of PAR-1 when bound to EPCR and possibly other receptors.16 Additional dimensions to studies on the protein C pathway include preclinical studies showing beneficial effects of second-generation recombinant APC mutants in sepsis and ischemic stroke, the generation of protein C- or protein S-deficient mice, and the findings that protein S can exert direct receptor-mediated effects on cells.16,17,18,19,20,21,22,23,25,27,28,29,30,31,32,33,34,35,36,37,38,39,40,41,42,43,44,45,46,47
Thrombin cleavage of the zymogen at Arg169 removes the activation peptide and generates APC, a trypsin-like serine protease with a typical serine protease active site triad (His211, Asp257, and Ser360). Endogenous circulating levels of plasma APC are low (<40 pM), and inactivation of APC in plasma is driven by serine protease inhibitors (SERPINs) that contribute to a remarkably long circulation half-life of APC in man of approximately 20 to 25 minutes. Most important inhibitors of APC in plasma are protein C inhibitor (PCI) and α1-antitrypsin and, to a lesser extent, α2-macroglobulin and α2-antiplasmin.
Table 19.1 Characteristics of the protein C pathway components | ||||||||||||||||||||||||||||||||||||||||||||||||||||||||||||||||||||||||||||||||||||||||||||||||||||||||||||||||||||||||||||||
---|---|---|---|---|---|---|---|---|---|---|---|---|---|---|---|---|---|---|---|---|---|---|---|---|---|---|---|---|---|---|---|---|---|---|---|---|---|---|---|---|---|---|---|---|---|---|---|---|---|---|---|---|---|---|---|---|---|---|---|---|---|---|---|---|---|---|---|---|---|---|---|---|---|---|---|---|---|---|---|---|---|---|---|---|---|---|---|---|---|---|---|---|---|---|---|---|---|---|---|---|---|---|---|---|---|---|---|---|---|---|---|---|---|---|---|---|---|---|---|---|---|---|---|---|---|---|
|
the liver but is also made by endothelium and other cells. Protein S circulates in plasma at a total concentration of 320 nM, and approximately 60% of the protein S is noncovalently complexed with C4b binding protein (C4BP) such that free protein S is 130 nM in plasma.56 C4BP is an octopus-like shaped glycoprotein of approximately 500 to 570 kDa and consists of six or seven disulfide-linked, identical alpha-chains and one smaller beta-chain (see Table 19.1).57 C4BP regulates protein S functions by binding via the beta-chain.58 Protein S binds to the C4BP beta-chain in a 1:1 stoichiometric complex with high affinity (Kd ˜ 0.1 to 0.6 nM).59 Although C4BP is an acute-phase response protein, only alpha-chains are increased causing the levels of free protein S levels to remain stable during an acute-phase response.60
the form of a 60.3-kDa single-chain transmembrane protein.63 Posttranslational O-linked and N-linked glycosylation accounts for approximately 20% of the apparent molecular weight of TM, and other modifications include covalently bound chondroitin sulfate moieties.64
![]() FIGURE 19.4 Amino acid sequence and ribbon polypeptide scheme of the EPCR. Amino acids are numbered from the amino-terminus of the mature protein with the signal peptide sequence underlined. Specific domains are color coded as indicated in the ribbon cartoon. Black circles represent sites of N-linked glycosylation. EPCR residues implicated in binding of APC are indicated in red.54 |
is located along opposing edges of a planar platform generated by an eight-stranded antiparallel beta-sheet. The crystal structure of soluble EPCR revealed that a phospholipid, most likely phosphatidylcholine, was bound in this groove between the two alpha-helices.69,70 EPCR’s short cytoplasmic tail (Arg-Arg-Cys-CO2H) suggests that induction of direct cell signaling is unlikely and that palmitoylation of the C-terminal Cys residue may help localize EPCR to certain lipid rafts or caveolae and facilitate modulation of cell-signaling pathways that depend on EPCR.71,72
is strongly linked to excessive complement activation.143 The lectin-like domain of TM (figure 19.3) can contribute to inhibition of complement activation and provide direct anti-inflammatory activity, although the molecular basis for this remains somewhat unclear.144,145,146,147
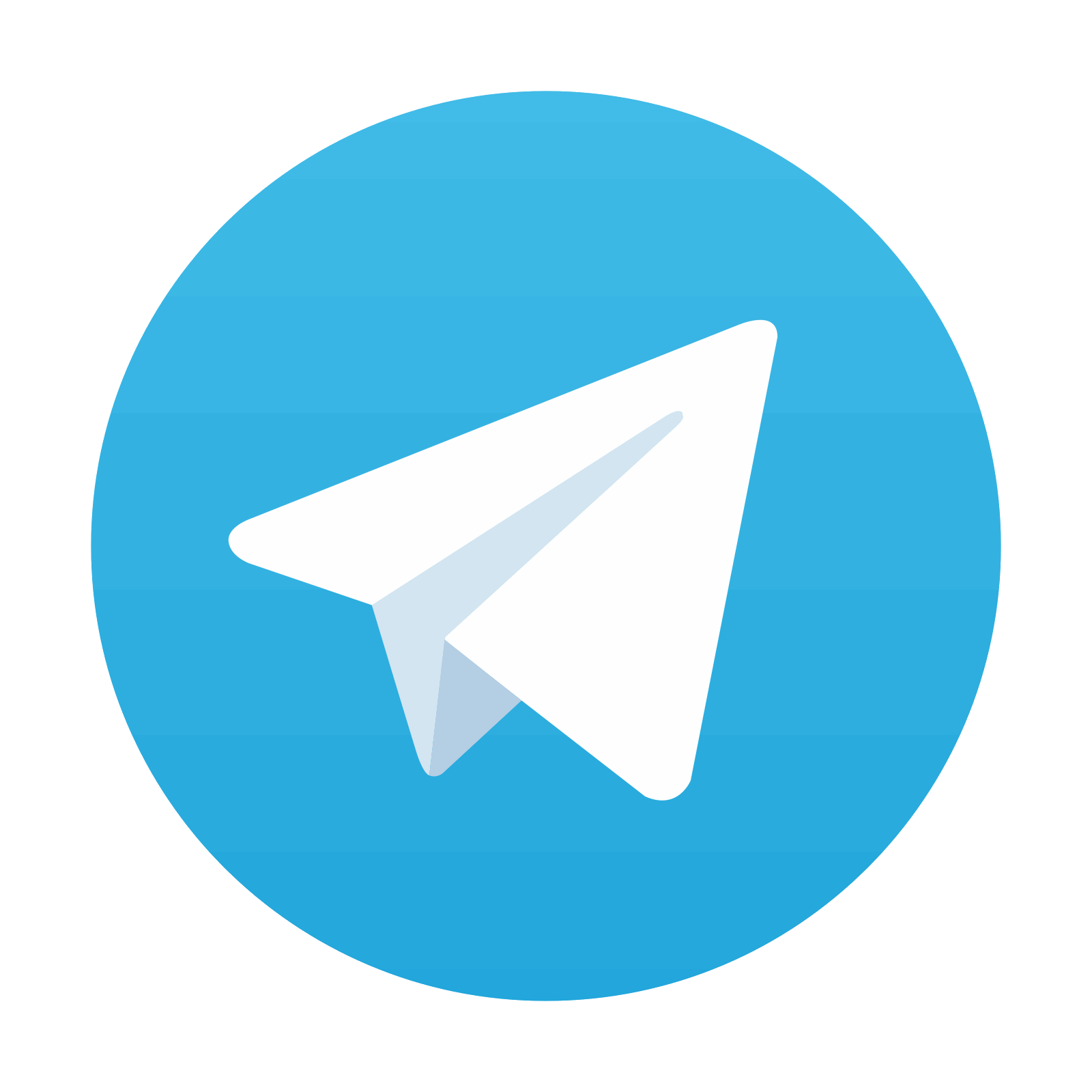
Stay updated, free articles. Join our Telegram channel

Full access? Get Clinical Tree
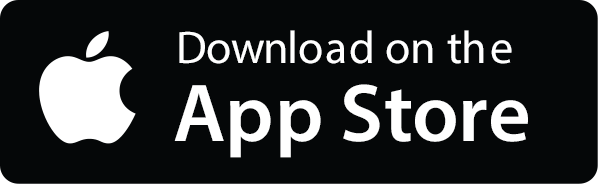
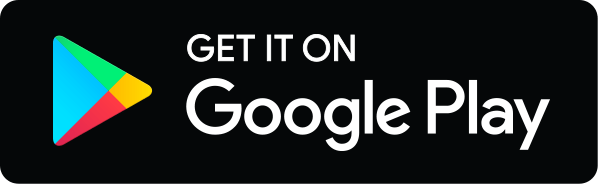