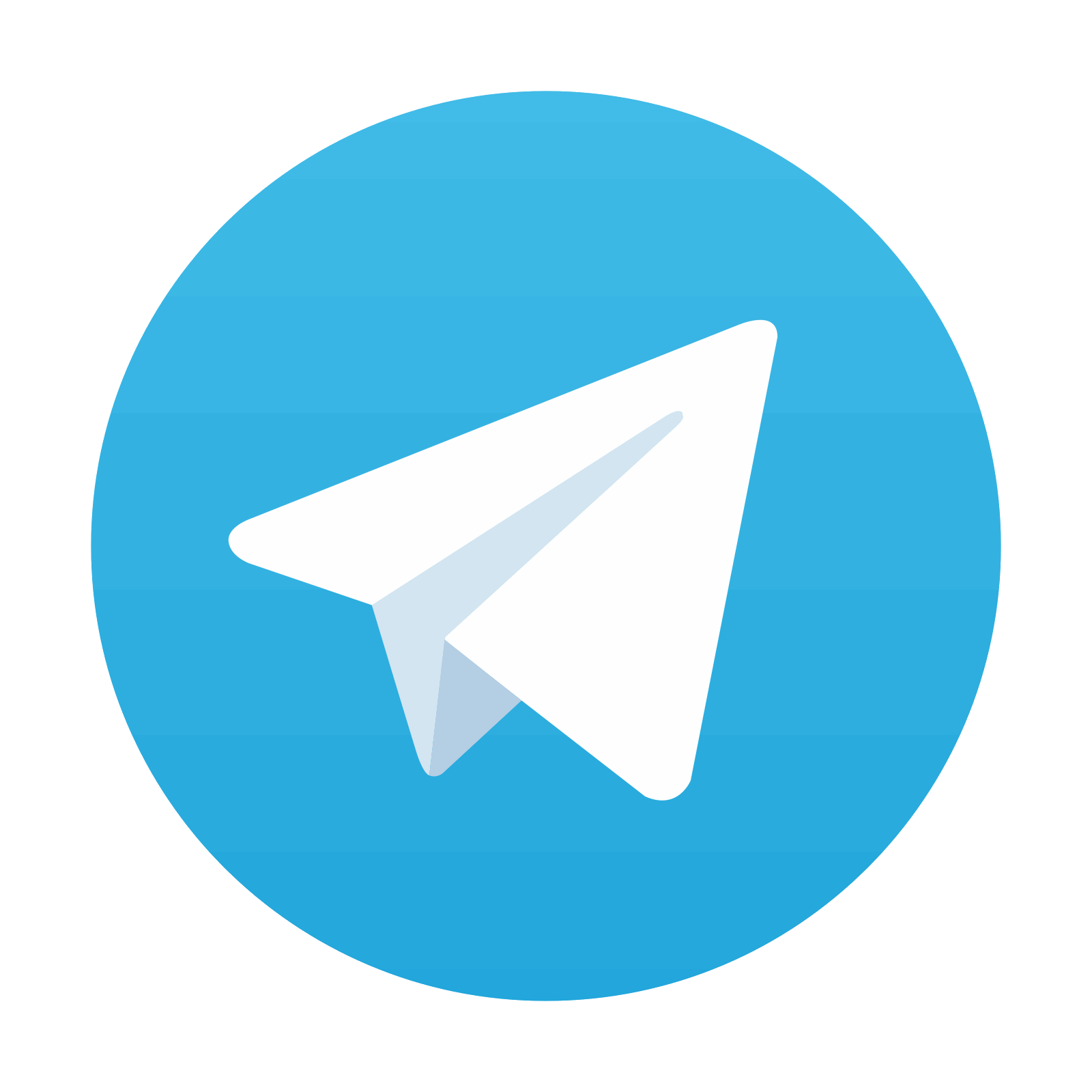