Puberty and its disorders in the female
DEVELOPMENT OF THE FEMALE REPRODUCTIVE SYSTEM
Maturation of the Neuroendocrine-Ovarian Axis
Regulation of the Neuroendocrine-Ovarian Axis
Adrenarche and the Regulation of Adrenal Androgen Secretion
Hormonal Secretion, Transport, Metabolism, and Action
NORMAL SEXUAL MATURATION: HORMONAL AND PHYSICAL STAGES
Introduction
By the mid-1960s, a general concept of the major factors involved in the initiation of puberty was established (Figure 15-1).1,2 A decrease in sensitivity of the brain “gonadostat” to sex hormone negative feedback was thought to be the primary event. This signaled the hypothalamus to discharge neurohumors (then unidentified), which in turn stimulated the pituitary to release gonadotropins. The resultant rise in secretion of the gonadotropins, luteinizing hormone (LH) and follicle-stimulating hormone (FSH), was thought to account directly for increased estrogen production by the ovary. A mature relationship was thought to develop in which the blood levels of estrogen and gonadotropins were regulated reciprocally via the gonadostat,3 much as a furnace is regulated by a thermostat. The pineal was identified as having gonadal suppressive properties. The increased adrenocortical secretion of 17-ketosteroids (17-KS), which becomes apparent at about the time of puberty (“adrenarche”), was thought to be due to a pituitary factor stimulating adrenal androgens in synergism with adrenocorticotropic hormone (ACTH).4
FIGURE 15-1 Schematic representation of the neuroendocrine-ovarian axis involved in normal pubertal development. ACTH, adrenocorticotropic hormone; CRF, corticotropin-releasing factor; GnRH, gonadotropin-releasing hormone; FSH, follicle-stimulating hormone; LH, luteinizing hormone.
Our present view of the mechanisms controlling puberty is more refined and complex than it once was, although the previously discussed schema is correct in a general sense. The gonadostat is a patently oversimplistic concept for a complex system that regulates the hypothalamic GnRH pulse generator, a functionally interconnected and synchronized network of GnRH neurons.5 The gonadostat setting seems to change throughout childhood in a biphasic manner. This concept is illustrated in Figure 15-2.6,7 During fetal and perinatal life, the gonadostat is insensitive to negative feedback by sex steroid hormones; at this time the nascent neuroendocrine-gonadal axis functions at a pubertal level. The gonadostat becomes increasingly sensitive to negative feedback during infancy, but it does not become highly sensitive until mid-childhood, at which time GnRH pulse generator activity is minimal. During late prepuberty the gonadostat begins to relinquish its inhibition. This permits the onset of puberty. The changing set point initially permits increasing, episodic secretion of GnRH. Increasing sensitivity of the pituitary gonadotropic cells to GnRH follows. The change in LH and FSH secretion is first detectable during sleep. Gradually the gonads become increasingly sensitized to gonadotropin stimulation, grow at an increased rate, and bring about sustained rises in plasma sex steroid hormone levels. Some of these phenomena synergize with others, so that autoamplification occurs and the pace of change accelerates. Eventually the set point for gonadotropin release comes to vary sufficiently to encompass a positive feedback mechanism.
FIGURE 15-2 The changing pattern of serum gonadotropins and sex hormones from fetal life to maturity in relationship to the apparent sensitivity of the central nervous system “gonadostat” to the negative feedback effect of sex hormones and the underlying hormonal events. FSH, follicle-stimulating hormone; GnRH, gonadotropin-releasing hormone; LH, luteinizing hormone. (Modified from M. Grumbach, C. Grave, & F. Mayer (Eds.), The control of the onset of puberty. New York: John Wiley & Sons.)
Development of the female reproductive system
Maturation of the neuroendocrine-ovarian axis
Fetus
Neuroendocrine unit.
The anterior lobe of the pituitary gland, of stromal ectodermal origin, and the posterior lobe, of neural origin, differentiate by 11 weeks’ gestational age.8 By this time, GnRH neurons have migrated from the olfactory placode into place in the medial basal hypothalamus.9 Hypothalamic GnRH subsequently rises in parallel with fetal pituitary and serum LH and FSH.10 All peak at about 20 to 24 weeks, as the connections of the pituitary portal system become complete, to levels not again seen until menopause.11
Serum LH and FSH levels are higher in human female than male fetuses.11 In rats, GnRH-containing neurons develop earlier in females than in males,12 and there are sexual dimorphisms in the degree of synapsing of specific tracts with dendritic spines in the preoptic nucleus, one of the major GnRH-containing areas of the hypothalamus.13,14 These differences may be determined by gonadal sex steroid hormone output. In all species studied, fetal secretion of LH, particularly LH pulse frequency, is permanently desensitized to estradiol (E2)-progesterone negative feedback by fetal virilization.15 In the rat this has been demonstrated to be mediated by permanent impairment of estradiol-induced progesterone receptor gene expression.16
In late gestation, fetal hypothalamic GnRH and pituitary gonadotropin secretion fall to low levels. These changes are likely explicable by the negative feedback effect of the high sex steroids produced by the fetoplacental unit. Meanwhile, maturation of the central nervous system (CNS) tracts that inhibit hypothalamic GnRH secretion and mediate gonadal negative feedback signals appears to progress throughout gestation.17,18
The production of gonadotropins by the fetal pituitary seems to facilitate normal ovarian development. Hypophysectomy of rhesus fetuses has been reported to reduce the number of germ cells and oocytes as well as the integrity of the rete ovarii.19 Therefore, it seems that the survival of gametes depends on the secretions of the fetal pituitary.
Ovary.
The ovaries differentiate in the urogenital ridge adjacent to the anlage of the adrenal cortex and the kidney. The granulosa cells are the homologues of the Sertoli cells of the testes. The theca, interstitial, and hilus cells are the homologues of the Leydig cells; hilus cells may even contain crystalloids like Leydig cells. Adrenocortical rests occasionally have been found in the hilus of the ovary.20 Conversely, ovarian rests have been identified in the adrenal glands.21
The primitive germ cells migrate into the ovary from the yolk sac endoderm during the first month of gestation. The ovaries begin to become distinguishable from testes by 8 weeks of gestation22 in the absence of testicular development being switched on by signaling cascade initiated by the SRY gene on the Y chromosome.23 Wnt-4 and forkhead (Fox) family transcription factor signaling by germ cells are critical for ovarian differentiation by sustaining oocyte and granulosa cell development and suppressing Sertoli and Leydig cell differentiation; they also support later aspects of follicle develoment.24 Steroidogenic factor-1 (SF-1) WT-1, LIM-1, and possibly DAX-1 genes play roles in the formation of the ovaries.25 Germ cell bone morphogenetic proteins (BMPs) are necessary for primordial germ cell proliferation. Mitotic division of oogonia is maximal before the third month and comes to an end by the seventh month. Oogonia then undergo oogenesis, entering the prophase of meiosis to become primary oocytes during the final 5 to 6 months of gestation.26 The number of oocytes reaches a peak at the fifth month when there are 6.8 million germ cells, of which 80% appear to be viable (Figure 15-3).27 When oocytes enter the diplotene stage of meiotic prophase, they must be furnished with granulosa cells to form a primordial follicle, or else they undergo atresia.28
FIGURE 15-3 The development of ovarian follicles from fetal life to maturity. Curves for total number of viable germ cells (thick line) and large antral follicles (thin line) smoothed from the data of Baker and Block. The number of germ cells is maximal at the fifth month of fetal life. The loss of germ cells is exponential throughout postnatal life. At puberty, a marked shift occurs in the pattern of development of follicles. An increased fraction grows to large antral size.
Primordial follicles appear in the fourth month, when the epithelium of the secondary sex cords provides granulosa cells to the oocytes, and they peak in number between the fifth and ninth months. They become primary follicles when the encircling granulosa cells become cuboidal. Primordial and small primary follicles (Figure 15-4)29,30 are resting follicles, which are the major repository of germ cells.31 This stock of germ cells is depleted only very slowly during childhood (see Figure 15-3). Secondary follicles and preantral follicles, characterized, respectively, by organization of theca and a larger granulosa cell population, then appear successively. After the seventh month, antral (graafian) follicles appear and those granulosa cells enveloping the oocyte become the cumulus.30,32,33 Typically one or two antral follicles of 1 to 2 mm in diameter are present in the ovary by term, at which time the number of small preantral follicles is at its peak. At term, ovarian follicle development is complete,30,32,33 and the complement of ova is greater than at any other time during postnatal life (see Figure 15-3), totaling 2 million, of which half appear atretic.27,34
FIGURE 15-4 The human ovary. The lower portion of the figure shows the classification of follicles. Preantral follicles contain as many as 300 granulosa cells, and their diameter ranges from 50 to 200 μm. The oocyte diameter increases from 25 or less to 80 μm. Antral (graafian, tertiary, or vesicular) follicles have a fluid-filled antrum and a full-grown oocyte, are lined with more than 300 granulosa cells, and have a well-developed theca. They are greater than 200 μm in diameter. The dimensions of the mature ovary are approximately 1.25 × 2.75 × 4 cm. The upper portion of the figure illustrates the histologic appearance of the perimenarcheal ovary. (Photomicrographs of ovarian details are reproduced from Peters, H. (1979). The human ovary in childhood and early maturity. Eur J Obstet Gynecol Reprod Biol, 9, 137; modified from Ross, G. T., Schreiber, J. R. (1978). The ovary. In S. S. C. Yen, & R. Jaffe (Eds.), Reproductive endocrinology (p. 63). Philadelphia: WB Saunders.)
Both X chromosomes are active in oocytes,35 and these are necessary for the induction of the granulosa cell layer necessary for oocyte survival.32,36 Several oocyte transcription factors then mediate the transition from primordial to primary follicles.24 Growth differentiation factor-9 is critical for signaling the granulosa cells of primary follicles to induce the theca cell layer. Other autosomally determined paracrine factors, prominently involving TGF-β superfamily signaling, are necessary for the survival of oocytes and the further differentiation and growth of follicles. The forkhead transcription factor FOXL2, expressed specifically in granulosa cells, both mediates the GDF9 effect and restrains it from prematurely activating oocytes.37
Follicle number is determined by the balance between the number of ovarian germ cells and the rate of atresia. The endowment of ovarian germ cells has been thought to be determined during fetal life, as the germ cells of the ovary, unlike those of the testes, seem to be a non-renewing population; however, this concept has been challenged by research with germline stem cells that suggests a process of slow neogenesis.38 Regardless, the regulation of atresia by the balance of cell survival and programmed cell death signals is a critical determinant of follicle number.39 The endowment of follicles may be influenced by environmental factors, such as toxins,40 thymic factors,41 and placental insufficiency.42 Fetal undernutrition has been suspected of temporarily or permanently altering ovarian follicular development.43–45
Theca-interstitial cells of the fetal primordial follicle form androstenedione and dehydroepiandrosterone as early as 3 months gestation in humans, but E2 formation probably does not occur until antral follicular development begins at 34-38 weeks gestation.18,22,46 Thus, the contribution of the ovaries to fetal sex steroid levels is probably relatively low.
Placenta.
The fetoplacental unit becomes the major source of sex hormones in the female fetus in the latter half of pregnancy: the fetal adrenal gland provides 17-ketosteroids as substrate for the formation of potent sex steroids by the placenta. Excess androgen, from any source, in the female fetus masculinizes genital differentiation, as discussed in other chapters. This also programs for LH elevation and insulin resistance in adult life.15 Placental insufficiency, via hypoxemia and the resultant overactivation of fetal prostaglandin production and cortisol secretion, is another factor predisposing to postnatal insulin resistance.47
Infant and child
Neuroendocrine unit.
Serum FSH and LH are low in cord blood and remain low until estrogen concentrations fall from inhibitory levels upon disruption of the fetoplacental unit at birth. Then the LH and FSH levels of neonates promptly begin to rise in pulsatile fashion to early pubertal levels in the first week of life (Figure 15-5).7,11,48–51 This pituitary activity wanes in older infants, which may be related to the maturation of neural tracts that conduct inhibitory signals from the CNS.
FIGURE 15-5 The distribution of serum gonadotropin levels according to early generation radioimmunoassays from birth to adulthood (age in years) Left, Follicle-stimulating hormone (FSH). Right: Luteinizing hormone (LH). Umbilical cord level of LH measured by beta-subunit-specific radioimmunoassay. Standard LER-907: 100 ng equivalent to 2 mIU FSH and 6 mIU LH of the First International Reference Preparation of human pituitary gonadotropin for bioassay. (Data from Winter, J., Faiman, C., Hobson, W., et al. (1975). Pituitary-gonadal relations in infancy: I. Patterns of serum gonadotropin concentrations from birth to four years of age in the human and the chimpanzee. J Clin Endocrinol Metab, 40, 545; from Kaplan, S., Grumbach, M., & Aubert, M. (1976). The ontogenesis of pituitary hormones and hypothalamic factors in the human fetus. Recent Prog Horm Res, 32, 161.)
Serum LH and FSH levels rise higher in female than male premature infants, reaching into the postmenopausal range.17,52 This sexual dimorphism seems to be related to a lack of negative feedback due to lagging ovarian follicular development: antral follicle development begins near term gestational age.18 There is parallel hyperprolactinemia without sexual dimorphism.53
By 40 weeks’ gestational age, serum gonadotropins and LH/FSH ratios fall to lower levels in girls than in boys,17 apparently because girls lack androgen-programmed accentuation of GnRH pulsatility.16,54,55 Responses to GnRH and GnRH agonist are similar to those of early puberty (Figure 15-6).56–59 In congenital agonadism, gonadotropins reach postmenopausal levels during the neonatal period.60
FIGURE 15-6 Basal and peak responses to the gonadotropin-releasing hormone agonist nafarelin (1 μg/kg subcutaneously) during development. Lines connect the basal and peak responses in control children. The responses are related to bone age in children and chronologic age in adults. Note the biphasic pattern of the responses. They are high in infancy, lower in mid-childhood, and rise again during puberty. The peak gonadotropin responses occur at approximately 4 hours, and peak estradiol responses occur at 20 hours. FSH, follicle-stimulating hormone; LH, luteinizing hormone. (From Rosenfield, R. L., Burstein, S., Cuttler, L., et al. (1989). Use of nafarelin for testing pituitary-ovarian function. J Reprod Med, 34, 1044.)
After about 4 months of age, gonadotropin and prolactin levels gradually begin to fall into the prepubertal range (see Figure 15-5). FSH is higher in girls than in boys, a tendency that tends to persist into early childhood.48,61 This appears in part related to negative feedback by the higher activin-A and lower inhibin-B serum levels of girls than boys.62 GnRH secretion also appears to be greater in girls than in boys at this time.63
The subsequent decline in gonadotropins may be related to an increase in hypothalamic sex steroid receptors. Hypothalamic estrogen receptors increase in a pattern reciprocal to the fall in serum gonadotropins in the rat (Figure 15-7),64 as do hypothalamic dihydrotestosterone (DHT) receptors.65 Increasing sensitivity of the hypothalamus to sex steroid hormone negative feedback could account for the inhibitory effect of the small amounts of circulating E2 and testosterone.
FIGURE 15-7 Relationship of maturation of hypothalamic estrogen receptors (top) to serum gonadotropin levels (bottom) in the developing female rat. FSH, follicle-stimulating hormone; LH, luteinizing hormone. (From Rosenfield, R. L. [1977]. Hormonal events and disorders of puberty. In J. R. Givens (Ed.), Gynecologic endocrinology. Chicago: Year Book Medical. By permission of Mosby-Year Book.)
A nadir in both serum gonadotropins occurs by about 6 years of age (see Figures 15-2 and 15-5). At this age the LH and FSH response to GnRH is minimal, apparently from lack of GnRH stimulation. Furthermore, at this stage agonadism is seldom reflected in a rise in serum gonadotropins or gonadotropin reserve.60
However, gonadotropin production is not completely suppressed in mid-childhood. Gonadotropins have been detected in the urine of young prepubertal children, at the limits of sensitivity of classic bioassays: LH excretion averaged 3% and FSH 15% of the adult amounts.66 Specific monoclonal antibody-based assays have revealed that LH falls to less than 0.2 U/L during the day, whereas FSH remains detectable, and that the gonadotropins produced at this stage are secreted in micropulses that approximately double in association with sleep.67 The gonadotropins also appear to be bioactive judging from their sensitivity to E2 negative feedback in the primate68 and the active formation of antral follicles during childhood, which indicates gonadotropin stimulation, as discussed in the following section on the adult.
Between 7 and 10 years of age, even prepubertal girls experience subtle but significant increases in gonadotropin levels.69 This change corresponds with rising secretion of GnRH.63 These data indicate that the hormonal secretory pattern of the prepubertal 10-year old child is different from that of the 7-year old and indicate that the hormonal changes signaling the development of puberty are found late in the first decade of life, antedating by some time the development of secondary sex characteristics.
Ovary.
The ovary of the infant and child is not quiescent. Initiation of growth and development of resting follicles occurs throughout childhood. The neonatal ovary typically contain an antral follicle with thecal luteinization,70,71 and the number of antral follicles approximately doubles over that in infancy by 7 years and quadruples by 9 years (see Figure 15-3).28 All these antral follicles normally undergo atresia in childhood, and this augments the amount of stroma.28 As a result, by mid-childhood the ovaries of normal girls have up to 5 antral follicles 4 to 9 mm in diameter, and ovarian volume increases up to approximately 3.5 cc. Ovarian morphologic development begins to accelerate just before the onset of puberty.28,72–76 Small follicle growth is reflected in serum anti-Müllerian hormone (AMH), which achieves adult levels by the time puberty begins.77
During the first few months of life, early pubertal blood levels of ovarian hormones are found as part of the transient activation of the hypothalamic-pituitary-gonadal axis that occurs in the newborn. Serum E2 and inhibin-B levels parallel those of FSH. In the neonatal period they begin rising to early pubertal levels, remain there for the first few months of life, and fall gradually thereafter (Figure 15-8).56,59 In premature infants ovarian function is delayed until near term gestational age, and it is then exaggerated and prolonged.18 The high gonadotropins of prematurity sometimes cause ovarian hyperstimulation in preterm infants.78 Breast diameter peaks at 2 to 4 weeks of age in term infants, later in premature infants, and commonly persists for several months.79 According to an ultrasensitive recombinant cell bioassay, girls’ estrogen levels in late infancy are several-fold greater than those of boys, averaging 1 pg/mL and ranging up to 3 pg/mL.80 On occasion there may be subclinical but detectable estrogen effects on urogenital cytology.81
FIGURE 15-8 The distribution of plasma estradiol levels in infant females compared with pubertal and adult female levels. The columns represent the normal ranges for the various stages of puberty. The area between 10th and 90th percentiles is dark. Stage P1 includes all prepubertal girls older than 2 years. The values between the ordinates were found between 2 and 5 days of age. (From Bidlingmeier, F., & Knorr, D. (1978). Oestrogens: physiological and clinical aspects. Pediatr Adolesc Endocrinol, 4, 43.)
In mid-childhood, gonadotropin secretion in response to GnRH agonist testing elicits a prompt small rise in E2 secretion.82,83 As girls begin to experience increasing diurnal production of gonadotropins in late prepuberty, E2 levels rise in diurnal fashion to approximate 10 pg/mL in midmorning.69,84
Adolescent
Increased GnRH secretion in humans was initially deduced when Kastin, Job, Grumbach, and their collaborators demonstrated that preadolescent children had GnRH-releasable pituitary stores of LH and FSH (Figure 15-9; see Figure 15-6).85 Subsequently, it was reported that in humans the output of an immunoreactive fragment of GnRH increases to adult levels during puberty.63,86 Studies in the rat suggest that hypothalamic GnRH increases through puberty.87
FIGURE 15-9 The luteinizing hormone (LH) and follicle-stimulating hormone (FSH) responses to gonadotropin-releasing hormone (GnRH) bolus (50 μg/kg/day) in males (M) and females (F) in prepuberty (age 5 to 6 years: F1, M1), early puberty (F2, M2), and later puberty (F5, M5). The responses to GnRH tend to progress with advancing puberty. However, early pubertal girls have a readily releasable FSH pool that is greater than that of more advanced adolescents. The peak responses of girls tend to be somewhat greater than those of boys at comparable stages. (Data from Dickerman, Z., Prager-Lewin, R., & Laron, Z. (1976). Response of plasma LH and FSH to synthetic LHRH in children at various pubertal stages. Am J Dis Child, 130, 634.)
Knobil subsequently showed that puberty can be induced in the immature female rhesus monkey by administering GnRH in hourly pulses that yield blood levels of about 2000 pg/mL.88 Prolonged administration of GnRH according to this regimen first gradually brings about transient increases in LH and FSH. This then induces cyclic follicular development. The resultant moderate E2 surge is of such magnitude as to result in menarche due to withdrawal menstrual bleeding in an anovulatory cycle (Figure 15-10). Continuation of the same GnRH regimen leads to the development of normal monthly ovulatory menstrual periods. Physiologic pulses of GnRH in humans probably attain lower concentrations (200 pg/mL) and occur at slightly wider intervals than in monkeys.89 Consequently, LH pulses in mature women occur at intervals of approximately 1 to 1.5 hour during the follicular phases, slowing during the luteal phase.90
FIGURE 15-10 Induction of puberty in a 13-month-old prepubertal rhesus monkey by an unvarying pulsatile gonadotropin-releasing hormone (GnRH) regimen (1 μg/min × 6 min hourly). Luteinizing hormone (LH), follicle-stimulating hormone (FSH), estradiol (E2), and progesterone were undetectable before the GnRH infusion. On GnRH infusion, a rise in FSH was the first change detectable by midmorning sampling midway between GnRH pulses. A substantial E2 surge occurred approximately 1 month later. The subsequent LH surge was too modest to elicit ovulation, but menses (M) occurred a few days after subsidence of the weeklong E2 surge—menarche resulting from an anovulatory cycle. Continuation of the GnRH led to the sustained occurrence of ovulatory menstrual cycles at 28-day intervals. An identical outcome results if an arcuate-lesioned adult animal undergoes this GnRH regimen. The third of the LH surges occurred 2 days after GnRH was discontinued. Progesterone secretion from the corpus luteum was blunted and transient in the absence of sustained LH secretion. A subsequent increase in plasma E2 produced by E2 implantation subcutaneously failed to elicit a gonadotropin surge, indicating that the animal had reverted to an immature state. Menarche eventually spontaneously recurred in such animals at the usual age (approximately 27 months). Small vertical lines beneath data points indicate values below the sensitivity of the assay. Note that gonadotropins and E2 were often undetectable (prepubertal range) during the induced puberty. (From Knobil, E. (1980). The neuroendocrine control of the menstrual cycle. Recent Prog Horm Res, 36, 53.)
Puberty begins when GnRH secretion increases. Serum LH then begins to rise disproportionately to FSH; this LH-FSH disparity is particularly evident during sleep, which is reflected in responses to GnRH or GnRH agonist (Table 15-1). Puberty becomes clinically apparent as thelarche when E2 levels are sustained > 10 pg/mL.83 It seems likely that a rise in inhibin-B as increasing ovarian follicles develop plays a key negative-feedback role in limiting further increase in FSH levels during puberty. FSH levels become less GnRH dependent during puberty.67 The mechanisms for differential regulation of FSH and LH are discussed later in this chapter.
TABLE 15-1
Typical Normal Ranges for LH, FSH, and Ovarian Steroids, at Baseline and in Response to ACTH and GnRH Agonist Testsa
DHEA, dehydroepiandrosterone; 17PREG, 17-hydroxypregnenolone; 17PROG, 17-hydroxyprogesterone.
a5th-95th percentile for third generation gonadotropin immunoassays and high-specificity assays after preparatory chromatography, except for DHEAS. Values differ slightly among laboratories.
b17-hydroxyprogesterone early follicular phase baseline levels. 130 ng/dL are found in women who are heterozygous for 21-hydroxylase deficiency, and they often have responses to ACTH greater than those shown. 17PROG begins rising during the late follicular phase and peaks as high as 400 ng/dL in the luteal phase of the cycle.
cAt 1600 hours after dexamethasone administration (0.5 mg po at 1200 hours) to blunt coincidental adrenocortical secretion.
dBaseline early morning serum LH: Prepubertal # 0.15 to 0.6, premenarcheal 0.1 to 7.2, postmenarcheal 1.4 to 5.3 U/L.
eBaseline early morning serum FSH: Prepubertal 0.5 to 2.9, premenarcheal 1.1 to 9, postmenarcheal 3.8 to 9.2 U/L.
Data from Rosenfield, R. L. (2007). Identifying children at risk of polycystic ovary syndrome. J Clin Endocrinol Metab, 92, 787–791; Rosenfield, R. L., Bordini, B., & Yu, C. (2013). Comparison of detection of normal puberty in girls by a hormonal sleep test and a gonadotropin-releasing hormone agonist test. J Clin Endocrinol Metab, 98, 1591–1601; Mortensen, M., Ehrmann, D. A., Littlejohn, E., & Rosenfield, R. L. (2009). Asymptomatic volunteers with a polycystic ovary are a functionally distinct but heterogeneous population. J Clin Endocrinol Metab, 94, 1579–1586; Forest, M. (1979). Function of the ovary in the neonate and infant. Eur J Obstet Gynecol Reprod Biol, 9, 145–160; de Peretti, E., & Forest, M. G. (1982). Pitfalls in the etiological diagnosis of congenital adrenal hyperplasia in the early neonatal period. Horm Res, 16, 10–22.
Pubertal gonadotropin cycles seem to develop well before menarche7,91 and are capable of inducing cyclic estrogen production.81,91 Our working model of the nature of pituitary-ovarian dynamics in early puberty is illustrated in Figure 15-11.
FIGURE 15-11 Diagram depicting our working hypothesis of the hormonal patterns in girls during very early puberty. We conceptualize this pattern as occurring both cyclically in the earliest stage of normal puberty and occasionally in unsustained sexual precocity (i.e., most U.S. cases of idiopathic premature thelarche). Daytime and nighttime serum concentrations of hormones (gonadotropins relative to the LER-907 standard) and the percentage of intermediate cells on vaginal smear are shown. The typical response to a gonadotropin-releasing hormone (GnRH) test is illustrated. Subclinical hormonal cycles lasting approximately 1 month result from a few days of increased follicle-stimulating hormone (FSH) and luteinizing hormone (LH) secretion. Because the drive to gonadotropin release is relatively weak, FSH and LH production are suppressed promptly and for long periods of time by the resultant modest amounts of estradiol (E2) secretion. Estradiol is detectable in plasma for only a few days a month. Maturation of the vaginal mucosa, however, is detectable for approximately 2 weeks after E2 production has waned.
Puberty progresses as LH rises. Whereas serum FSH levels rise about 2.5-fold over the course of puberty, LH levels rise 25-fold or more.67 The initial change in LH secretion at the beginning of puberty is a nightly increase in LH secretion that begins within 20 minutes of the onset of sleep. Subsequently, LH increases more with the onset of sleep, stays up longer, and falls less during waking hours. As the child approaches menarche, the daytime LH levels continue to increase until the diurnal rhythm is typically lost. FSH levels follow a similar pattern, although the FSH changes are less striking. The gonadotropin diurnal rhythm during puberty seems entirely related to sleep, unlike the cortisol circadian rhythm.92 There is a delay of about 12 hours between the peak LH level during sleep and the E2 zenith, such that E2 levels are maximal between late morning and early afternoon.84,93 The gonadotropin and E2 rhythms in an early pubertal girl are shown in Figure 15-12.93
FIGURE 15-12 The patterns of serum luteinizing hormone (LH), follicle-stimulating hormone (FSH), and estradiol (E2) typical of early female puberty. Note that daytime gonadotropin levels are in the prepubertal range. Note also the episodic nature of LH release at intervals of 1 to 3 hours. Estradiol levels are seen to fluctuate considerably in the course of the daytime, rising to peak levels about 12 hours after the maximum nocturnal gonadotropin surges. (From Boyar, R. M., Wu, R. H. K., Roffwarg, H., et al. (1976). Human puberty: 24-hour estradiol patterns in pubertal girls. J Clin Endocrinol Metab, 43, 1418.)
Augmentation of the bioactivity of serum LH occurs during pubertal progression. Plasma LH bioactivity rises nearly fivefold more during the course of puberty than does LH as measured by polyclonal RIA (Figure 15-13).94,95 The change in bioactive LH is mirrored well by the “third generation” monoclonal antibody-based immunometric (“pediatric”) assays that have very high specificity for bioactive LH epitopes. However, disparities in the ratio of bioactive to immunoreactive LH (B/I) persist with these assays, for reasons related to the molecular microheterogeneity of gonadotropins, which is discussed later. Serum FSH rises during puberty according to immunoassay more so than by bioassay.96
FIGURE 15-13 Bioactive luteinizing hormone (B-LH) (right) and immunoreactive LH (I-LH) (left) in the same daytime serum samples of girls 10 to 16 years of age at various pubertal stages. The dashed lines indicate the limit of sensitivity of the assays. B-LH rises relatively more than I-LH in the course of puberty. The peak in the apparent biopotency of LH, estimated from the ratio of B-LH to I-LH, occurs at about the time of menarche. Late indicates early follicular phase normally menstruating postmenarcheal girls. Standard LER-907: 100 ng equivalent to 6 mIU LH of First International Reference Preparation (IRP) of human pituitary gonadotropin. The disparity between immunoreactivity and bioactivity is principally due to the presence of different proportions of immunoreactive and bioactive LH moieties in serum and standards. The ratio of bioactivity to immunoreactivity is closer to unity when sera are assayed against highly purified standards (like the first and second IRP of human luteinizing hormone), which have about fivefold higher specific activity and yield dose response relationships with serum LH that are more linear. (Data from Lucky, A. W., Rich, B. H., Rosenfield, R. L., et al. (1980). LH bioactivity increases more than immunoreactivity during puberty. J Pediatr, 97, 205; Rosenfield, R. L., Helke, J. (1992). Is an immunoassay available for the measurement of bioactive LH in serum? J Androl, 13, 1.)
E2 output increases rapidly in the year approaching menarche.97 This seems to be the result of a variety of autoamplification phenomena that facilitate puberty, maturation of the dominant follicle, and ovulation. These are summarized in Box 15-1.98–111 These phenomena occur at all levels of the axis. The CNS is stimulated by preovulatory levels of E2 to increase GnRH pulse amplitude. At the pituitary level there is the self-priming effect of GnRH, whereby a pulse of GnRH sensitizes the pituitary to have a greater LH response to a subsequent identical GnRH pulse. Critical patterns of E2 and progesterone secretion enhance the pituitary LH and FSH responsiveness to GnRH. At the gonadal level, the cascade of events is augmented by the FSH induction of aromatase activity and progestin production in granulosa cells, phenomena in which androgens play a synergistic role. Furthermore, FSH stimulates granulosa cell mitosis and induces LH receptors, phenomena in which E2 may play a synergistic role. Subsequently, LH is able to further enhance the aromatase and progesterone effects. Progesterone itself plays a synergistic role in stimulating granulosa cell progesterone and prostaglandin synthesis in concert with both FSH and LH. In the rat, ovarian GnRH receptor sites also diminish just before ovulation,112 and at about this time the ovary changes its pattern of metabolism so that the secretion of androstanediol-3β-monosulfate decreases to levels that are no longer inhibitory to LH secretion.113
The preovulatory gonadotropin surge occurs when all these cascading processes culminate in activation of the positive feedback mechanism, the hallmark of sexual maturity in the female. “Positive feedback” refers to the neuroendocrine system acquiring the ability to secrete a midcycle surge of LH when the ovary signals via increasing estrogen secretion that it is prepared for ovulation.
Menarche does not necessarily indicate full maturation of the neuroendocrine-ovarian axis. As the studies of Knobil illustrate (see Figure 15-10), menarche can be due to estrogen-withdrawal bleeding—and it is about half of the time—but ovulatory cycles may follow in short order. General characteristics of the mature ovary are shown in Figure 15-4.
The morphology of the normal adolescent ovary has long been considered polycystic, and histologic examination typically has shown thecal luteinization.70,114 In the perimenarcheal period, the combination of a high number of follicles and mature gonadotropin stimulation leads to a greater number of large antral follicles than at any other stage (see Figure 15-3),27 which often leads to a “multifollicular” ultrasonographic appearance.72,115,116 By 1 year after menarche, at approximately 14 years of age, the ovaries normally achieve adult functional and anatomic characteristics. Many exceed normal adult ultrasonographic size or follicle count criteria, the largest reaching approximately a volume of 10.8 to 11.8 mL with 10-17 small (2-9 mm) antral follicles in the maximum plane.73,116–119 Thus, many eumenorrheic normal postmenarcheal adolescents meet current ultrasonographic criteria for polycystic ovary morphology (see the “polycystic ovary syndrome” section),120 often transiently.121
Adult
The follicular phase of each menstrual cycle recapitulates puberty in many respects. Gonadotropin and sex hormone levels are low during the premenstrual phase of the mature cycle (Figure 15-14A).122,123 Gonadotropin concentrations then increase at the time of menstruation, FSH predominating in the early follicular phase while nocturnal LH pulsation is slow124 (Figure 15-14B). Luteinizing hormone pulsation increases to a circhoral pattern around a stable baseline, and E2 production slowly begins as antral follicles develop (Figure 15-14C). E2 levels gradually increase and serum FSH levels fall reciprocally (Figure 15-14D). Upon formation of a dominant follicle, serum E2 concentrations increase geometrically. This selectively begins to amplify the pituitary’s LH response to GnRH as E2 reaches about 90 pg/mL for over 3 days104,105,125 (Figure 15-14E).
FIGURE 15-14 Diagram of gonadotropin and female hormone levels during the normal menstrual cycle. The levels are centered in reference to the day of the midcycle luteinizing hormone (LH) peak (day 0). Letters A through F above the top panel correspond to stages of follicular development in Figure 15-15. G and H are discussed in the text. M (bottom panel) shows time of menses. E2, estradiol; FSH, follicle-stimulating hormone; PROG, progesterone. (Based on data of Abraham, G. E. (1974). Ovarian and adrenal contributions to peripheral androgens during the menstrual cycle. J Clin Endocrinol Metab, 39, 340; Ross, G. T., Cargille, C. M., Lipsett, M. B., et al. (1970). Pituitary and gonadal hormones in women during spontaneous and induced ovulatory cycles. Recent Prog Horm Res, 26, 1; Soules, M., Steiner, R., Clifton, D., et al. (1984). Progesterone modulation of pulsatile luteinizing hormone secretion in normal women. J Clin Endocrinol Metab, 58, 378.)
When the serum estradiol rises to over 200 to 300 pg/mL for 36 hours, the positive feedback mechanism is activated and the midcycle gonadotropin surge commences (Figure 15-14F). E2 then appears to induce progesterone receptor (PR) expression in the hypothalamus and pituitary.126 An increase in progesterone to 100 ng/dL facilitates the LH surge, shortens the duration of time over which E2 is required for the surge to 24 hours, and brings about an FSH surge. The mechanism of progesterone action involves inhibition of GnRH cleavage.106 Androgens may also play a role in facilitating FSH and GnRH release.127,128 The LH surge is then primarily responsible for luteinizing the preovulatory ovarian follicle (see Figure 15-14F). At this time, LH pulses become larger in amplitude but slower in frequency and their apparent bioactivity increases. Ovulation then results.
As the follicle is disrupted by ovulation, estrogen levels fall (Figure 15-14G). As the corpus luteum begins to form, progesterone increases steadily to be sustained at very high levels for several days, along with lesser but substantial increases in E2 and 17-hydroxyprogesterone levels.122,123,129 In response to the high progesterone level, LH pulses become slow and large.124,129 In the absence of increasing human chorionic gonadotropin (hCG) from a conceptus, the corpus luteum’s life span is exhausted and its production of progesterone and E2 wanes. Subsequently, FSH begins to rise out of proportion to LH. Shortly after the sex steroids withdraw from the scene, the endometrium sloughs, giving rise to menstrual flow. Meanwhile, the follicular growth induced earlier by FSH begins to gain momentum and the next cycle begins.
Follicular (proliferative) phase ovary.
The hormonal functions of the follicle have dual purposes that must be closely coordinated: to change the milieu of the ovum to prepare for ovulation and to signal the pituitary to send the signal to ovulate (i.e., the LH surge). Thus, the ovary is the zeitgeber for the cycle: the normal cyclic pattern of ovarian hormone secretion induces the midcycle surge of pituitary gonadotropins, even in the presence of unchanging circhoral pulses of GnRH.88 Ovarian hormones also augment the amplitude of the GnRH response,98–101 which is a fail-safe mechanism that “guarantees” a preovulatory gonadotropin surge.
Ovarian follicular development and steroid secretion in relationship to changing gonadotropin levels are illustrated in Figure 15-15.31,130–132 FSH and LH play major roles in granulosa and thecal cell differentiation, respectively, whereas a host of local factors modulate gonadotropin action. For example, follicular maturation in response to gonadotropins is enhanced by insulin-like growth factors (IGFs), transforming growth factor (TGF)-β and fibroblast growth factor, whereas it is inhibited by TGF-α, epidermal growth factor, and other factors.
FIGURE 15-15 Relationships among gonadotropins, the ovarian follicle, and ovarian steroids according to the two-cell two-gonadotropin model of ovarian steroidogenesis. A through F, Stages of ovarian follicular development found during the times of the menstrual cycle designated by the corresponding letters on Figure 15-14. The size of the letters designating hormones relates to the magnitude of their serum and/or follicular concentrations. A, Preantral follicle with luteinizing hormone (LH) and follicle-stimulating hormone (FSH) receptors in theca and granulosa cells, respectively. There is no antrum surrounding the ovum (stippled in center). B, Small antral follicle. Activin up-regulates FSH receptors, and the FSH receptor activation is required to initiate antrum formation. C, Large antral follicle (1 mm or larger). Aromatase activity (.) has been induced in granulosa cells. Interactions between theca and granulosa cells, the former producing androgens (androstenedione: A), result in increasing estradiol (E2) and dihydrotestosterone (DHT) synthesis. D, FSH-dependent follicular growth results in more E2 synthesis. E, Estradiol enhances pituitary LH secretion in response to GnRH, while at the same time inhibiting pituitary FSH secretion. The increased LH induces more theca LH receptors and stimulates androgen production. Androgens serve as substrate for E2 formation and synergize with FSH to stimulate progesterone (P) secretion, initiating luteinization of granulosa cells. F, In the preovulatory follicle, FSH induces LH receptors on the granulosa cell—which completes luteinization of granulosa cells. Steroid secretion is augmented further. Then, increasing progesterone amplifies the positive feedback effect of E2 to initiate the preovulatory gonadotropin surge.
Primordial follicle growth and development is gonadotropin independent. Subsequently, granulosa cells of preantral follicles develop FSH receptors, and theca cells, which encircle granulosa cells, develop LH receptors (Figure 15-15A). Activin causes FSH-independent up-regulation of FSH receptors in preantral follicles,111 although it opposes FSH stimulation of antral follicle development.31 Primordial follicle growth is constitutively repressed by nuclear forkhead transcription factor Foxo3; when Foxo3 is released in response to stimulation of the PTEN-PI3K-Akt pathway, follicular growth progresses to the point where follicles become responsive to FSH.133
Antrum formation requires a trace (prepubertal) amount of FSH receptor activation (Figure 15-15B).31,134–137 FSH stimulates androgen receptor expression in primary follicles, and androgens in turn stimulate further expression of FSH receptors and the early stages of follicular growth.138 Androgen action is also necessary for the development of a full complement of follicles, and androgen excess stimulates excessive follicle number.139,140 Luteinizing hormone stimulates the appearance in thecal cells of the enzymes necessary for androgen biosynthesis.141 Evidence that theca cells of small antral follicles form E2 is meager.142
As antral follicles grow over 2.5 mm diameter, their granulosa cells begin to form E2 from androgen supplied by theca cells (Figure 15-15C).143–147 Androgen production at low levels may synergize with FSH to stimulate aromatase activity within the granulosa cells.107,148,149
At this stage, follicles are increasingly FSH dependent and are consequently uniformly FSH responsive.31,134 IGF-1 is required for follicular growth beyond the early antral stage in response to FSH.150 Antral follicles do not grow over 5 mm in diameter without a pubertal degree of FSH stimulation.136 By the midfollicular phase, the proliferation of FSH-responsive granulosa cells results in an accelerating rate of E2 production and preferential conversion of androstenedione to E2 rather than DHT by these cells (Figure 15-l5D).143–145,147,151,152 Estradiol itself clearly stimulates proliferation of granulosa cells and oocyte survival in rodents.153 In humans, E2 appears to promote antral growth independently of LH154 and is synergistic with FSH in bringing about the development of the dominant follicle.155,156
A dominant follicle is selected at the beginning of the menstrual cycle from a crop of follicles that were recruited 2.5 months prior.31 Recruitment of a group of follicles is normally promoted by the midcycle FSH surge and regresses with increasing corpus luteum progesterone secretion. Another wave of follicle growth in the late luteal phase is promoted by the rise of FSH as luteal progesterone and E2 secretion wanes. The selected follicle is the one that is the most sensitive to FSH (lowest “FSH threshold”). FSH is critically important during the follicular phase for optimal development of this dominant follicle. By the midfollicular phase of the cycle this follicle becomes virtually the sole source of E2 (Figure 15-15E). Typically, there is only one such follicle. Only this follicle continues to grow so as to reach a diameter of 10 mm or more. All other gonadotropin-dependent follicles undergo atresia.
At this stage the rising E2 level is suppressing FSH secretion and augmenting pituitary LH responsiveness to GnRH. FSH is more bioactive in the dominant follicle because it is more efficiently concentrated151 and because local factors increase ovarian responsiveness to FSH. The increased LH causes further proliferation of thecal cells and an increase in their LH receptor content.108 Consequently, androgen production increases. This synergizes with FSH to both augment aromatase activity and bring about increasing progesterone secretion by the well-estrogenized granulosa cells of these follicles. Progesterone then enhances the synthesis of both itself and E2.108,110 The increased thecal androstenedione production is diverted much more to E2 than to dihydrotestosterone biosynthesis. Antral fluid steroid concentrations reflect these changes (Figure 15-16).143,144,151 Activin acts so as to prevent premature luteinization of granulosa cells, and activin tone seems to wane as the preovulatory phase approaches.31,111
FIGURE 15-16 Normal human antral fluid steroid concentrations. Healthy follicles are well populated by granulosa cells (50% or more of maximal complement). Healthy follicles seem capable of further development because many of them (75%) contain healthy-appearing oocytes (histologically intact germinal vesicles), 96% of which are viable in culture. Moderately large follicles (8 mm or larger in diameter) make their appearance only in the midfollicular phase of the cycle and contain follicle-stimulating hormone. Data are shown only for those large follicles well populated by granulosa cells, only one of which usually arises in the follicular phase of each menstrual cycle. Atretic follicles are small follicles beginning to show degenerative changes in the number of granulosa cells and appearance of the oocyte. Cystic follicles tend to be larger follicles with only a sparse granulosa cell lining. The testosterone content of antral fluid is about a third that of dihydrotestosterone (DHT) owing to the pattern of granulosa cell metabolism of androstenedione (A). E2, estradiol; P, progesterone. (Interpolation based on the data of McNatty, K. P., Makris, A., Reinhold, V. N., et al. (1979). Steroids, 34, 429; McNatty, K. P., Smith, D. M., Makris, A., et al. (1979). The microenvironment of the human antral follicle: interrelationships among the steroid levels in antral fluid, the population of granulosa cells, and the status of the oocyte in vivo and in vitro. J Clin Endocrinol Metab, 49, 851.)
FSH next induces LH receptors in the granulosa cells (Figure 15-15F).110 These luteinized granulosa cells are capable of augmenting E2 and progestin production in response to LH as well as FSH.
The LH and FSH surge then occurs in response to the positive feedback action of E2 at both the CNS and pituitary levels, an effect amplified by the rising levels of progesterone. The final steps in follicle maturation ensue rapidly: the LH surge induces granulosa cell PR and prostaglandin synthase while inhibiting cyclin gene transcription,24,157 and the FSH surge up-regulates vascular endothelial growth factor.158 In the absence of these critical steps, ovulation and follicular rupture do not occur. Then the follicle promptly becomes desensitized to LH and FSH and ceases to grow.159 This is followed by an inflammatory-type response. Protease activity, prostaglandin production, and vascular permeability increase, cell junctions loosen, and cumulus cells form a mucopolysaccharide envelope around the oocyte (cumulus expansion).
Oocyte meiotic maturation resumes in response to a specific phosphodiesterase,160 forming the haploid gamete (secondary oocyte) and the first polar body in response to the LH surge.161 Ovulation of the cumulus-oocyte complex then occurs. The presence of a favorable follicular steroidal milieu is necessary both for ovulation (a premature LH surge in a subject with an unripe follicle will not result in ovulation) and subsequent developmental competence of the oocyte.162,163 Meiosis will complete and the second polar body will be extruded only in response to contact with a sperm.
The processes stimulating dominant follicle emergence are delicately balanced by those preventing it. It seems critical that the intraovarian concentration of androgens not become excessive or no follicles will remain viable beyond about the 8-mm stage.145 Androgen excess seems to prevent the emergence of dominant follicles by antagonizing granulosa cell proliferation and development.164 The mechanisms involved include inhibition of aromatase in situations of low FSH activity107,148 and antagonism of LH receptor formation and action.109,165 Follicles arrested in their growth become atretic, and atretic follicles contain relatively high concentrations of androgens (see Figure 15-16). Progesterone also suppresses further differentiation of nondominant follicles166 by some of the same mechanisms.167 High concentrations of estrogen play a critical role in inhibiting selection of the dominant follicles in primates.168 If there is interference with estrogenization, multiple large cystic follicles develop that are impaired in their ability to ovulate and undergo androgen-dependent atresia.169–171
AMH and inhibins have emerged as other follicular factors important in the direct and indirect regulation of follicular development. Granulosa cells of preantral and small antral follicles produce AMH, which regulates growth of follicles by exerting a paracrine negative feedback effect on the recruitment of precursor (primordial) follicles and inhibits aromatase activity.172,173 AMH levels do not fluctuate during the normal menstrual cycle174 but are indirectly inhibited by FSH during ovulation induction in response to estradiol produced by large antral follicles.175 AMH serum levels index the size of the oocyte pool (“ovarian reserve”) and gradually fall from young adulthood to become undetectable after menopause.176 Granulosa cells also produce inhibins, which are regulated by FSH in a negative feedback loop and up-regulate thecal steroidogenesis, as discussed later: inhibin-B is the predominant form of inhibin: inhibin-A is a product of the preovulatory follicle (and corpus luteum) that responds to both LH and FSH.177,178
Atresia is the fate of all except the few hundred follicles chosen for ovulation during an individual’s life span. Most follicles beyond the primordial stage become atretic. Atresia occurs by the process of programmed cell death.31 This apoptotic process has diverse determinants, including cell death inducer and repressor genes.39,132 FSH support becomes increasingly necessary for survival as the follicle matures, and normally only the follicle that has the lowest FSH threshold escapes atresia.
Luteal (secretory) phase ovary.
Histologically, luteinization is a process of lipid accumulation and begins as the preovulatory follicle forms. The biochemical hallmark of luteinization is the capacity for progesterone biosynthesis in response to LH; this is accompanied by increased secretion of estrogen and 17-hydroxyprogesterone in man.179–181 Following ovulatory rupture of the Graafian follicle, capillaries and fibroblasts from the theca proliferate and break down the separating basement membrane. The luteinized granulosa and theca cells then intermingle and complete the luteinization process by forming the corpus luteum.182
During its functional life span, the corpus luteum is normally the major source of the sex hormones secreted by the ovary. Corpus luteum function reaches its peak about 4 days after ovulation and begins to wane about 4 days before menstruation (Figure 15-14H). Loss of sensitivity to LH and estradiol heralds luteal senescence. Regression of the corpus luteum—luteolysis—occurs if pregnancy does not provide hCG. Luteolysis is probably mediated by prostaglandin. Transformation of the corpus luteum into an avascular scar, the corpus albicans, then occurs.
Early luteal phase increases in secretion of both E2 and progesterone cause secretory transformation and hyperplasia of the endometrium. A significant rise in basal body temperature, averaging 0.35° C, occurs when serum progesterone reaches an average of 400 ng/dL and continues as long as that level is maintained.183 Later fall-off in secretion of female hormones to a level insufficient to maintain the endometrium results in menstruation. Withdrawal of progesterone is specifically responsible for constriction of spiral arteries, local prostaglandin accumulation, and subsequent ischemic necrosis of the endometrium. Normal menstrual flow then results from a complete slough of the secretory endometrium.
A major determinant of normal corpus luteum formation and function is optimal development of the corpus luteum predecessor, the dominant follicle. Experimental lowering of FSH levels in the early follicular phase has been shown to impair subsequent corpus luteum function.184
Regulation of the neuroendocrine-ovarian axis
Factors controlling the onset of puberty
Pubertal onset is under the control of a complex regulatory network that is able to dynamically respond to numerous endogenous and environmental signals. GnRH neurons play a critical hierarchical role in the direct and indirect integration of these central and peripheral signals. Reproductive development is coupled with metabolic cues that influence the maturational process. The mechanisms by which neuroendocrine and genetic factors control pubertal development remain unknown. Epidemiologic studies indicate that nutrition, ethnicity, and genetic factors are normally important in the pubertal process.185 Environmental chemicals and chronic inflammatory disease can disrupt the process.185–188
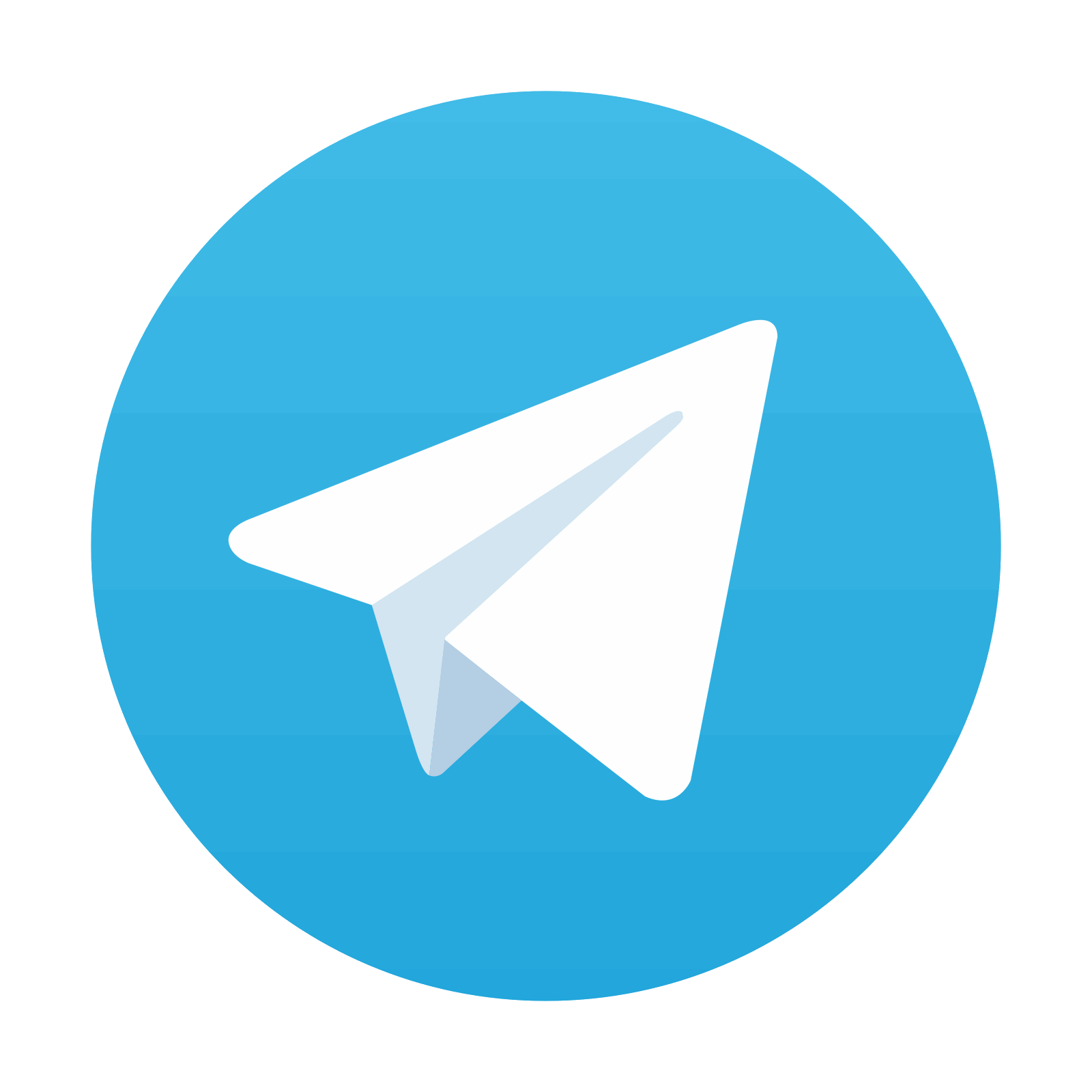
Stay updated, free articles. Join our Telegram channel

Full access? Get Clinical Tree
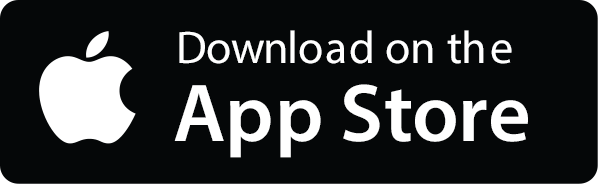
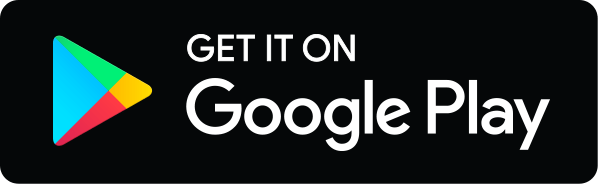