Abstract
Prolactin is a single chain polypeptide secreted by anterior pituitary lactotroph cells. Prolactin secretion is primarily under inhibitory control by hypothalamic dopamine. Prolactin levels rise substantially during pregnancy and lactation and serve an important role in preparing the breast for lactation.
Prolactin excess can be caused by a variety of etiologies, both physiologic and pathologic. The latter include medications, pituitary disease, and systemic causes, or it can be idiopathic. Hyperprolactinemia often leads to hypogonadism and infertility. Prolactin deficiency is uncommon and causes failure of lactation postpartum.
Prolactin-secreting pituitary adenomas are common, benign neoplasms that may present with mass effect, hypopituitarism, and the effects of hyperprolactinemia itself. Dopamine agonist therapy is the mainstay of treatment for prolactinomas, whereas surgery and radiation therapy have important therapeutic roles in very selected cases.
Keywords
Adenoma, bromocriptine, cabergoline, dopamine agonist, hyperprolactinemia, pituitary, prolactin
Prolactin (PRL) is a single chain (23-kDa) polypeptide hormone that is secreted by anterior pituitary lactotroph cells. Several lines of evidence indicate that PRL has an essential role in reproduction and lactation. In addition, animal data have supported a role for PRL in a variety of metabolic processes. However, such PRL actions have not been unequivocally confirmed in humans. The present chapter reviews PRL physiology, followed by a discussion of the role of PRL in pathologic states, including hyperprolactinemia and PRL deficiency. Data on the epidemiology, pathology, clinical evaluation, and management of PRL-secreting pituitary adenomas (prolactinomas) are then reviewed, including data relevant to prolactinomas in the setting of preconception and pregnancy.
Lactotroph Development
- ◆
Lactotroph cells develop under carefully orchestrated control by several transcription factors.
- ◆
Lactotroph hyperplasia is physiologic during pregnancy and is reversible postpartum.
Pituitary lactotrophs are relatively abundant in the human anterior pituitary gland, accounting for up to 25% of cells in individuals of both genders. During embryogenesis, the pituitary gland develops from ectodermal primordial cells, destined to form the anterior and intermediate lobe and neuroectodermal tissue arising from the floor of the diencephalon, which ultimately forms the posterior lobe. During development, inductive interactions and a host of transcription factors are critical in the formation of the pituitary and differentiation into mature functioning cells. Transcription factors that have been implicated in pituitary ontogenesis include SIX3, HESX1, LHX3, LHX4, SOX2, SOX3, PITX2, OTX2, BMP2, BMP4, and GLI2.
Lactotroph and somatotroph cells generally develop from common progenitor cells (mammosomatotrophs) under the influence of several transcription factors, though it is possible that some lactotrophs may develop through other precursor cell lines. In particular, the transcription factor POU1F1 (also known as PIT1), a member of the POU homeodomain transcription factor family, is critical in the differentiation and proliferation of lactotrophs, somatotrophs, and thyrotrophs, and the expression of genes encoding PRL, growth hormone (GH), and the beta subunit of thyrotropin (TSH beta). Patients with inactivating mutations in the POU1F1 gene lack lactotrophs, somatotrophs, and thyrotrophs, accounting for the presence of PRL, GH, and thyrotropin deficiency, respectively. Another homeodomain transcription factor, known as prophet of PIT1 (PROP1), has a critical role in the expression of POU1F1. Patients with inactivating mutations in the PROP1 gene may have PRL, GH, and thyrotropin deficiency, as well as gonadotropin (follicle stimulating hormone [FSH] and luteinizing hormone [LH]) deficiency. Patients with inactivating mutations in genes encoding other transcription factors, including HESX1, LHX3, and LHX4, generally have multiple pituitary hormone deficiencies as well as other midline cranial defects.
Lactotroph hyperplasia is physiologic during pregnancy and is reversible within several months after delivery, but is delayed by nursing.
Prolactin Gene
- ◆
A single gene encodes prolactin in humans.
- ◆
Prolactin gene transcription is increased by estradiol and inhibited by thyroid hormone.
- ◆
Dopamine (DA), the major factor regulating prolactin secretion in humans, acts by inhibiting adenyl cyclase–dependent signaling pathways.
In humans, the gene encoding PRL consists of five coding exons, one noncoding exon, and four introns. It spans approximately 10 kb in length and is located on chromosome 6. There are several regulatory elements located in the 5′ region of the PRL gene, including areas responsible for stimulation of PRL gene transcription in response to POU1F1 or estradiol, as well as those responsible for suppression of PRL gene transcription by thyroid hormone. In addition to its direct effects, estradiol also modulates DA-induced inhibition on PRL gene transcription. The stimulatory effects of POU1F1 on PRL gene transcription are also subject to modulation by a variety of factors, including cyclic adenosine monophosphate (cAMP), glucocorticoids, estradiol, thyrotropin releasing hormone (TRH), and epidermal growth factor (EGF).
DA is the major inhibitory factor regulating PRL secretion and acts through the D2 dopamine receptor to inhibit adenyl cyclase and the cAMP-dependent protein kinase A (PKA) pathway. In contrast, TRH stimulates PRL secretion via the phosphoinositide pathway, leading to activation of membrane calcium channels and the release of calcium ions from the endoplasmic reticulum into the cytoplasm, which in turn activates protein kinase C (PKC), causing the downstream phosphorylation of other proteins, and also binds to calmodulin or topoisomerase II, which have a variety of downstream actions. Of note, DA inhibits PRL secretion caused by intracellular calcium ion release. This DA action is antagonized by several calcium channel antagonists in vitro, including verapamil, diltiazem, and nimodipine. Interestingly, verapamil causes the opposite effect from what would be predicted by in vitro data—that is, it leads to increase in PRL levels in humans. This observation was associated with decreased tuberoinfundibular DA release and may likely involve an effect of verapamil on N-calcium channels present in neurons. Other types of calcium channel antagonists, including dihydropyridines and benzothiazepines, do not affect PRL levels in vivo.
Vasoactive intestinal peptide (VIP) stimulates adenyl cyclase, leading to PRL secretion. Several factors that stimulate PRL secretion, including TRH, neurotensin, and angiotensin II, may also act via the stimulation of phospholipase A2, leading to the release of arachidonic acid, which causes an increase in calcium influx. This effect can be antagonized by DA and phospholipase A2 inhibitors.
Prolactin Synthesis in Pituitary Lactotrophs
- ◆
Several posttranslational prolactin modifications occur and influence prolactin bioactivity.
- ◆
Macroprolactin species are large prolactin aggregates that have decreased bioactivity.
- ◆
A 16-kDa prolactin fragment has been implicated in the pathogenesis of peripartum cardiomyopathy and preeclampsia.
The PRL gene is transcribed to mRNA, which undergoes processing in the nucleus to yield a mature, 1 kb mRNA species, encoding a 227-amino acid PRL precursor. This contains a 28-signal peptide sequence, which is cleaved posttranslationally to yield a 199-amino acid PRL protein. Additional posttranslational modifications of the PRL molecule include glycosylation, phosphorylation, cleavage, and polymerization.
Of note, the large majority (80% to 90%) of the circulating PRL is monomeric, whereas about 10% of circulating PRL is dimeric (“big PRL,” molecular mass ~50 kDa) and approximately 5% of circulating PRL is multimeric (“big big PRL”). Such high molecular mass PRL species are collectively called “macroprolactin” and may additionally contain bound immunoglobulin. Macroprolactin has been found to exhibit decreased binding to PRL receptors and has decreased receptor binding affinity and decreased bioactivity in most, but not all, assays. Patients with macroprolactinemia, who have elevated total serum PRL as a consequence of elevated multimeric PRL, appear to have normal pituitary gonadal function, likely as a consequence of decreased bioactivity of multimeric PRL species.
Cleavage of the 23-kDa PRL species by metalloproteases or cathepsin D may occur in peripheral tissues, leading to the generation of an N-terminal 16-kDa PRL variant. This PRL species has antiangiogenic, proapoptotic, and proinflammatory properties, and has also been implicated in the pathogenesis of peripartum cardiomyopathy and preeclampsia, based on animal and human data.
Prolactin Synthesis in the Decidua and Other Tissues
- ◆
Extrapituitary prolactin secretion occurs in the decidua and other tissues.
- ◆
Prolactin of decidual origin appears to promote tolerance of the fetus in utero.
- ◆
The physiologic role of extrapituitary prolactin in other tissues remains poorly understood in humans.
Several lines of evidence suggest that PRL is synthesized in the decidua. Very high PRL levels (10 to 100 times those in maternal serum) have been found in amniotic fluid. In culture, decidual and chorion cells secrete PRL. This PRL species is identical in sequence and activity to pituitary PRL and is expressed under control by an alternative promoter, which is located upstream from the transcription initiation site used in pituitary lactotrophs. Of note, PRL secretion in the decidua is stimulated by progesterone (either alone or together with estrogen), relaxin, insulin, and insulin-like growth factor 1 (IGF-1), but is not influenced by DA agonists or antagonists (in contrast to pituitary PRL). Decidual PRL appears to have an important role in maintaining pregnancy by downregulating interleukin 6 and 20 alpha hydroxysteroid dehydrogenase. Of note, decidual PRL synthesis was reduced in decidual tissue from women who had suffered a miscarriage, wherein proinflammatory cytokines were elevated. In aggregate, these data suggest that decidual PRL supports pregnancy by promoting tolerance of the fetus in utero.
Extrapituitary PRL expression has also been documented in the mammary gland, ovaries, testes, prostate, endothelial cells, brain, skin, adipose tissue, lymphocytes, and cochlea in a variety of animal paradigms. The physiologic role of extrapituitary PRL synthesis is under investigation. It has also been proposed that extrapituitary PRL may promote tumorigenesis (including tumor initiation and/or propagation) in the breast and prostate. This is an area of ongoing debate and uncertainty, since some studies have found little PRL expression in human tumors. Gain of function PRL receptor variants do not appear to be associated with higher risk of breast cancer or fibroadenomas. Nevertheless, available data have prompted the development of a variety of inhibitors of PRL receptors or PRL signaling, and their study, as potential therapies in human prostate or breast cancer.
Prolactin Assays
- ◆
Prolactin is commonly measured using two site antibody immunoassays.
- ◆
Several artifacts and conditions may influence the results of these assays, including the hook effect, the presence of macroprolactin, heterophilic antibodies, and biotin.
Serum PRL is currently measured using two site (“sandwich”) immunometric assays, including immunoradiometric (IRMA), chemiluminescent (ICMA), and electrochemiluminescent (ECLIA) platforms, which use a dual-antibody system. A “capture” antibody is used to collect the PRL molecules present in the specimen to a solid substrate (such as microparticle beads or coated tubes). Subsequently, a second (“reporter”) antibody attaches to the PRL—capture antibody complex—and is used to generate a detection signal through its radiolabeled or chemiluminescent tag. Measured PRL levels can vary considerably between different immunoassays. Assay interference may occur and should be suspected in the presence of nonlinearity in serially diluted specimens or discrepancy between measured hormone levels and the clinical presentation. Several factors and mechanisms, including the “hook effect,” the presence of macroprolactin, heterophilic antibodies, or exogenous biotin, may lead to assay interference.
Hook Effect
The hook effect may occur when serum specimens being assayed contain very high PRL levels, as can be the case in patients with large PRL-secreting pituitary macroadenomas. In these patients, PRL is present in vast stoichiometric excess to the two assay antibodies in the test solution, thus preventing the formation of the heterotrimeric complex (capture antibody—PRL—reporter antibody). The hook effect can cause substantial under-reporting of PRL levels. This artifact is clinically significant, since patients with adenomas that are thought to be PRL-secreting will be generally offered a trial of medical therapy. In contrast, failure to diagnose a prolactinoma based on a normal or low prolactin level in a patient with a large pituitary adenoma may likely lead to a recommendation for surgery rather than medical therapy. In one study, the hook effect was reported in 5.6% of 69 patients with macroadenomas. The frequency of hook effect using current assay techniques is uncertain. To detect this possible artifact, PRL measurements should be obtained both in undiluted and diluted (1 : 100) serum specimens in patients with large macroadenomas.
Macroprolactin
Monomeric PRL normally accounts for approximately 90% of circulating PRL, the rest consisting of PRL multimers of varying molecular mass, sometimes associated with immunoglobulin. Multimeric PRL species are collectively termed macroprolactin (as previously noted). Macroprolactin can be resolved and separated from monomeric PRL by gel filtration. In routine practice, polyethylene glycol (PEG) precipitation has been used to separate macroprolactin from PRL monomers (only the latter is left in the supernatant after centrifugation). Of note, hyperprolactinemia can be solely attributed to the presence of macroprolactin, only if the monomeric PRL level present in the serum is within the reference range.
Macroprolactin appears to have decreased bioactivity in some, but not all, bioassays. Women with macroprolactinemia who were treated with DA agonists were reported to experience resolution of galactorrhea (if originally present) but had no consistent improvement in menstrual cyclicity. Macroprolactinemia should be considered in patients with hyperprolactinemia who lack typical symptoms attributable to PRL excess. However, the presence of macroprolactinemia does not obviate the need for pituitary imaging, since some patients with pituitary adenomas may exhibit macroprolactinemia. Therefore the clinical utility of assessing macroprolactin in symptomatic patients is limited.
Heterophilic Antibodies
Heterophilic antibodies, including human antimouse or other antianimal immunoglobulins and the rheumatoid factor autoantibody, may cause assay interference by bridging the capture and reporter antibodies in the absence of analyte (i.e., PRL), thus giving rise to artifactually high PRL levels. Heterophilic antibodies may also confound many other assays, in addition to PRL immunoassays. Using blocking animal sera or heterophilic antibody blocking tubes can help prevent this type of interference.
Exogenous Biotin
Several immunoassay platforms use biotinylated capture antibodies that collect the analyte being assayed onto streptavidin-coated microbeads. Measurement of hormone levels in patients taking biotin supplements in large doses is subject to possible interference, if the immunoassay platform in use employs the biotin-streptavidin reaction. In these cases, ingested biotin may prevent the formation of the streptavidin-biotin complex in the assay solution, leading to under-reporting of the analyte being measured by sandwich immunoassays (including PRL) or overreporting of hormones measured using competition assays.
Prolactin Secretion
- ◆
PRL secretion is pulsatile.
- ◆
Serum PRL levels rise in many physiologic states, including pregnancy and lactation.
PRL secretion is pulsatile, involving approximately 14 secretory peaks per 24 hours, each peak lasting 67 to 76 minutes. The PRL pulse amplitude increases during slow wave sleep, with pulses beginning about 60 minutes after sleep onset. In addition, PRL levels increase by 50% to 100% about 30 minutes after meals as a result of stimulation of PRL secretion by amino acids being absorbed postprandially (particularly tyrosine, phenylalanine, and glutamate).
Changes in Prolactin Levels With Age
Immediately following delivery, PRL levels in neonates are elevated about 10 times above baseline, presumably as a consequence of high levels of placental estrogen, and decrease toward baseline over several months. Subsequently, PRL levels rise somewhat during adolescence. In women, PRL levels decrease by approximately 50% within about 2 years after menopause. In older men, PRL levels also decline by about 50% in comparison with young adults.
Prolactin Levels During Physiologic Stress
Acutely, exercise leads to an increase in serum PRL levels, which is not sustained long term in long distance runners. Other forms of physiologic stress, such as acute illness or injury, also lead to a two- to threefold increase in PRL levels, lasting about 1 hour, which is not sustained in patients with prolonged illness. Women have a more robust prolactin response than men.
Prolactin Levels During the Menstrual Cycle
Prolactin levels are lower in the follicular phase and rise during the luteal phase in some women. In addition, PRL and LH secretion are synchronous in the luteal phase.
Prolactin Levels During Pregnancy and the Postpartum State
During pregnancy, PRL levels increase continuously and may reach 10-fold or higher levels over those in non-pregnant women. This is a consequence of estrogen secretion by the placenta, which leads to lactotroph hyperplasia and stimulates PRL secretion, preparing the breast for lactation postpartum.
After delivery, basal PRL levels remain elevated in women who are nursing. In addition, PRL secretory peaks occur rapidly at the time of each suckling event. Within several months, there is a gradual decline in basal PRL levels towards normal as well as a decrease in the amplitude of secretory spikes in response to suckling. These events occur as a consequence of a gradual decrease in intensity of breast-feeding, while formula is being introduced into the infant’s diet. Menses resume as postpartum hyperprolactinemia abates.
Nipple stimulation, either acute or chronic (by nipple rings), may cause hyperprolactinemia in some healthy women who are not nursing.
Regulation of Systemic Prolactin Levels
- ◆
PRL secretion is primarily under inhibitory control by hypothalamic DA.
- ◆
TRH, VIP, serotonin, and other factors may have some role in stimulating prolactin secretion.
Hypothalamic regulation of PRL secretion is primarily mediated via inhibitory factors (predominantly DA), as evidenced by an increase in PRL secretion and systemic PRL levels in patients who suffered pituitary stalk damage. In addition, several releasing factors may have a role in modulating PRL secretion ( Fig. 3.1 ).
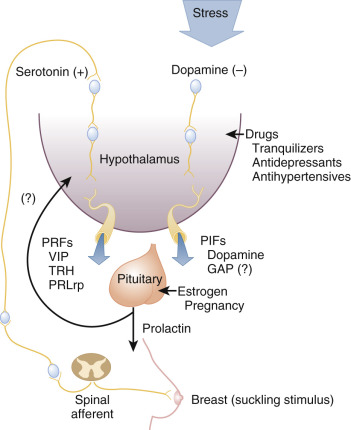
Prolactin Inhibitory Factors
Several lines of evidence indicate that hypothalamic DA is the most important PRL inhibitory factor under physiologic conditions. DA is present in hypothalamic portal vessels at levels that are sufficient to suppress PRL secretion. Stimuli that elicit PRL secretion also lead to a decrease in DA levels in the hypophyseal portal circulation. Mice with targeted disruption of D2 receptors, whose absence prevents DA action in pituitary lactotrophs, develop hyperprolactinemia, lactotroph hyperplasia, and multiple prolactinomas. Infusion of DA in low doses leads to suppression of PRL secretion in humans. Estradiol partially reverses the effects of DA infusion on PRL secretion. Pharmacologic agents that inhibit DA receptors lead to hyperprolactinemia in humans.
DA is synthesized in neurons whose perikarya are located in the dorsal arcuate nucleus and the ventromedial nucleus of the hypothalamus. This neuronal pathway is termed the tuberoinfundibular DA pathway . Axon terminals originating from these neurons terminate in the median eminence, where DA is released, enters the hypophyseal portal system, and traverses the pituitary stalk to reach pituitary lactotrophs in the pars distalis, where DA activates D2 receptors to suppress PRL secretion. Mice with disrupted PRL genes do not synthesize any PRL and have substantially decreased DA in the tuberoinfundibular DA pathway, consistent with the existence of a positive effect of secreted PRL on hypothalamic DA release, generating a short loop feedback mechanism of secreted PRL that negatively regulates its own secretion.
Whether other physiologically relevant PRL inhibitory factors exist is a matter of controversy. Some data suggest that gonadotropin releasing hormone (GnRH)–associated protein (GAP) as well as gamma-aminobutyric acid (GABA) can suppress PRL in experimental paradigms, but their relevance in humans is unclear.
Prolactin Releasing Factors
Several factors have been proposed as being stimulatory of PRL secretion (as detailed later). However, their physiologic relevance is uncertain in many cases. Overall, their role appears relatively minor in comparison with the inhibitory role of DA on PRL secretion.
Thyrotropin Releasing Hormone
TRH induces PRL secretion in vitro and in vivo in humans, when administered in doses that also cause TSH secretion. On the other hand, targeted disruption of the TRH gene in mice leads to central hypothyroidism but does not influence PRL levels. In addition, nipple stimulation during suckling leads to a PRL secretory spike but does not influence TSH levels. Thus it appears that TRH does not have a major role as a releasing factor under normal conditions. It should be noted, however, that patients with primary hypothyroidism have elevated TSH and PRL levels, which are normalized by levothyroxine replacement, suggesting that endogenous TRH may be relevant in PRL regulation in these patients, but does not exclude the possibility that changes in dopaminergic tone in the hypothyroid state may also contribute to hyperprolactinemia. Of note, patients with hyperthyroidism have normal PRL levels, but do exhibit an abnormally low PRL response to TRH administration, which is restored after euthyroidism is achieved.
Vasoactive Intestinal Peptide and Peptide Histidine Methionine
VIP and peptide histidine methionine are derived from posttranslational processing of the same precursor. Of note, VIP producing neurons have been localized to the paraventricular hypothalamic nucleus. In addition, VIP is expressed in the anterior pituitary. Administration of VIP induces PRL synthesis and secretion in vitro and in vivo in humans. In addition, VIP immunoneutralization or antagonism blocks PRL secretion in various paradigms. Administration of peptide histidine methionine has led to inconsistent results with regard to PRL secretion in humans. Overall, the physiologic role of VIP and peptide histidine methionine in the regulation of PRL secretion remains incompletely elucidated.
Serotonin
In experimental animals, administration of either serotonin or 5-hydroxytryptophan (serotonin precursor) induces PRL secretion. Inhibition of serotonin synthesis blunts PRL secretion in response to suckling. Similarly, intravenous administration of 5-hydroxytryptophan in humans triggers PRL secretion. Cyproheptadine, a serotonin receptor antagonist, inhibits both the nighttime PRL surge and the fenfluramine-induced increase in PRL levels. Administration of fluoxetine, a serotonin reuptake inhibitor, leads to a mild increase in PRL levels over baseline. Overall, these data suggest a possible role for serotonin in the regulation of nocturnal PRL secretion, as well as PRL release after nipple stimulation.
Opioids
In experimental animals, administration of opioid peptides results in an increase in PRL secretion, likely mediated via activation of the mu opioid receptor. It appears that the effect of opioids on PRL secretion is indirect and is mediated via a decrease in DA release in the tuberoinfundibular DA pathway.
In humans, opioid administration results in PRL secretion, both acutely and chronically. Of note, administration of naloxone, a mu opioid receptor antagonist, causes an increase in PRL levels during the late follicular and midluteal phase of the menstrual cycle in humans, but does not blunt PRL secretion in response to physiologic stress. In aggregate, available data suggest that endogenous opioids do not have a major physiologic role in the regulation of PRL secretion.
Other Neuropeptides and Neurotransmitters
Growth hormone releasing hormone (GHRH) administration triggers PRL secretion in healthy individuals and repeated GHRH administration leads to increased PRL levels in children with GH neurosecretory dysfunction. However, it is unclear if GHRH is physiologically relevant in the regulation of PRL secretion.
GnRH induces PRL secretion in vitro and in vivo in humans, including women with subfertility who are undergoing ovulation induction with gonadotropins. In addition, some healthy women secrete PRL in response to GnRH administration, with the highest proportion of responses noted in women around ovulation. Of note, GnRH administration does not induce PRL secretion in healthy men. In contrast, GnRH leads to PRL secretion in transgender men on estrogen therapy, suggesting that the estrogenic milieu may be essential for GnRH to induce PRL release.
Oxytocin administration triggers PRL secretion in vitro and in vivo in some experimental paradigms. In contrast, oxytocin alone does not appear to affect PRL secretion in humans. However, oxytocin administration has a minor positive effect on VIP-elicited PRL secretion in healthy individuals. There is no evidence that vasopressin stimulates PRL secretion.
Prolactin releasing peptide (PrRP) is expressed in neurons in the paraventricular and supraoptic nucleus of the hypothalamus. In vitro, PrRP stimulates PRL secretion. However, its physiologic role with regard to the regulation of PRL secretion has not been elucidated.
Prolactin Actions
- ◆
PRL exerts its actions by stimulating its receptor, a member of the cytokine receptor superfamily.
- ◆
PRL has major physiologic effects on the breast and possibly other tissues.
- ◆
PRL in excess causes hypogonadism, mediated primarily via hypothalamic actions but also effects on the ovaries and testes.
In humans, PRL has a well-established physiologic role on the breast during pregnancy and the postpartum period, wherein it is essential for lactation to occur after delivery. In addition, PRL in excess suppresses the activity of the hypothalamic-pituitary-gonadal axis in the nonpregnant state. In animals, PRL has been implicated in many additional roles, including growth and development, effects on beta cell function, electrolyte transport, osmoregulation, and effects on the skin and cartilage, in addition to effects on behavior, immune function, and tumorigenesis. None of these additional actions have been adequately substantiated in humans. It is likely that the physiologic role of PRL is species specific.
Prolactin Receptor
PRL exerts its actions by activating its cognate receptor, which is a member of the class 1 (hematopoietic) cytokine receptor superfamily. In humans, the PRLR gene is located on chromosome 5 and contains 10 exons, which yield several diverse receptor isoforms as a result of alternative splicing of the primary transcript. These are broadly grouped into long, intermediate, and short isoforms, most of which possess the same extracellular and transmembrane domains but differ in the length of the intracellular domain. There is also a soluble isoform, which lacks transmembrane and intracellular domains. The long PRL receptor isoform is predominant in humans and is widely expressed in many cell types. The extracellular domain contains two disulfide bridges that are critical for PRL binding. The intracellular domain contains two conserved regions (Boxes 1 and 2), one of which (Box 1) is recognized by tyrosine kinases.
The long isoform receptor is already dimerized before ligand binding occurs and can only initiate signaling upon PRL binding to the extracellular domain of the receptor homodimer. Signaling primarily involves activation of the Janus kinase 2 (JAK2)—signal transducer and activator of transcription 5 (STAT5) pathway. In addition, the mitogen-activated protein kinase (MAPK) pathway is activated, and the phosphatidylinositol 3 kinase (PI3K) pathway is also recruited. Activation of these pathways results in altered transcription of target genes in the nucleus that mediate PRL actions.
Prolactin Effects on the Breast
PRL has a critical role in breast development during pregnancy and milk synthesis postpartum, as well as in pathologic states of PRL excess. Of note, PRL stimulates alveolar tissue growth indirectly by stimulating, in synergy with progesterone, the synthesis of the receptor activator of NF-kappa B ligand (RANKL) and insulin-like growth factor 2 (IGF2), both of which act in a paracrine manner to induce alveolar tissue growth. In addition to PRL, several other hormones, including placental lactogen, GH, insulin, cortisol, thyroxine, estrogen, and progesterone, have important roles in the development of breast tissue during pregnancy in preparation for lactation. Of note, high levels of estrogen prevent milk production during pregnancy. In contrast, some degree of estrogen priming of the breast is needed for lactation, and this likely explains the infrequent occurrence of galactorrhea in postmenopausal women and men with hyperprolactinemia. After the placenta is delivered, the rapid decline in estrogen relieves its inhibitory effect on milk production. Postpartum suppression of PRL secretion by bromocriptine leads to a rapid decline in milk production.
Galactorrhea is defined as the secretion of milky fluid from one or both breasts in women who have either never been pregnant or have stopped nursing for over 12 months. Galactorrhea may rarely occur in men. Galactorrhea has been reported in 1% to 45% of women, depending on examination techniques and the patient population under study, and can be an important clue to the presence of PRL excess. Evaluation of PRL levels and thyroid function is advisable in women with galactorrhea. However, only 28% of women with galactorrhea and regular menses have hyperprolactinemia.
Prolactin Effects on Gonadotropin Secretion
In experimental animals, PRL appears to have an essential role in the regulation of the reproductive axis. Thus, mice with targeted disruption of either the PRL gene or the PRL receptor gene have abnormal estrous cycles and are infertile. Whether physiologic PRL levels have an important role with regards to the regulation of the reproductive axis in humans is less clear. Healthy women who are administered bromocriptine to decrease PRL levels to approximately 5 ng/mL have no change in gonadotropin pulsatility, but exhibit higher estradiol levels in the late follicular phase and lower progesterone levels during the luteal phase of the menstrual cycle.
Hyperprolactinemia leads to a marked decrease in gonadotropin secretion, including decreased LH pulse frequency and amplitude, as demonstrated by frequent serum sampling ( Fig. 3.2 ). In menopausal women, hyperprolactinemia blunts gonadotropin levels, which are restored by bromocriptine therapy that lowers PRL levels.

PRL appears to exert direct effects on hypothalamic neurons to suppress GnRH secretion. In vitro, PRL leads to a decrease in GnRH secretion by hypothalamic neuronal cell lines. Hyperprolactinemic mice develop central hypogonadism, characterized by low gonadotropin and sex steroid levels. These animals have decreased kisspeptin 1 expression in the arcuate and anteroventral periventricular nucleus. These data are corroborated by studies in rats, which are also consistent with the hypothesis that PRL in excess blunts kisspeptin 1 expression in the hypothalamus. In hyperprolactinemic mice, administration of kisspeptin 1 restores the function of their reproductive axis, consistent with the hypothesis that kisspeptin 1-expressing neurons in the hypothalamus have a major role in the pathogenesis of hyperprolactinemia-induced hypogonadism.
In experimental animals, hyperprolactinemia blunts gonadotropin secretion after GnRH administration, suggesting that PRL may also directly influence gonadotroph function. However, the findings of similar studies in humans have been less consistent with observations made in animals. Hyperprolactinemic women also exhibit lack of a positive effect of estradiol on gonadotropin secretion.
Prolactin Effects on the Ovary
In experimental animals, PRL has a role in maintaining the function of corpus luteum after ovulation. At physiologic levels, PRL stimulates the expression of 3 beta hydroxysteroid dehydrogenase (type 2) and has an important role stimulating progesterone synthesis in human granulosa cells. However, studies in humans have not been conclusive with regard to the physiologic role of PRL on ovarian function. In healthy women, bromocriptine administration that suppresses PRL levels attenuates progesterone secretion and leads to shorter luteal phases in some, but not all, studies.
At high levels, PRL inhibits aromatase expression in rat granulosa cells and decreases estradiol and progesterone synthesis in human ovaries. In aggregate, available data suggest that PRL excess suppresses the reproductive axis in women at several levels, leading to oligo-amenorrhea. It should be noted that patients presenting with both amenorrhea and galactorrhea are likely to have hyperprolactinemia (75% of 471 patients in aggregated case series). Typically these patients present with secondary, rather than primary, amenorrhea. Low libido and sexual dysfunction is also common in this group. On the other hand, young patients with hyperprolactinemia developing before the onset of puberty may present with primary amenorrhea. These younger patients appear to be less likely to have galactorrhea and may even present with delayed puberty as a result of estrogen deficiency induced by PRL excess.
Hyperprolactinemia appears to be common among women presenting with infertility, representing about one-third of 367 women with infertility in aggregated case series. In a more recent study of women with infertility, the prevalence of hyperprolactinemia was 46%. Although most of these patients also have amenorrhea and galactorrhea, some patients manifest neither symptom but nevertheless have shorter luteal phases. Treatment of hyperprolactinemia in these patients increases progesterone synthesis during the luteal phase and improves fertility. Preovulatory, transient hyperprolactinemia in eumenorrheic women has also been associated with infertility.
Hyperprolactinemia is variably present in women with the polycystic ovary syndrome (PCOS) and is presumed to be a consequence of sustained elevations in estradiol levels that stimulate PRL secretion, though this hypothesis is not universally accepted. In these patients, bromocriptine therapy that suppresses PRL levels may lead to a decrease in testosterone and LH levels and regular menstrual cycles.
Prolactin Effects on the Testis
Whether PRL at physiologic levels is relevant to the regulation of the reproductive axis in males has been a matter of debate. Male mice with targeted deletion of the PRL gene have normal testosterone levels, despite decreased gonadotropin levels, and are fully fertile. On the other hand, approximately 50% of male mice with targeted disruption of the PRL receptor gene have decreased fertility despite apparently normal spermatogenesis. In healthy men, bromocriptine administration for 8 weeks leads to a decrease in testosterone levels, both at baseline as well as after human chorionic gonadotropin (hCG) administration. In human sperm, PRL increases fructose utilization, glycolysis, and glucose oxidation.
Available data suggest that PRL in excess suppresses the reproductive axis in men at several levels. In addition to decreased gonadotropin pulsatility, both baseline and hCG-stimulated testosterone levels are often reduced in hyperprolactinemic men. These patients frequently present with sexual dysfunction (low libido and/or erectile dysfunction) as a consequence of central hypogonadism. Up to 25% of men presenting with erectile dysfunction are hyperprolactinemic. Gynecomastia and/or galactorrhea may develop in hyperprolactinemic men, but are much less frequent than sexual dysfunction in this population. Infertility may also occur in hyperprolactinemic men. Up to 5% of men presenting with infertility have been reported to be hyperprolactinemic. Cabergoline therapy that suppresses PRL excess often leads to improvement in sexual function and recovery of the reproductive axis provided that pituitary gonadotrophs remain intact. Whether PRL normalization is essential for recovery of erectile function is uncertain.
Prolactin Effects on the Adrenal Cortex
Hyperandrogenism of adrenal origin, based on the presence of elevated dehydroepiandrosterone sulfate (DHEA-S) levels, has been reported in some, but not all, studies of women with hyperprolactinemia. Elevation in testosterone levels may also occur in some patients as a consequence of conversion from androgenic precursors. Hirsutism may occur in women with elevated PRL levels, but it is not clear if it correlates well with DHEA-S levels. Bromocriptine therapy that suppresses PRL excess may lead to improvement in DHEA-S levels in women with hyperprolactinemia.
Prolactin and the Skeleton
Several lines of evidence suggest that abnormalities in PRL levels or action are often associated with abnormal bone metabolism. Mice with targeted deletion of the PRL receptor gene have low bone mineral density (BMD) and decreased bone formation. These animals also have low sex steroid levels, which may account for the low BMD, rather than the absence of PRL action.
In humans, PRL excess is associated with deleterious effects on bone. Women and men with hyperprolactinemia and hypogonadism have decreased BMD and increased risk of morphometric vertebral fractures. DA agonist therapy that suppresses PRL excess leads to improvement in BMD. Of note, hyperprolactinemic women who have regular menstrual cycles also have normal BMD. These data are most consistent with the hypothesis that PRL-induced sex steroid deficiency, but not PRL excess per se, underlies the development of low BMD in hyperprolactinemic patients.
Pathologic States of Prolactin Deficiency and Excess
- ◆
PRL deficiency generally signifies extensive pituitary failure.
- ◆
PRL excess (hyperprolactinemia) can be caused by physiologic mechanisms, medications, pituitary, and systemic etiologies, or can be idiopathic.
Prolactin Deficiency
PRL deficiency may occur in patients with pituitary macroadenomas or other large sellar masses, wherein it is frequently associated with multiple additional pituitary hormone deficiencies. In these patients, the presence of PRL deficiency generally indicates advanced pituitary failure as a consequence of destruction of pituitary tissue by tumoral hemorrhage or pituitary surgery. PRL deficiency may also occur in women with Sheehan syndrome (postpartum pituitary infarction in the setting of hemorrhagic shock as a consequence of obstetric complications). These patients classically present with failure of lactation and postpartum amenorrhea. Sheehan syndrome is currently uncommon in the United States and other Western countries because of advances in obstetric care. Isolated, idiopathic PRL deficiency has been reported in three patients, who were unable to lactate, but suffered no other evident manifestations. In clinical studies, recombinant human PRL has been administered with success in some patients with PRL deficiency but is not currently FDA-approved as replacement therapy.
Hyperprolactinemia—Causes
Hyperprolactinemia is relatively common in the general population (0.4%) and can be caused by diverse etiologies ( Box 3.1 ).
Physiologic
Pregnancy
Nursing
Sleep
Food consumption
Coitus
Stress
Medications
Antipsychotic agents (phenothiazines, haloperidol, risperidone, paliperidone)
Monoamine oxidase inhibitors
Fluoxetine
Opioids
Cocaine
Verapamil
Alpha methyldopa
Metoclopramide
Domperidone
Pituitary
Prolactinomas
Somatotropinomas and other co-secreting tumors
Stalk effect in patients with nonfunctioning sellar and suprasellar masses
Radiation therapy
Stalk transection
Systemic
Primary hypothyroidism
Renal failure
Cirrhosis
Adrenal insufficiency
Polycystic ovary syndrome
Spinal cord lesions
Chest wall lesions
Nipple stimulation
Ectopic prolactin secretion
Prolactin receptor gene mutations
IDIOPATHIC
Physiologic
As already noted, PRL levels normally rise postprandially or during slow wave sleep. Acute stress (exercise, coitus, hypoglycemia, seizure, and others) may also lead to a transient increase in PRL levels. Hyperprolactinemia is present during pregnancy and lactation, as already discussed, wherein it has a major physiologic role in breast development and milk production.
Medications
Older antipsychotic agents, including phenothiazines and butyrophenones, cause hyperprolactinemia by virtue of their DA antagonist properties. In these patients, hyperprolactinemia is generally mild to moderate (typically up to 100 ng/mL), but can occasionally be more severe and may exceed 300 ng/mL. Hyperprolactinemia resolves within four days after discontinuation of the offending agent. Some of the newer, “atypical” antipsychotic agents, including risperidone, paliperidone, and molindone, also cause substantial hyperprolactinemia, which may exceed 100 ng/mL. Other atypical antipsychotic agents, including olanzapine, quetiapine, aripiprazole, and ziprasidone, have a lesser effect on PRL levels as a consequence of weaker action on dopamine (D2) receptors and their partial DA agonist activity. As a corollary, the addition of low dose aripiprazole in patients with antipsychotic-induced hyperprolactinemia reduces PRL levels in patients taking risperidone.
Older, tricyclic antidepressants cause mild to moderate hyperprolactinemia in 25% of cases. Monoamine oxidase inhibitors have also been associated with mild hyperprolactinemia. Fluoxetine may raise PRL levels modestly, but these generally remain within the normal range. Other serotonin reuptake inhibitors, bupropion, nefazodone, trazodone, venlafaxine, and lithium do not cause hyperprolactinemia. Opioids and cocaine may also cause mild hyperprolactinemia, which may result in hypogonadism.
Metoclopramide and domperidone inhibit DA receptors and commonly cause hyperprolactinemia and hypogonadism after chronic administration. There have been occasional case reports of hyperprolactinemia linked to use of histamine (H2) receptor inhibitors, proton pump inhibitors, and protease inhibitors, but a causal relationship between these agents and PRL elevation has been disputed.
Some older antihypertensive agents have been associated with hyperprolactinemia. Alpha methyldopa inhibits l -aromatic amino acid decarboxylase, which converts l dopa to DA and acts as a false neurotransmitter interfering with DA secretion, thus causing hyperprolactinemia. Verapamil, but not other calcium channel antagonists (dihydropyridines and benzothiazepines), raises PRL levels by decreasing hypothalamic DA.
Medications cannot be assumed to be the cause of hyperprolactinemia by default, although the timing between medication initiation and onset of symptoms of hyperprolactinemia can be helpful. If the potentially offending medication can be safely discontinued, then PRL levels should be checked 3 to 4 days later. If PRL levels normalize, then hyperprolactinemia can be attributed to the drug. If the medication cannot be safely discontinued, the patient should undergo pituitary imaging to detect a possible sellar mass (as detailed later).
Pituitary
In addition to PRL-secreting adenomas and PRL co-secreting tumors, which are discussed separately, virtually any mass lesion involving the pituitary and stalk may lead to hyperprolactinemia by interfering with DA outflow through the hypothalamic hypophyseal portal system into the anterior pituitary (“stalk effect”). Hyperprolactinemia is generally mild to moderate in patients with stalk effect, with PRL levels generally being below 150 ng/mL in most cases. Previous radiation therapy to the brain, delivered by linear accelerator, has been associated with mild hyperprolactinemia, presumably as a consequence of hypothalamic dysfunction. Similarly, proton beam radiation therapy to the base of the skull has been frequently associated with hyperprolactinemia.
Systemic
Primary hypothyroidism has been associated with mild hyperprolactinemia in up to a third of patients and resolves with levothyroxine replacement. It has been proposed that elevated TRH secretion and increased sensitivity of lactotroph cells to TRH underlie the increased PRL levels in these patients. In rare cases, primary adrenal insufficiency has been associated with mild hyperprolactinemia, which resolves after glucocorticoid replacement.
Chronic renal dysfunction is associated with hyperprolactinemia, which becomes most common in patients with end-stage renal disease, requiring renal replacement therapies. In these patients, hyperprolactinemia appears to be a consequence of decreased PRL clearance and increased secretion and resolves after renal transplantation. Cirrhosis has been associated with hyperprolactinemia in 5% to 100% of patients in different series and has been postulated to occur as a consequence of decreased DA release in patients with hepatic encephalopathy.
Nipple stimulation, chest wall lesions (burns, thoracic zoster, etc.), as well as spinal cord injuries have been associated with the presence of hyperprolactinemia, presumably as a consequence of neurogenic stimulation of PRL release. Very rarely, ectopic PRL secretion has been documented in isolated cases with gonadoblastoma, pituitary tissue present within an ovarian teratoma, bronchogenic carcinoma, and renal cell carcinoma.
Prolactin Receptor Gene Mutation
In one report, three sisters were found to have hyperprolactinemia in the absence of a pituitary tumor or other demonstrable etiology. Two of these patients presented with oligomenorrhea and one had infertility. Of note, the propositus had given birth to 4 children but was treated with bromocriptine because of persistent galactorrhea postpartum. All three patients were found to have a heterozygous, germline mutation of the PRL receptor gene, resulting in a single amino acid substitution (His188Arg), which reduces signal transduction through the JAK-STAT pathway.
Idiopathic
In some patients with hyperprolactinemia, no evident etiology can be found. In such cases, hyperprolactinemia is termed idiopathic. The underlying mechanisms are likely heterogeneous and may reflect the presence of very small PRL secreting adenomas that are below the resolution of current pituitary imaging modalities in some cases, or underlying dysregulation of PRL secretion in other cases. On follow-up, 23 out of 199 patients with idiopathic hyperprolactinemia developed pituitary microadenomas during a 2 to 6 year period. Of note, idiopathic hyperprolactinemia may resolve spontaneously in approximately one third of patients during follow-up.
Hyperprolactinemia—Diagnosis
Patients with hyperprolactinemia require careful evaluation, including history and physical examination. Confirmation of PRL levels by repeat testing after overnight fasting is advisable, particularly in patients with mild hyperprolactinemia. Women of reproductive age should have a pregnancy test. Evaluation of kidney, liver, and thyroid function is also advisable. If no obvious cause is found to explain the presence of hyperprolactinemia, such as pregnancy or primary hypothyroidism, patients should undergo pituitary imaging by magnetic resonance imaging (MRI) using a pituitary protocol (or computed tomography [CT] if MRI is contraindicated, such as in patients with most pacemakers).
There is a good correlation between PRL levels and tumor size in patients with PRL-secreting pituitary adenomas. As a corollary, patients with PRL-secreting macroadenomas generally have PRL levels over 200 ng/mL. In contrast, PRL levels tend to be lower (below 150 ng/mL) in patients with nonfunctioning sellar masses causing stalk effect. As a word of caution, PRL levels should be rechecked in dilution in patients with very large sellar masses who have modest PRL elevations in order to detect a possible hook effect immunoassay artifact which may occur in patients with exuberant PRL secretion (as noted previously). Patients with a microadenoma and modest PRL elevation may have either a functioning tumor (microprolactinoma) or a clinically nonfunctioning adenoma causing stalk effect. Regular pituitary imaging can be helpful in making this distinction, since a prolactinoma will generally shrink in size in response to DA agonist therapy, whereas a nonfunctioning adenoma will not get smaller, and may even enlarge, despite the institution of DA agonist therapy that normalizes PRL levels.
Prolactin-Secreting Pituitary Adenomas (Prolactinomas)
- ◆
Prolactinomas are common, benign pituitary tumors.
- ◆
Clinical manifestations may include mass effect, hypopituitarism, and effects directly attributable to hyperprolactinemia.
- ◆
DA agonist therapy is generally first line treatment for most patients who require therapy, with surgery and radiotherapy having important roles in selected cases.
Epidemiology and Natural History
PRL-secreting tumors represent the most common type of functioning pituitary adenoma. The incidence of these tumors was previously reported as being 10 cases per million per year, and their prevalence as approaching 100 patients per million in the general population. However, more recent studies from several European countries, including Belgium, the United Kingdom, Finland, Iceland, and Switzerland, have suggested that the incidence and prevalence of these tumors is substantially higher (about five times) than previously reported.
By definition, tumors below 10 mm in greatest diameter are termed microadenomas, whereas those that reach or exceed 10 mm are termed macroadenomas. This classification is clinically relevant, as larger tumors are more likely to cause mass effect or invade neighboring structures and have a greater growth potential. Most prolactinomas are microadenomas. However, a larger proportion of macroadenomas occurs in men than women.
A plethora of autopsy studies have identified the presence of clinically unsuspected pituitary adenomas in about 11% of subjects autopsied, with almost all (over 99%) of these tumors being microadenomas. These data are corroborated by the findings of imaging studies, which have identified the presence of incidental pituitary hypodensities consistent with microadenomas in 10% to 20% of study subjects. In autopsy studies, approximately 40% of adenomas examined by immunohistochemistry showed PRL immunostaining.
Several studies have reported on the natural history of untreated microprolactinomas. Out of a total of 139 women followed for up to 8 years, tumor growth was detected in nine patients (6.5%). These studies predated the use of MRI for pituitary imaging, but nevertheless do suggest that the growth potential of microprolactinomas is fairly low.
Macroadenomas are proportionately more common in children or adolescents than in adults, and are also more common in men than in women. Larger tumors tend to have a higher proliferation (Ki67) index. In some cases, tumor growth has been associated with estrogen administration. However, predictors and mechanisms of tumor growth remain poorly understood. It may be noted that most prolactinomas are benign, with frankly malignant tumors, which are, by definition, associated with intracranial or extracranial metastases, being exceedingly rare.
Pathogenesis
PRL-secreting pituitary adenomas are monoclonal tumors. Factors involved in the pathogenesis of these neoplasms are unknown in most cases. Of note, mutations in the gene encoding the D2 dopamine receptor or the gene encoding the G i2 alpha subunit, which couples the D2 receptor to adenyl cyclase, have been found in up to 15% of prolactinomas that are resistant to DA agonist therapy. Somatic mutations in several putative oncogenes or tumor suppressor genes, including ras, myc, Pit-1, PROP-1, c-fos, MENIN, and others, have not been found in these tumors.
PRL-secreting pituitary adenomas develop in about 20% of patients with the multiple endocrine neoplasia 1 (MEN1) syndrome, caused by germline inactivating mutations of the MENIN gene, which is a tumor suppressor gene. Prolactinomas in these patients have been previously reported to behave more aggressively and be more resistant to medical therapy in comparison with sporadic tumors. On the other hand, more recent data in patients with the MEN1 syndrome suggest that prolactinomas generally respond well to medical therapy in this population. The coexistence of a prolactinoma (or other pituitary adenoma) and hypercalcemia secondary to primary hyperparathyroidism defines an index case for MEN1 and has been reported in approximately 14% of patients without known familial disease who were screened for hypercalcemia. About one-third of these patients were also found to have gastrin-secreting enteropancreatic neuroendocrine tumors, which constitute another cardinal manifestation of MEN1. As a corollary, it is advisable to measure serum calcium levels in all patients with presumed prolactinomas.
The syndrome of familial isolated pituitary adenoma (FIPA) has been the subject of substantial investigation in the recent past. In some of these patients, germline, inactivating mutations in the gene encoding the aryl hydrocarbon receptor interacting protein (AIP) have been identified and impart predisposition to pituitary tumor formation. This is transmitted as an autosomal dominant trait with incomplete penetrance and is associated with increased risk of developing GH-secreting tumors, prolactinomas, or co-secreting tumors. Pituitary adenomas present at a younger age in patients with AIP gene mutations and appear to be more aggressive and resistant to medical therapy.
Approximately 10% of prolactinomas are resistant to DA agonist therapy. Decreased expression of D2 DA receptors, including the long DA receptor isoform, decreased D2 receptor density, and DA binding sites on pituitary lactotrophs have been reported in these tumors and may underlie resistance to DA agonists. In addition, decreased expression of transforming growth factor beta 1 (TGF beta1) and several components of its signaling cascade (Smad2 and Smad3) have been reported in tumors resistant to DA agonist therapy. Animal data suggest that TGF beta1 inhibits lactotroph proliferation and PRL secretion, raising the possibility that restoration of TGF beta1 activity might be efficacious as a therapy in patients with prolactinomas that are resistant to DA agonists.
Pathology
PRL-secreting pituitary adenomas are almost always benign. In rare cases, distant metastases within the cranium or in remote locations, such as liver or bone, may develop and are essential to define the presence of malignancy in these patients. Some prolactinomas may co-secrete other hormones, most often GH, but in some cases TSH, ACTH, or FSH. Acidophil stem cell adenomas often have oncocytic features and co-secrete PRL and GH. Symptoms attributable to hyperprolactinemia most often predominate over those related to GH excess in these patients.
Clinical Manifestations
In addition to symptoms and signs directly attributable to PRL excess, patients with PRL-secreting macroadenomas may manifest symptoms related to mass effect or anterior hypopituitarism. Headache may occur as a result of pressure exerted on the dura and can be particularly severe and acute in the setting of pituitary apoplexy, generally occurring as a consequence of hemorrhage within an adenoma. Tumors extending superiorly into the suprasellar cistern may impinge upon the optic apparatus, causing a variety of visual field deficits. Bitemporal hemianopsia is typically noted in patients whose tumors compress the optic chiasm. Central scotomas may develop in patients with compression of one of the prechiasmatic optic nerves and homonymous hemianopsia may occur as a consequence of impingement onto one of the optic tracts. Rarely, large tumors may extend into the third ventricle, causing obstructive hydrocephalus.
Tumors extending laterally often involve the cavernous sinus and may encase the intracavernous segment of the internal carotid artery, but are very unlikely to cause arterial narrowing. Clinical manifestations attributable to compression of the cranial nerves (III, IV, V1, V2, or VI) coursing through the cavernous sinuses, namely ophthalmoplegia, facial pain or numbness, are very uncommon in patients with pituitary adenomas, including prolactinomas. Nevertheless, such symptoms may occur in patients with pituitary apoplexy or occasionally in patients with large, aggressive adenomas. Rarely, large tumors may impinge upon the ipsilateral temporal lobe, causing complex partial seizures.
Tumors extending inferiorly often erode into the sphenoid base and may involve the sphenoid sinus or even the posterior nasopharynx. These patients may rarely develop cerebrospinal fluid rhinorrhea in response to DA agonist therapy. In these cases, medical therapy causes dramatic tumor shrinkage, which unmasks gaps present in the bone and dura, leading to cerebrospinal fluid leakage. These patients typically require neurosurgical intervention in order to close these defects and prevent the attendant risk of meningitis.
Patients with macroadenomas may also develop anterior hypopituitarism and all require thorough evaluation of anterior pituitary function. Of note, diabetes insipidus is extremely unlikely to develop in patients with pituitary adenomas in the absence of surgical intervention. As a corollary, patients who present with central diabetes insipidus and a sellar mass should be suspected of harboring a nonadenomatous lesion (such as a craniopharyngioma or metastasis or an infiltrative lesion), rather than a pituitary adenoma.
Hyperprolactinemia is generally proportionate to tumor size in patients with prolactinomas. Women frequently present with galactorrhea, secondary amenorrhea, or infertility. In a total of 21 case series (1621 women), galactorrhea was present in 85% of women and oligomenorrhea in 93% of women. Men often present with low libido, erectile dysfunction, infertility, gynecomastia, or uncommonly, galactorrhea. In a total of 16 case series (444 men), erectile dysfunction was present in 78% and galactorrhea was present in 11%. As already mentioned, men are more likely than women to harbor macroadenomas. Whether this gender difference is only related to biologic factors, such as sex steroids, which may influence tumor behavior, is debatable; delayed recognition of pituitary adenomas in men who may ignore their symptoms and attribute them to aging is another possibility.
Children and adolescents may present with primary amenorrhea and delayed puberty. As already noted, younger patients are more likely to have macroadenomas, which are resistant to DA agonist therapy. Adolescents with hyperprolactinemia are at increased risk of developing low BMD, which is likely a consequence of hypogonadism occurring during a critical time of peak bone mass acquisition.
Management
Overview
The goals of therapy differ among patients with prolactinomas, depending on their symptoms, tumor size, presence of hypogonadism, and patients’ interest in pregnancy. In patients with macroadenomas, timely decrease in tumor size and long-term tumor control, relief of mass effect, and restoration of pituitary function are all important goals of therapy. In addition, PRL normalization is desirable in all hyperprolactinemic patients who have hypogonadism or desire fertility in order to restore their gonadal function and optimize their fertility potential. Other possible indications for therapy include the presence of bothersome galactorrhea, gynecomastia, hirsutism, and acne, all of which may improve with PRL normalization.
Medical therapy with a DA agonist represents an appropriate first line approach for most patients with prolactinomas who require treatment. However, patients with microadenomas who have central hypogonadism, but are not seeking immediate fertility, can be treated with sex steroid hormone replacement therapy or an oral contraceptive instead of DA agonists, provided that their tumors remain stable during follow-up. Studies in patients with idiopathic hyperprolactinemia or microprolactinomas have not shown a deleterious effect of oral contraceptives on PRL levels or tumor size.
Asymptomatic patients with hyperprolactinemia and microadenomas who have normal gonadal function and are not seeking to improve fertility can be followed expectantly. In this group, it is advisable to monitor the patients’ PRL levels, periodically reassess their gonadal function, and obtain follow-up pituitary MRI examinations in order to detect possible tumor progression (reported to occur in about 7% to 10% of patients over a 4 to 6 year interval, although not all changes are clinically significant) or the subsequent development of hypogonadism, either of which would require the institution of therapy. Although PRL levels and tumor size are generally well-correlated in patients with prolactinomas, it should be noted that tumor growth has been rarely reported in the absence of a change in PRL levels. This observation suggests that periodic pituitary imaging is prudent in patients followed expectantly in order to detect this unusual possibility.
Medical Therapy
DA agonist therapy represents the cornerstone of management of most patients with prolactinomas who require therapy. Bromocriptine and cabergoline are both ergot alkaloid derivatives, and are currently approved by the Food and Drug Administration (FDA) for use in patients with hyperprolactinemia and prolactinomas. Pergolide is no longer available in the United States. Quinagolide, a non-ergot compound, was never introduced in the United States, but is available for use in several other countries.
Bromocriptine was the first drug in this class used to treat prolactinomas and hyperprolactinemia. Bromocriptine normalizes PRL levels in approximately 75% of patients and leads to resumption of menses in the majority of treated women. In addition to inhibiting PRL secretion, bromocriptine inhibits DNA synthesis and cell proliferation, leading to a decrease in tumor size.
About 76% of patients treated with bromocriptine experience a decrease in tumor size over a treatment period of up to 10 years. An example of a tumor response to bromocriptine therapy is shown in Fig. 3.3 . Tumor size reduction is variable in patients treated with bromocriptine. In approximately 40% of 112 patients reported in a total of 10 studies, there was a greater than 50% decrease in tumor size after the institution of bromocriptine therapy. Some tumors can completely disappear on follow-up MRI examinations. In patients with macroadenomas impinging on the chiasm, an improvement in visual field deficits occurs in more than 80% of treated patients and may be noticeable within 1 to 3 days after the institution of bromocriptine therapy. Visible decreases in tumor size occur with a variable time course and can be observed within a period of several weeks to several months on therapy. Pituitary function may also improve in patients whose tumors shrink on bromocriptine therapy. The extent of tumor shrinkage and the magnitude of reduction in PRL levels are not well correlated in all patients. Some of the patients whose tumors do not respond adequately to bromocriptine therapy may be effectively treated with cabergoline (as will be detailed later).
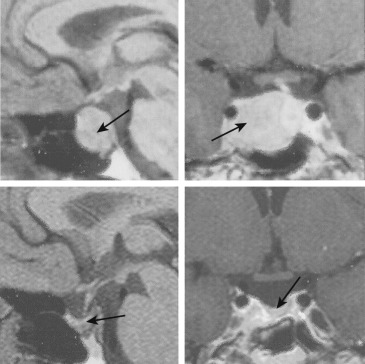
Bromocriptine therapy is usually initiated at a dose of 0.625 mg to 1.25 mg daily, taken in a single nightly dose to optimize tolerance, and is advanced every 3 to 7 days to a dose of 2.5 mg daily. Dose titration may occur in 4-week intervals toward achieving normal PRL levels, provided that the medication remains well-tolerated. The medication is generally given in two or even three divided daily doses, when the total daily dose exceeds 2.5 mg daily to improve effectiveness and tolerance; total daily doses exceeding 10 mg are rarely needed.
Adverse effects associated with bromocriptine therapy most commonly include nausea and orthostatic dizziness. These can be minimized with gradual dose titration and intravaginal administration in some cases. In some patients, these adverse effects may improve over time. Less common adverse effects include headache, nasal congestion, constipation, digital vasospasm, vivid dreams, or nightmares. Pituitary tumor fibrosis may occur in some patients and hinder subsequent attempts at transsphenoidal resection. Rarely, systemic fibrotic manifestations have been reported, including mediastinal and retroperitoneal fibrosis. The association between DA agonist use and cardiac valvulopathy will be discussed later. Other rare, but serious, adverse effects include the development of psychosis or impulsivity, both of which resolve after medication discontinuation. Patients with history of psychosis are, in general, not good candidates for DA agonist therapy. However, psychotic reactions can occur even in patients without such history. Medical therapy of prolactinomas in patients prescribed neuroleptics, which act as DA antagonists, should be carefully reviewed with the treating psychiatrist in order to avoid precipitating an increase in psychiatric symptoms.
Among patients whose tumors decrease in size in response to bromocriptine therapy, the medication dose can often be downtitrated, as the therapeutic benefits can generally be maintained on a lower dose. Eventually, some patients can be considered to be weaned off the medication after a treatment period of 2 to 3 years. Good candidates for DA agonist withdrawal are those who have maintained normoprolactinemia and have no visible tumor on follow-up MRI examinations. Of note, recurrence of hyperprolactinemia may occur after bromocriptine withdrawal. About 20% to 50% of patients with microadenomas and 16% of patients with macroadenomas maintain normal PRL levels after medication withdrawal. As a corollary, patients who are withdrawn from therapy should be warned of the risk of recurrence and monitored with serial PRL levels and MRI examinations to detect possible recurrence, which should prompt the reinstitution of therapy.
Cabergoline is another ergot alkaloid derivative with a very long half-life after oral administration, which generally allows for infrequent dosing (once or twice a week). Cabergoline has high affinity for D2 receptors in the pituitary and is very slowly eliminated as a consequence of its slow release from pituitary binding sites and extensive enterohepatic cycling.
Cabergoline is more effective than bromocriptine in normalizing PRL levels and restoring gonadal function and is generally better tolerated. In one partially double-blind randomized clinical trial of 459 women, most of whom had microprolactinomas, cabergoline therapy led to PRL normalization in 83% and restoration of regular menses in 72% of patients. In the same study, bromocriptine therapy led to PRL normalization in 59% and restoration of regular menses in 52% of patients. Similarly, cabergoline therapy leads to restoration of gonadal function in the large majority of hyperprolactinemic men.
Cabergoline is also effective in reducing tumor size in most patients with prolactinomas. In one study of hyperprolactinemic patients with macroadenomas who were treated with cabergoline therapy, 96% out of 26 patients who were treatment-naive experienced a decrease in tumor diameter exceeding 50% over baseline. In the same study, 64% out of 33 patients who were resistant to bromocriptine experienced a decrease in tumor diameter exceeding 50% over baseline after the institution of cabergoline therapy. Overall, available data indicate that many patients who do not respond to bromocriptine therapy can be treated effectively with cabergoline.
Cabergoline therapy is usually initiated at a dose of 0.25 to 0.5 mg per week, taken at bedtime with a small snack, and is generally titrated every 4 to 6 weeks, aiming to restore normoprolactinemia. More rapid dose titration can be appropriate in patients with large tumors causing mass effect. Most patients can be effectively treated with a total dose of 3.5 mg per week or less. About 10% of patients can be resistant to cabergoline (as well as bromocriptine), even in high doses, and may often require additional therapies, including pituitary surgery, radiation therapy, and/or temozolomide chemotherapy (as will be subsequently discussed).
Cabergoline use has been associated with the same adverse effects noted among patients who are treated with bromocriptine, albeit less frequently. In a clinical trial of 459 hyperprolactinemic women, cabergoline therapy was discontinued in 3% of women because of adverse effects, whereas bromocriptine was discontinued in 12% of women because of adverse effects. Many patients who cannot tolerate bromocriptine can be treated safely with cabergoline, administered either orally or intravaginally. Indeed, published guidelines recommend using cabergoline over bromocriptine in most cases, based on greater efficacy and better tolerance of cabergoline therapy, except in the setting of preconception or pregnancy.
Cardiac valvulopathy has been reported in some patients with Parkinson disease who were treated with very high doses of cabergoline (or pergolide, but not bromocriptine). In these patients, fibrotic lesions in the endocardium occur as a consequence of serotonin (5HT2B) receptor activation by cabergoline and can lead to clinically significant valvular regurgitation. A higher cumulative dose exposure is associated with a higher risk of valvulopathy. In contrast, patients treated with usual cabergoline doses (up to 2.0 mg/week) appear to be at low risk of valvulopathy. It appears sensible to inform patients as to available data and consider performing periodic echocardiographic monitoring on a case by case basis (particularly in patients who require larger than usual cabergoline doses).
Among patients whose tumors decrease in size in response to cabergoline therapy, the medication dose can often be downtitrated, as the therapeutic benefits can generally be maintained on a lower dose. Cabergoline withdrawal can be eventually considered in patients who have responded well to medical therapy for at least 2 to 3 years, remain normoprolactinemic, and have no visible residual tumor on MRI. In a study of hyperprolactinemic patients who had achieved normoprolactinemia on cabergoline therapy, recurrence rates (5-year Kaplan-Meier estimates) for hyperprolactinemia after drug withdrawal were higher among patients with visible residual tumor on MRI and were also higher among patients with macroadenomas than those with microadenomas. Among 105 patients with microadenomas, hyperprolactinemia recurred in 42% of patients with visible residual tumor on MRI and 26% of patients without visible residual tumor at the time of medication withdrawal. Among 70 patients with macroadenomas, hyperprolactinemia recurred in 78% of patients with visible residual tumor on MRI and 33% of patients without visible residual tumor. All patients who are eventually withdrawn from cabergoline therapy should be made aware of the risk of recurrence, and be followed with serial PRL levels and pituitary MRI examinations.
Pergolide is another DA agonist, which was previously used off-label in the United States to treat hyperprolactinemia. Pergolide appears to be comparable to bromocriptine with regard to efficacy and tolerability in patients with hyperprolactinemia. In high doses, pergolide was also used to treat Parkinson disease and was FDA-approved for that indication. The medication was withdrawn from the United States in 2007 because it was associated with cardiac valvulopathy, when administered in high doses in patients with Parkinson disease.
Quinagolide is a non-ergot DA agonist that has been used to treat hyperprolactinemia in some countries. Approximately 50% of hyperprolactinemic patients who are resistant to bromocriptine can be treated effectively with quinagolide. In addition, some patients tolerate quinagolide better than bromocriptine. It is not approved by the FDA and is not currently available in the United States.
Surgery
Pituitary surgery is typically performed transsphenoidally, using an operating microscope or endoscope. Transsphenoidal pituitary surgery is generally considered second-line therapy in patients with prolactinomas.
Patients who should be considered for surgery include those with macroadenomas that do not respond to DA agonist therapy, including patients with persistent chiasm compression or tumor growth during a trial of medical therapy. Some cystic prolactinomas are not responsive to medical therapy and should be considered for resection. Patients with tumors that are resistant to medical therapy are also candidates for surgical intervention. In addition, patients presenting with pituitary apoplexy should be considered for surgery.
Another indication for surgery is intolerance of DA agonist therapy. The rare development of cerebrospinal fluid leak, manifesting as rhinorrhea, or the occurrence of pituitary apoplexy on medical therapy (either of which may rarely occur in patients with prolactinomas being treated with DA agonists) are additional indications for surgical intervention.
Patients with psychotic depression or schizophrenia are not good candidates for DA agonist therapy, as these medications can exacerbate psychosis. These patients should be considered for pituitary surgery, particularly if they have large tumors that are encroaching upon the optic apparatus.
Among patients with microadenomas, individual preference and their desire to avoid medical therapy, if possible, is another possible indication for surgical intervention. However, patients with microprolactinomas opting for pituitary surgery should be referred only to expert pituitary neurosurgeons, who can achieve the best possible outcomes.
Women who have macroadenomas with suprasellar extension and are seeking fertility should be considered for pituitary surgery in order to decrease their risk of tumor progression during subsequent pregnancy, particularly if a trial of DA agonist therapy fails to reduce tumor size. In addition, women with microadenomas who are seeking fertility and are resistant to the effects of DA agonist therapy are candidates for pituitary surgery. However, these patients may also be treated with ovulation induction in order to help them conceive.
The likelihood of achieving complete resection depends on tumor size and location, as well as the skill and experience of the neurosurgeon. In aggregated data from 50 case series, PRL normalization was reported in 75% out of 2137 patients with microadenomas and 34% of 2226 patients with macroadenomas. Recurrence of hyperprolactinemia was reported in 18% of patients with microadenomas and 23% of those with macroadenomas. Long-term remission of hyperprolactinemia occurs in about 60% of patients with microadenomas but only approximately 25% of those with macroadenomas who underwent pituitary surgery. However, pituitary surgery is very effective in quickly relieving mass effect. Patients with visual deficits and large tumors that do not promptly respond to DA agonist therapy should be referred to an expert pituitary neurosurgeon. In these patients, tumor debulking and decompression of the optic apparatus generally leads to improved visual outcomes.
Postoperatively, pituitary function improves in approximately one-third of patients who had evidence of anterior hypopituitarism preoperatively. However, new deficits in pituitary function may also develop in up to 20% of patients after surgery. Permanent diabetes insipidus is uncommon when surgery is performed by an expert pituitary neurosurgeon. Other postoperative complications include epistaxis and cerebrospinal fluid rhinorrhea. Very uncommonly, tumor bed hemorrhage, meningitis, or stroke may occur. Perioperative mortality risk is about 0.2% to 0.5% and approaches zero in expert hands. Perioperative morbidity is also lower among patients operated on by more experienced neurosurgeons.
Other Treatment Modalities (Radiation Therapy and Chemotherapy)
In patients with prolactinomas, the role of radiation therapy (radiotherapy) is limited at present. Radiation therapy is very effective for tumor control and is advisable in patients with macroadenomas who require tumor control and cannot be adequately treated with DA agonist therapy and surgery. Of note, PRL normalization has been reported in only 35% of patients treated with a combination of surgery and radiation therapy, and generally takes 5 to 15 years after radiotherapy to occur. Increasingly, radiation therapy is administered using stereotactic techniques that deliver either photons (Gamma knife, Cyberknife) or protons selectively to the target. These newer modalities largely spare the normal brain tissue from radiation exposure in comparison with older, conventional radiotherapy methods. Stereotactic radiation therapy can be administered in a single fraction (a technique known as radiosurgery) in appropriate candidates, who have smaller tumors that are distant from the optic apparatus. Based on historical comparisons, it has been suggested that radiosurgery is associated with a faster endocrine response than conventional radiation therapy.
Anterior hypopituitarism is frequent after radiation therapy, regardless of the modality used for administration. This complication occurs in about 40% of patients at 5 years after radiation therapy and becomes even more prevalent long-term. As a corollary, all patients who receive radiation therapy require lifelong yearly reassessment of pituitary function and replacement of hormone deficiencies as needed.
Other uncommon complications of radiation therapy include optic neuropathy or other cranial neuropathies. Infrequent complications in patients who received conventional radiation therapy include stroke, temporal lobe necrosis, or secondary tumor formation, which may occur several decades after radiation therapy. Whether stereotactic radiation therapy is associated with a lower risk of developing these very infrequent complications remains unknown.
Temozolomide is an orally active alkylating agent, which has useful activity against many gliomas, including glioblastoma multiforme. Temozolomide exerts its effects by methylating DNA bases, resulting in DNA fragmentation and cell death. This drug has been helpful for tumor control in several patients with large, locally aggressive prolactinomas or the rare PRL-secreting carcinomas that continue to enlarge despite adequate DA agonist therapy, surgery, and radiation therapy. Up to 73% of patients with aggressive PRL-secreting adenomas appear to respond to temozolomide, using either tumor size or PRL levels as treatment endpoints. Expression of the DNA repair enzyme O6-methylguanine-DNA methyl-transferase (MGMT) in the tumor is inversely correlated with response rates to temozolomide in several, but not all, studies. Lack of a treatment response after three cycles of temozolomide therapy predicts resistance to this agent in patients with aggressive pituitary adenomas (including prolactinomas). Escape from the salutary effects of temozolomide on tumor growth may occur.
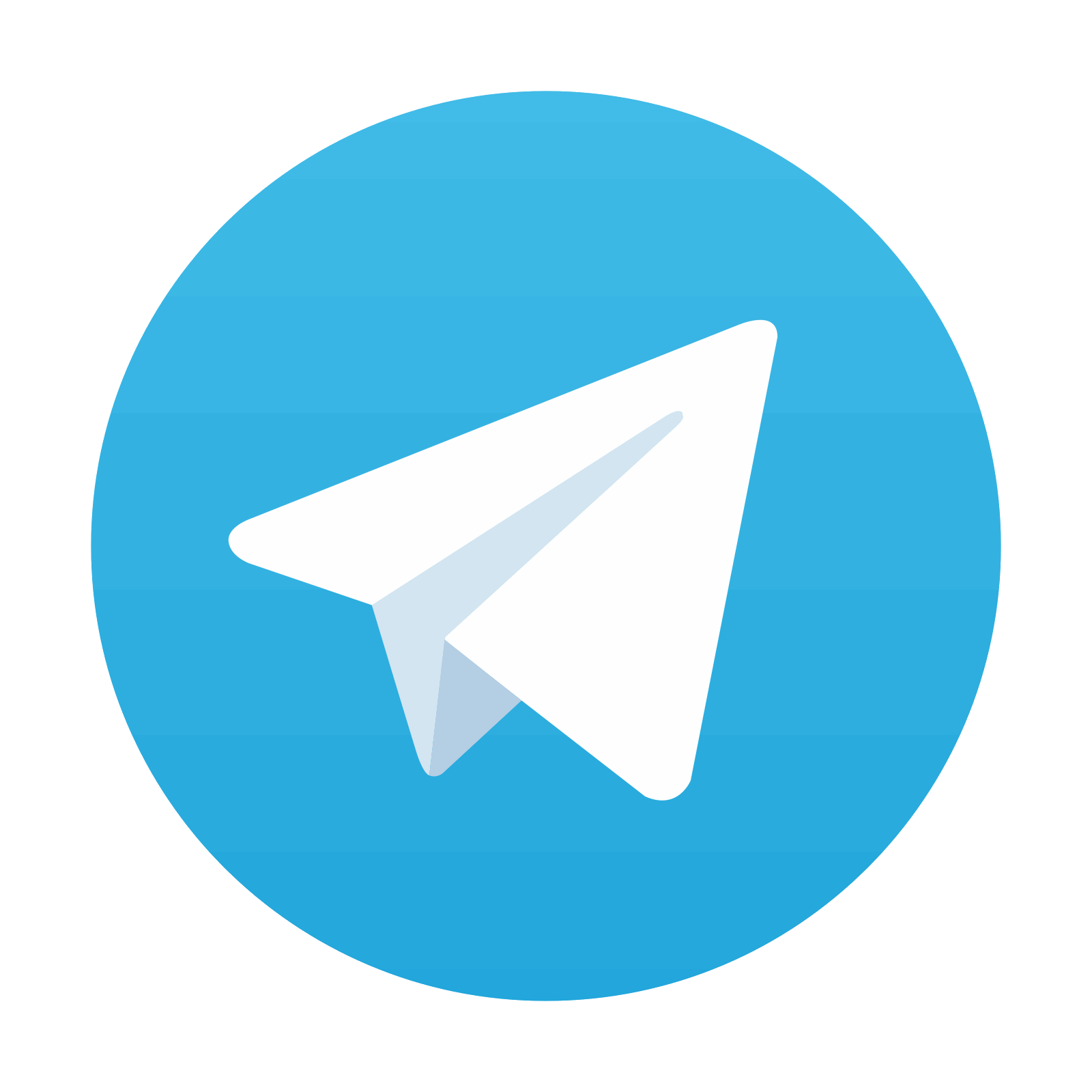
Stay updated, free articles. Join our Telegram channel

Full access? Get Clinical Tree
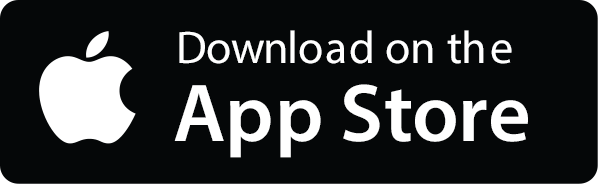
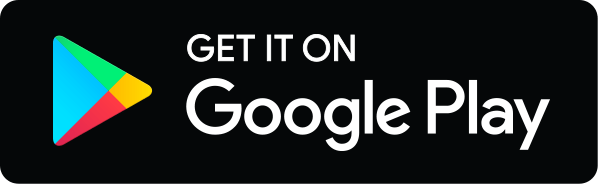