Introduction
Numerous planning studies demonstrate superior dosimetry for proton radiotherapy compared with photon-based radiotherapy for breast cancers of all stages, even compared with intensity-modulated radiotherapy. Protons can reduce dose to the heart by a factor of two to three compared with well-designed photon-based three-dimensional conformal plans, and target coverage is improved with protons. An ongoing randomized clinical trial is comparing proton therapy to photon therapy to examine the effect on cardiac toxicity (NCT02603341). To date, however, clinical outcome data comparing protons to photons for breast cancer are limited, so translation of this dosimetric advantage remains under investigation, and access and cost may offset the obvious dosimetric gains in some cases. Reports of several hundred patients treated with protons have been published, and these highlight patient satisfaction and good early clinical outcomes in most studies but increased skin toxicity and other side effects in others. , A broad range of dose regimens has been used, adding complexity to the analysis of the emerging data ( Table 8.1 ). Here we briefly review the available data on dosimetric, clinical, and value related to proton therapy for breast cancer, highlight cases for whom there is an obvious benefit in the context of the existing data, and touch on planning issues to optimize outcomes. For all plan reviews, plans should be evaluated by physics before review by the radiation oncologist. Physics sign-off should confirm that the plan is robust. When multiple passive fields are used, each beam should be viewed independent of the others for complete coverage. For multiple-field-scanning beam planning, robust optimization, discussed below, should be used before physician review.
Publication | n | Median FU (Months) | Gy (RBE)/# Fractions | IBTRFS | Early Skin Toxicity ( n ) | Late Skin Toxicity | Good-Excellent Cosmesis |
---|---|---|---|---|---|---|---|
APBI | |||||||
Bush et al. | 100 | 60 | 40/10 once a day | 97% (5 years) | 0 MD | 7% grade 1 telangiectasia | 90% |
Chang et al. | 30 | 59 | 30/5 once a day | 100% (5 years) | 1 MD | 69% (at 3 years) | |
Galland-Girodet et al. | 19 | 82.5 | 32/8 twice a day | 89% (7 years) | NR | 54%–69% late skin toxicities | 62% (MD at 7 years); 92% (PRO) |
MDACC a | 113 | 36 | 34/10 twice a day | 100% (3 years) | 0 MD | 23% telangiectasia | NR |
LABC | grade 2 esophagitis | ||||||
Cuaron et al. | 30 | 6 | 50.4/28 | NA | 8 MD | n = 8 | 1 grade 3 reconstructive complication |
MacDonald et al. | 12 | 2 | 50.4/28 (nodal dose 45) | NA | 9 grade 2 skin | n = 0 | NA |
Mutter et al. | 12 | 10 | 50/25 | NA | 1 MD, 5 grade 2 skin | n = 2 | 1 small dehiscence |
Verma et al. | 91 | 15.5 | 50.4/28 + 10/5 boost | NA | ∼7 treatment break | n = ∼30 | NA |
Bradley et al. | 18 | 20 | 50.4/28 + 10/5–16/8 boost | NA | ∼4 grade 3 skin tox | n = 5 | NA |
Complex geometry/locally advanced breast cancer
A commonly spoken misconception about radiotherapy for breast cancer is that it is easy. Some of it is. When it isn’t easy, though, breast cancer can present a vast array of unique and complex scenarios that arise from a combination of body habitus, disease factors, and surgical or systemic therapy choices that make tried-and-true breast radiation techniques suboptimal. Regional nodal irradiation (RNI; infraclavicular, supraclavicular, and internal mammary nodal basin coverage) is a primary driver of both lung and cardiac dose. Although these nodal basins can be treated within normal tissue constraints for many patients by using photon electron combinations, meeting the target goals and constraints can be impossible with photon- and electron-based therapy for patients where (1) depth to the internal mammary nodes is large, (2) involved nodal coverage requires significant coverage of internal mammary spaces directly anterior to the heart, or (3) a significant proportion of the overall lung volume lies in the supraclavicular field. Importantly, a meta-analysis of two randomized trials examining the benefits of RNI demonstrated increased overall survival in RNI-treated patients. In complex geometry cases where RNI is warranted, the radiation oncologist often must compromise on the goals, perhaps using intensity-modulated radiation therapy and accepting a higher heart and lung dose or lowering the dose to the target to achieve safe constraints. Numerous dosimetric studies demonstrate that these challenges can be overcome for patients with complex anatomy by using proton therapy. , , , Efforts to create objective indications for patients for whom geometric challenges merit proton therapy have been published, including the work by Mailhot Vega et al. demonstrating value for all patients with one or more cardiac risk factors for whom the mean heart dose would otherwise be greater than 5 Gy. This recommendation is based on clear demonstration that protons can reduce left anterior descending coronary artery dose and mean heart dose. The ongoing randomized photon versus proton RadComp trial will determine if improvements in dosimetry from using proton therapy will improve clinical cardiac toxicity from breast radiotherapy among unselected patients receiving RNI (NCT02603341).
Postmastectomy radiation planning
Planning begins with obtaining planning computed tomography (CT) images, paying careful attention to setup and immobilization including head position. , , , The physician draws the clinical target volume (CTV), which the physicist edits to create a scanning target volume (STV). The STV excludes 5 mm for the skin rind and includes a distal margin on the CTV calculated based on the range (∼3.5%). Reducing the distal margin can avoid overshooting into the lung because the chest wall thickness for those patients is rather small (∼5 mm), but it must be balanced with the concern that an adequate dose is delivered to the tissues immediately anterior to the chest wall, and in some cases, the chest wall itself. In most cases, photon/electron treatments of locally advanced postmastectomy targets would fully cover the chest wall in the target, and this should be considered in the patient selection and planning process.
To avoid tangential beam entrance, an en face beam is preferred, and thus often only one beam with one ideal angle is needed. This is achieved by a 30-degree beam from the anteroposterior direction, with ±10-degree variation, depending on the patient’s setup. Given the field size limit of 30 cm, in some cases, the full volume cannot be encompassed in one field, and a two-isocenter plan is used with two junctions during the course of treatment. In this case, field-specific targets may be created, and each field would have at least a 4-cm dosimetric overlap to have a smooth transition at the junction of both beams.
Robust optimization should be performed to ensure that coverage is not overly affected by variation in setup and anatomy. If a single-field plan is used, robust optimization may have little benefit.
Silicone implants
Implants are a major driver of complex geometry in patients with locally advanced breast cancer. We have demonstrated that implants and reconstruction can compromise optimal electron/photon plans. This could be overcome with the dosimetric advantages of protons. Because the silicone material is very uniform and the range prediction is very good, the planning is very similar to that for the mastectomy case discussed earlier, taking a correction for the stopping power of silicone into account.
To determine this correction, we tested three different silicone types (smooth, rough surface, and profiles) from two different vendors (Mentor and Allergan). The first step was to determine the water-equivalent range of the different types of tissue expanders (TEs). The silicone material stopping power does not lie on the CT calibration curve (Hounsfield unit [HU]-to-stopping power conversion curve) for human tissue because of its rather high Z number (14) compared with human tissue, which has an average Z-number of 7. Thus its HU value in CT is overestimated, and the HU value must be overridden to avoid underranging the proton beam and thus missing the distal target.
To obtain the HU value, we scanned all implants by using a Siemens Definition Edge CT scanner and its clinical CT protocol used for patient treatment planning CT scans. The devices were positioned along the CT’s z -axis in such a way that they do not overlap and thus do not cause any attenuation artifacts. The material properties and the measured HU numbers are shown in Table 8.2 . The overestimated relative stopping powers based on HU values are shown in the last column.
Surface | Shape | Volume (cc) | Measured Hounsfield (HU) | Estimated. Relative Stopping Power | |
Rough | Teardrop | 445 | 92 ± 3 | 1.0828 | |
Mentor | Smooth | Round, high profile | 650 | 90 ± 4 | 1.0811 |
Allergan Naturelle | Smooth | Full round | 445 | 93 ± 3 | 1.0836 |
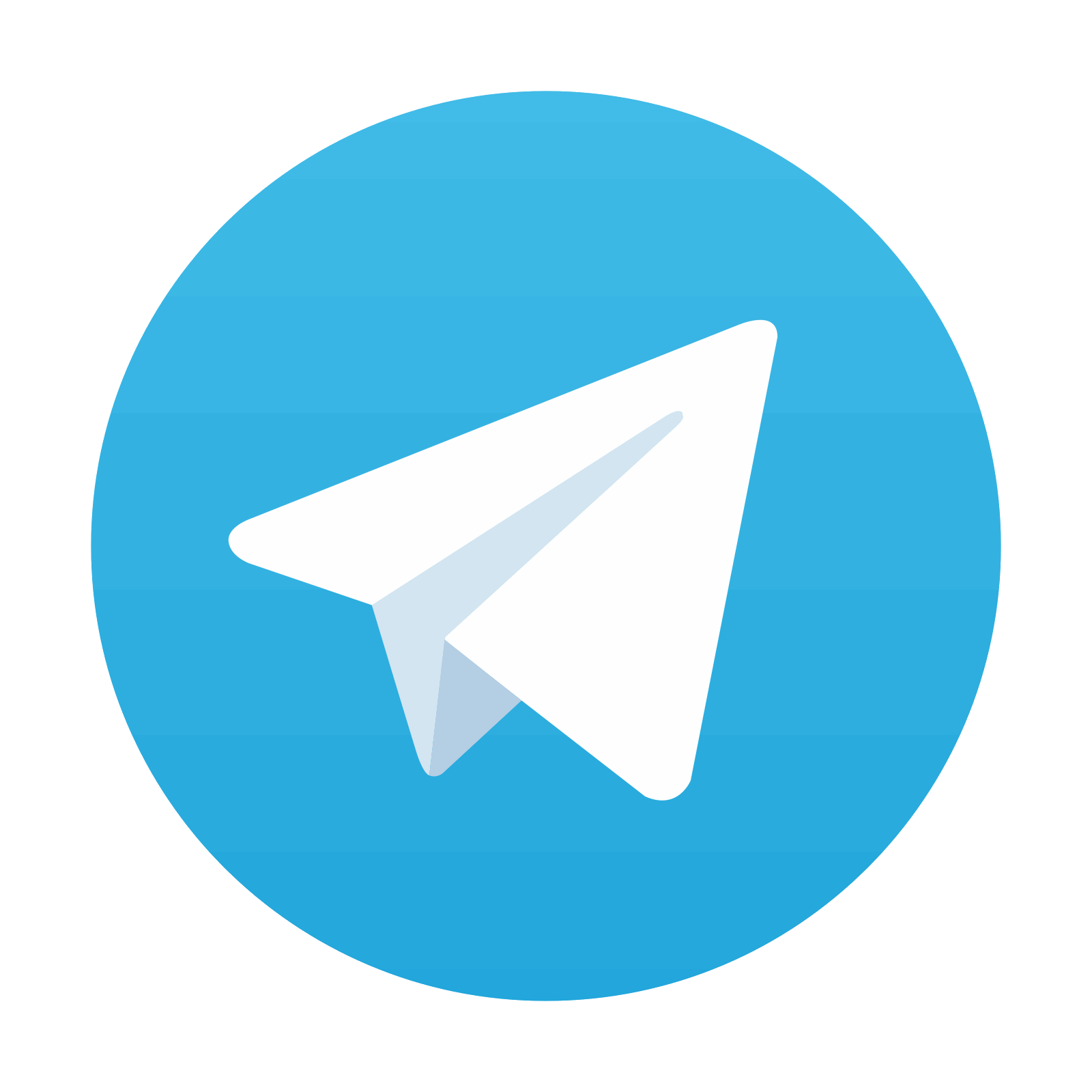
Stay updated, free articles. Join our Telegram channel

Full access? Get Clinical Tree
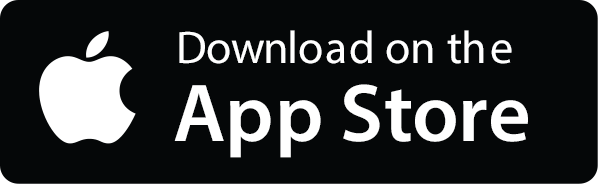
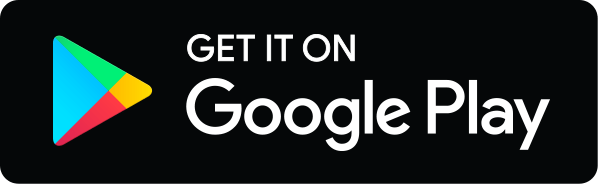
