Abstract
The primary biological function of the breast is to produce milk that provides both nutrition and immunoprotection for a newborn. The key hormones involved in breast growth, differentiation and lactogenesis are estradiol, progesterone, prolactin, growth hormone, insulin-like growth hormone-1 (IGF-1), and oxytocin. In women who are exclusively breastfeeding, suppression of kisspeptin and gonadotropin-releasing hormone (GnRH) activity results in oligo- or anovulation. Within the first 6 months of birth, exclusive breastfeeding without the use of supplements and minimization of intervals without breastfeeding in the presence of continuing amenorrhea is associated with a low chance of becoming pregnant. Breastfeeding may reduce the mother’s risk of endometriosis, type 2 diabetes mellitus, heart disease, breast cancer, and ovarian cancer.
Keywords
Mammary stem cells, estradiol, progesterone, prolactin, oxytocin, growth hormone, IGF-1, β-casein protein, lactogenesis, breastfeeding.
Structural Features of the Breast
- ◆
The primary biological function of the breast is to produce milk that provides both nutrition and immunoprotection for a newborn.
The primary biological function of the breast is to produce milk to nurture newborns. Mammals are differentiated from other animal classes by the production of breast milk to nurture newborns, the presence of hair on the skin and live birth. The word mammal is derived from the Latin “mamma,” which means “resembling the milk gland or breast.” The breast overlies the second to the sixth ribs. The medial border of the breast is the sternum, and the lateral border is the latissimus dorsi muscle. The superior and inferior borders are the clavicle and the costal margin and upper rectus sheath. The breast consists of glandular, adipose, and connective tissues.
The glandular tissue of the human breast is arranged in approximately 15 to 20 lobes ( Fig. 10.1 ). Each lobe consists of a branching structure made up of lobules and acini (also referred to as alveoli ). The acini are lined by a single layer of milk-secreting epithelial cells. Each acinus is surrounded by an interwoven pattern of contractile myoepithelial cells. The lumens of the acini connect to collecting intralobular ducts, which empty into the main 15 to 20 lobar collecting ducts. In turn, each lobe drains into the nipple. The nipple is surrounded by a pigmented areola. The sebaceous glands located on the perimeter of the areola are referred to as the glands of Montgomery. The glandular tissue is embedded in fat, which accounts for most of the mass of the breast. The lobules are separated by connective tissue septa (Cooper ligaments) that run from the subcutaneous tissue to the chest wall.
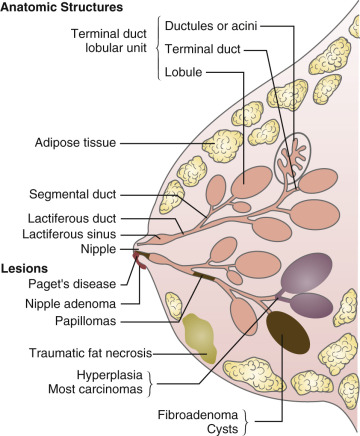
In the mouse, the main histologic component of the mammary gland is a duct with a luminal epithelial layer and an outer myoepithelial layer. One end of the duct is connected to a larger milk collecting duct, and the other end is connected to a terminal end bud (TEB). During mammary growth, the TEB advances into the surrounding adipose tissue until it reaches the end of the fat pad and then the TEBs recede. The TEB consists of an outer layer of cap cells that differentiate into the outer myoepithelial layer of the duct and an inner layer of body cells that differentiate into luminal cells. Mammary stem cells (MaSCs) and progenitor cells reside in the ducts within the luminal and myoepithelial layers. MaSCs give rise to progenitor cells, which can differentiate into any of the cell types residing in the gland.
The breast is sensitive to the sex steroids estradiol, progesterone, and testosterone and to numerous protein hormones, including prolactin, oxytocin, insulin-like growth hormone-1 (IGF-1), and other growth factors. During puberty and pregnancy, the breast is stimulated to grow. Estradiol is especially important in stimulating the growth of the ductal system, and progesterone is important in stimulating the growth of the acini and stromal elements.
Development of the Breast
- ◆
The key hormones involved in breast growth, differentiation, and lactogenesis are estradiol, progesterone, prolactin, growth hormone, IGF-1, and oxytocin.
- ◆
In women who are exclusively breastfeeding, suppression of kisspeptin and gonadotropin-releasing hormone (GnRH) activity results in oligo- or anovulation.
The development of the breast occurs in three major phases: in utero, at puberty, and during pregnancy. This process has been studied best in mice. During in utero development of the breast, the growth of epithelial elements into the underlying mesenchyme results in the development of a rudimentary ductal system. This process is independent of stimulation by estradiol, progesterone, prolactin or growth hormone (GH). Immediately after birth, the rudimentary mammary ducts are quiescent. In the mouse, the majority of mammary duct morphogenesis occurs during puberty.
During puberty, the mammary gland fully develops its tubular complexity through branching morphogenesis. The branched ductal network that forms extends into the stromal fat pad, and the branching process is led by the TEB. The differentiated mammary gland is composed of luminal epithelial cells and surrounding basal myoepithelial cells. Estradiol working through the estrogen receptor alpha (ERα) is critical for pubertal mammary gland development. Estradiol stimulates epithelial cell proliferation and promotes the elongation and branching of ducts into the mammary fat pad. ERα-null mice do not have mammary development. In humans and mice, proliferating breast epithelial cells do not express ERα, suggesting that estradiol action is mediated by a paracrine mechanism. Estrogen receptor beta (ERβ) is abundantly expressed in mammary glands, but mice lacking ERβ have normal mammary development. In addition, conditional knockout of ERα during pregnancy or lactation causes a loss of ductal side-branching and a decrease in the number of lobuloalveolar units.
In mice, progesterone signaling through the progesterone receptor (PR) promotes ductal branching and stimulates alveolar development during pregnancy. PR has two isoforms, PRA and PRB. Compared with PRA, PRB contains an additional 165 amino acids, third transcription activation function domain at its 5′ end. In mice, progesterone signaling is mediated by PRB in the breast and PRA in the ovary. Progesterone stimulates the production of two paracrine mediators RANKL and WNT-4, which stimulate duct branching and alveologenesis. In humans and mice, proliferative activity of the breast is highest postovulation (luteal phase in humans, diestrus in mice) when both progesterone and estradiol are present. During diestrus, breast stem/progenitor cells undergo a 14-fold expansion compared with estrus. The coactivators and corepressors that modulate ER and PR action are described in detail in Chapter 5 .
In the mouse, knockout of the prolactin gene by homologous recombination causes infertility in female mice, but not male mice. The mammary glands of prolactin knockout mice have a normal ductal tree but fail to develop alveolar structures. Knockout of the prolactin receptor gene causes both inhibition of ductal branching and failure of the development of alveolar structures. During pregnancy, progesterone stimulates epithelial proliferation and alveolar morphogenesis and prolactin stimulates alveolar development and lactogenesis. Prolactin binding to the prolactin receptor stimulates the intracellular Jak2/p-STAT 5, which activates the expression of milk protein genes. Prolactin also stimulates the production of the transcription factors GATA3 and ELF5, which stimulate the differentiation of luminal progenitor cells into secretory cells capable of producing milk proteins.
GH, IGF-1, and insulin are important regulators of breast development. GH regulates breast development by increasing circulating IGF-1 through actions on the liver and by directly increasing IGF-1 production in mammary tissue. In the mouse, intramammary production of IGF-1 is more effective in promoting ductal development than circulating IGF-1, suggesting a paracrine rather than classic endocrine mechanism. In human breast tissue in vitro, progesterone stimulates the production of GH, which act through a paracrine mechanism to stimulate duct branching and alveologenesis. IGF-1 amplifies the effect of estradiol and progesterone on mammary gland development and knockout of IGF-1 or the IGF-1 receptor in mouse mammary tissue limits ductal growth. In women with atypical hyperplasia of the breast, administration of pasireotide, a somatostatin analogue that blocks GH secretion and IGF-1 action, decreased breast cell proliferation and increased apoptosis. However pasireotide caused significant hyperglycemia. In lactating cows, administration of bovine GH, a common farming practice, increases milk production by about 10%.
The epidermal growth factor receptor (EGFR) and its ligands, epidermal growth factor (EGF), amphiregulin, and transforming growth factor-alpha (TGFα) are involved in mammary development and growth. In a human mammary cell line, the fate of precursor cells was differentially regulated by EGFR ligands. When stimulated by EGF, mammary cells differentiated into both luminal and myoepithelial cells. Stimulation by amphiregulin resulted in the development of luminal cells but not myoepithelial cells, while stimulation with TGFα resulted in the development of only myoepithelial cells. In mice, EGFR appears to be absolutely required for ductal development but not for the development of the acini. Estradiol and progesterone stimulation of mammary gland development can be partially blocked by inhibiting EGF receptor activity.
In the mouse, parathyroid hormone-related protein (PTHrP) and the type 1 parathyroid hormone receptor (PTHR1) are required for the in utero development of rudimentary mammary glands. In a human fetus with an inactivating mutations of PTHR1 (Blomstrand chondrodysplasia), breast tissue did not develop. Full elongation and branching of the breast duct system requires luminal cell-mesenchymal cell cross-talk. In utero, PTHrP produced by luminal epithelial cells stimulates PTHR1 on mesenchymal cells resulting in duct branching and elongation through WNT and bone morphogenetic protein (BMP) signaling. After birth, PTHrP is expressed at low levels in the breast. With the onset of lactation, modest amounts of PTHrP are secreted into the maternal circulation, and large amounts of PTHrP are secreted into breast milk. In the mouse, PTHrP secreted by the breast into the maternal circulation mobilizes calcium from the skeleton into the circulation to support sufficient calcium concentration in milk. Calcium sensing receptors (CaSRs) in the breast respond to elevated circulating calcium concentration by suppressing PTHrP secretion, thereby closing a classic endocrine feedback loop. In case reports, women with primary hypoparathyroidism have been reported to have temporary resolution of their hypocalcemia during lactation, likely due to mammary production of PTHrP and increased circulating PRHrP. In some lactating women, severe osteoporosis and vertebral fractures have been associated with elevated circulating concentrations of PTHrP and hypercalcemia. In these women, following discontinuation of lactation, circulating PTHrP returned to normal levels, calcium homeostasis normalized, and bone density improved.
During the normal menstrual cycle, the breast undergoes cycles of growth and quiescence. In the early follicular phase, the ductal cells proliferate. In the luteal phase of the cycle, progesterone stimulates the proliferation of the terminal duct structure, basal epithelial cells, and stromal cells. Accompanying these proliferative changes is an increase in stromal edema and vacuolization of epithelial cells. These effects may account, in part, for the sense of breast fullness women experience during the late luteal phase. At menses, the decrease in estradiol and progesterone is associated with a decrease in cell proliferation, stromal edema, and the size of the ducts and acini.
The two most common congenital anomalies of the breast are supernumerary nipples and accessory axillary breast tissue. Supernumerary nipples result from the persistence of epidermal thickenings along the embryologic milk line. Supernumerary nipples can extend from the axilla to the suprapubic area. On occasion, breast tissue extends into the axillary fossa. Ectopic breast tissue in this region can be mistaken for an axillary mass or may cause pain after pregnancy because of the engorgement of the tissue with milk.
Mammary Stem Cells
In early pregnancy, estradiol, progesterone, and prolactin stimulate epithelial cell proliferation and the formation of alveoli. In late pregnancy under the stimulation of prolactin, alveolar cells synthesize and secrete milk proteins. The dynamic development of the breast during pregnancy relies on MaSCs that can expand and differentiate to support lactation. Mammary epithelial structures in both mice and women contain cells spanning a developmental hierarchy from pluripotential stem cells to progenitor cells committed to populate the epithelial structures to mature terminally differentiated luminal cells. It has been demonstrated that a single MaSC can generate an entire functional mammary gland. MaSCs that are located in the basal epithelium generally do not express ERα or the PR, but administration of estradiol, progesterone, or prolactin to female mice increases the number and clonogenicity of MaSCs. This suggests the presence of paracrine cross-talk between the luminal epithelium and the basal MaSCs. Progesterone and prolactin stimulate luminal epithelial cells to produce and secrete RANKL and WNT4, which in turn stimulate the clonogenicity of MaSCs.
Estradiol and Testosterone: Dueling Steroids
A genetic experiment of nature, androgen insensitivity syndrome , provides a clinical example of the important interplay between estrogens and androgens in the regulation of breast growth. In androgen insensitivity due to mutations in the androgen receptor (AR), genetic males (46,XY) do not have a fully functional AR. Testosterone is produced by the testis, but target tissues are not capable of responding to the high levels of circulating androgens. In this syndrome, circulating estradiol concentration is in the range of 50 pg/mL, comparable to early follicular-phase levels observed in women . Breast volume in individuals with androgen insensitivity is typically above average. This suggests that, in the complete absence of androgen inhibition, modest levels of estradiol are capable of stimulating significant breast growth. Progesterone levels are low in individuals with loss of the AR. This suggests that breast volume is not absolutely dependent on progesterone stimulation.
In the mouse, ARs are present in the mammary luminal epithelial cells, stromal fibroblasts, and adipocytes. Hormone-sensing mammary epithelial cells that contain ERα and PRB also contain the AR. In female mice, administering androgen antagonists during puberty markedly increases the number of proliferating breast epithelial cells. In the adult female monkey, administering androgen antagonists enhances estrogen-stimulated breast epithelial cell proliferation. In cultures of human breast tissue, dihydrotestosterone (DHT) and testosterone inhibit estradiol-induced stimulation of cell proliferation, cell survival, and the density of ERα containing cells. The antagonist effect of androgens on estrogen stimulation of breast epithelial proliferation is the basis for the use of androgens to treat breast pain syndromes (see section Cyclical Mastalgia ). In human breast cancer, androgen-independent splice variants of the AR play a role in cell proliferation in some cancers.
Gynecomastia is the presence of excessive breast glandular tissue in men. Approximately 50% of men greater than 50 years have gynecomastia at autopsy. In many older men, gynecomastia can be detected on physical exam. Gynecomastia can be detected on physical examination by having the patient in the supine position and placing the index finger at 12 o’clock and the thumb at 6 o’clock on the breast skin just outside the areolar rim. The finger and thumb are then slowly brought together. If a rubbery-firm mound of concentric tissue is grasped between the two fingers, gynecomastia is likely present. If no disk of tissue is detected, gynecomastia is unlikely, and excess fat is the likely cause of the enlarged breast. In most cases, gynecomastia is caused by a relative excess of free estradiol and decrease in free testosterone. The most common causes of gynecomastia are aging, antiandrogen medications, cirrhosis, dialysis in the setting of renal failure, hyperthyroidism, and estradiol secreting testicular or adrenal tumors. If a specific cause can be identified, treatment may cause regression of the excess glandular tissue. If the gynecomastia is long standing, spontaneous regression is unlikely. In this setting, if the gynecomastia is bothersome to the patient, subcutaneous mastectomy or liposuction are the best treatment options.
Inducing the growth of breast tissue is important for many male to female (MtF) transgender individuals because breasts are viewed as a physical sign of gender (see Chapter 28 ). In feminizing treatment of MtF transgender individuals, two agents are commonly used, estrogen to stimulate breast growth plus an antiandrogen to block the inhibition of breast growth by testosterone secreted from the testes. In MtF individuals, prior to orchiectomy, transdermal estradiol 0.1 mg/day is applied and after a few weeks, the dose is increased to 0.2 mg/day. To enhance breast growth, estrogen therapy is combined with an antiandrogen. The antiandrogen, spironolactone, can be initiated at a dose of 100 mg daily with an increase in dose over a few months to 300 mg daily. After orchiectomy, with the resulting decrease in circulating testosterone, lower doses of estradiol and an antiandrogen can be used to maintain breast size. After orchiectomy, a regimen of transdermal estradiol 0.1 mg/day plus spironolactone 50 mg daily can be used to maintain breast size.
Lactation and Breastfeeding
In reproduction, the primary role of the breast is secretion of milk to nurture a newborn. Breast milk is recommended, as the exclusive source of nutrition for the newborn for 6 months followed by the gradual introduction of solid foods and liquids and continuation of breastfeeding for another 12 months. For the newborn, breast milk provides both nutrition and immunoprotection. Breastfeeding reduces the risk of childhood hospitalizations, gastroenteritis, respiratory infections, otitis media, urinary tract infections, and sepsis. Conflicting data have been reported on the potential association of breastfeeding and protection from childhood obesity, type 1 diabetes mellitus, cancer, and allergic conditions. Breastfeeding has significant long-term health benefits for the mother. Breastfeeding reduces the risk of the mother developing breast cancer, ovarian cancer, type 2 diabetes, and cardiovascular disease.
Breast milk consists of milk proteins, including casein, whey, and mucin proteins, lipids (98% triglycerides), the milk sugar lactose, oligosaccharides, immunoglobulins, water, and minerals and vitamins. The oligosaccharides in milk cannot be digested by the fetal gut, but they can be used as a fuel by Bifidobacterium longum biovar infantis , a beneficial gut bacteria that comprises up to 90% of the gut microbiome of the breastfed infant and less than 3% of the gut microbiome following weaning. Bifidobacterium longum biovar infanti s produces unique glycosidases that permit the bacterium to digest oligosaccharides, suggesting that this bacterium coevolved with mammals.
In pregnancy, the breast is exposed to high levels of lactogenic hormones, including prolactin, human placental lactogen, and placental GH. In nonpregnant women, prolactin levels are typically below 25 ng/mL. During the third trimester of pregnancy, prolactin levels are typically 15-fold higher in the range of 200 to 450 ng/mL. Human placental lactogen reaches concentrations in the range of 6000 ng/mL during the third trimester. The human prolactin receptor can be activated by prolactin, GH, and placental lactogen. These lactogenic stimuli cause the breast to grow and prepare the alveoli for lactation. During pregnancy, the high concentration of progesterone blocks lactogenesis. After delivery, the decrease in circulating estradiol and progesterone concentration and the continued elevated prolactin concentration result in an increase in the production of all of the components of milk. Milk production also requires the synergistic action of GH, insulin, thyroxine, and cortisol. The molecular mechanisms subserving these effects are discussed later in this chapter. Following birth and the resulting decrease in estradiol and progesterone, milk production requires two to five days to become established. In the postpartum interval, milk production can be suppressed by treatment with large doses of estradiol and progesterone or by suppressing prolactin secretion.
During suckling, sensory signals originating in the nipple travel through thoracic nerves 4, 5, and 6 to the central nervous system. When the suckling-induced signals reach the paraventricular and supraoptic nuclei, they stimulate the release of oxytocin from the posterior pituitary. Noradrenergic, histaminergic and excitatory amino acids play a role in oxytocin release. Oxytocin release during suckling causes the contraction of the myoepithelial cells of the mammary acini and ducts, which induces the flow of milk. In addition to suckling, oxytocin can be released from the posterior pituitary by visual and auditory stimuli.
Prolactin maintains lactogenesis by stimulating the transcription of the casein and lactalbumin genes and other genes needed for the synthesis of free fatty acids and lactose. Suckling-induced signals also cause the hypothalamus to release of prolactin in part by suppressing dopamine secretion. In lactating women, in the first 6 weeks postpartum, basal prolactin levels are elevated, and each episode of breastfeeding initiates an increase in prolactin, which peaks at levels 8 times higher than basal concentration. From 7 to 28 weeks postpartum, basal prolactin levels are in the normal range, and the magnitude of the spike in prolactin levels with suckling is about sixfold above baseline. Use of a breast pump produced spikes in prolactin similar to those seen with suckling, but manual stimulation of the areola does not reliably produce a spike in prolactin. Having breastfeeding mothers play with their infants caused milk-let down but did not produce a spike in prolactin levels. As the frequency and duration of suckling diminishes, the magnitude of the spike in prolactin levels following suckling declines, eventually becoming extinguished.
Effect of Prolactin on β-Casein Gene Function
The cellular mechanisms that control milk protein production have been best elucidated for β-casein. In the murine mammary epithelial cell line HC11, β-casein protein production is largely regulated at the transcriptional level by prolactin, glucocorticoids, and progesterone. Prolactin and glucocorticoids stimulate transcription, and progesterone inhibits transcription.
Prolactin and glucocorticoids are the key endocrine regulators of β-casein gene transcription. The prolactin receptor is a class 1 receptor with extracellular cysteine motifs, a single transmembrane-spanning domain, and the absence of an intrinsic receptor-associated tyrosine kinase in the intracellular domain. Prolactin binding to its receptor results in dimerization of the receptor and activation of the tyrosine Janus kinase 2 (JAK2) associated with the membrane-proximal, intracellular domain of the receptor (see Chapter 3 ).
In turn, JAK2 phosphorylates and activates STAT5, a member of the signal transducers and activators of transcription (STAT) family. STAT5, previously known as mammary growth factor (MGF), increases transcription of the β-casein gene. Various phosphatases, which are themselves regulated, dephosphorylate STAT5 and reduce the transcription of β-casein gene transcription. STAT5 stimulation of milk protein gene transcription requires the presence of insulin and cortisol.
Prolactin and glucocorticoid stimulation of β-casein gene transcription is dependent on a proximal promoter region (−235 bp) and a distal enhancer, −6 kb upstream of the transcription start site. The recruitment of transcription factors (STAT5, p300, RNA polymerase II) and the formation of a chromatin loop bring these two sites together to enhance β-casein gene transcription. The progesterone-PR complex binds to the proximal promoter and distal enhancer region, preventing both the formation of chromatin loop and the assembly of transcription factors into an activation complex.
Breastfeeding and Amenorrhea
- ◆
Within the first 6 months of birth, exclusive breastfeeding without the use of supplements and minimization of intervals without breastfeeding in the presence of continuing amenorrhea is associated with a low chance of becoming pregnant.
Lactation stimulates multiple neuroendocrine processes that suppress both kisspeptin activity and GnRH secretion (see Chapters 1 and 3 ) and hence follicle growth and ovulation. The lactational amenorrhea method of contraception is the intentional use of exclusive breastfeeding to reduce the risk of pregnancy during the first 6 months following birth. When a woman is exclusively breastfeeding and amenorrheic, the risk of becoming pregnant during the first 6 months following birth is less than 3%.
During suckling, activation of dopamine, serotonin, gamma-aminobutyric acid, and corticotropin-releasing factor systems inhibit kisspeptin activity and GnRH secretion resulting in oligoovulation or anovulation. In rats, lactation reduces the number of kisspeptin containing neurons in the arcuate nucleus and median eminence, thereby suppressing GnRH secretion. In addition, lactation increases prolactin secretion, and prolactin directly stimulates phosphorylation of STAT5 in kisspeptin and dopamine containing neurons, thereby suppressing kisspeptin activity and increasing dopamine activity. The decrease in kisspeptin activity and the increase in dopamine activity inhibits GnRH secretion. In addition, direct neural signals from the breast to the area of the hypothalamus containing kisspeptin neurons also reduce kisspeptin activity.
The degree to which breastfeeding suppresses GnRH secretion is modulated by the intensity of the breastfeeding, the nutritional status, and the body mass of the mother. Lactation represents a metabolic energy burden. When nutrition is adequate and the basal body mass and body composition is normal, intensive lactation is less likely to result in prolonged suppression of GnRH. When nutrition is inadequate to meet the energy demands of both daily living and lactation, oligoovulation or anovulation is more likely to persist for a greatly extended time period.
During exclusive breastfeeding, approximately 40% of women will remain amenorrheic at six months postpartum ( Fig. 10.2 ). In observational studies performed in the United Arab Emirates, the median duration of postpartum amenorrhea in breastfeeding women was 7 months. Women who remain amenorrheic during breastfeeding may have higher prolactin levels than do women who become ovulatory while breastfeeding. Among postpartum women who do not breastfeed, return of ovulation, as determined by urinary pregnanediol measurement, occurs between 45 and 94 days postpartum. In some women who exclusively breastfeed; with at least six episodes of suckling per day, totaling more than 80 minutes in 24 hours; basal prolactin levels remain elevated up to 52 weeks postpartum, and amenorrhea can persist for 1 year.
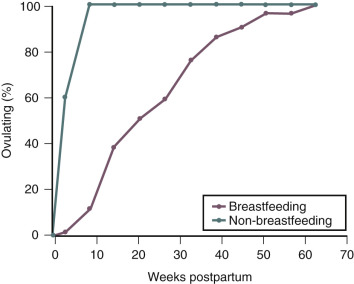
During the first 6 months following birth, if a woman breastfeeds exclusively, uses no supplements, minimizes intervals without breastfeeding during the day and night, and remains amenorrheic, the risk of pregnancy is less than 3%. Pregnancy rates rise exclusively among breastfeeding women 6 months following birth, and initiation of a complementary method of family planning is recommended at that time.
Effect of Steroid Contraceptives on Lactation and Breastfeeding
The available data indicate that (1) estrogen-progestin contraceptives reduce milk volume and change breast milk composition, (2) progestin-only contraceptives have no effects on milk volume and minimal effects on breast milk composition, and (3) long-term studies of the infants of breastfeeding mothers who used either estrogen-progestin contraceptives or progestin-only contraceptives show no detrimental effects of contraceptive steroid use on infant growth or neurodevelopment. Taken together, these data indicate that there is no significant effect of the use of contraceptive steroids by breastfeeding mothers on the development of their infants. The Center for Disease Control (CDC) concluded that for breastfeeding mothers, one month after birth, there are no restrictions on the use of a progestin-only contraceptive pill, progestin contraceptive injection, or progestin implant. The CDC also concluded that for breastfeeding women, one month after birth, the advantages of the combination estrogen-progestin contraceptive generally outweigh any theoretical or proven adverse effect on milk quantity or quality. The use of a combination estrogen-progestin contraceptive in the first month after birth is not recommended because of the increased risk of postpartum venous thromboembolism.
Most studies, but not all, report that estrogen-progestin contraceptives reduce breast milk volume more than progestin-only contraceptives. For example, in one study of more than 170 lactating women, the combination of 30 µg of ethinyl estradiol and 150 µg of levonorgestrel reduced daily milk volume by 31%. In contrast, a progestin-only minipill containing 75 µg of norgestrel increased daily milk volume by 10%. Similarly the use of depot medroxyprogesterone acetate increased milk volume by 10%. Combination estrogen-progestin contraceptives also decrease the concentration of nitrogen, lactalbumin, lactoferrin, and lactose in breast milk. These changes in the composition of breast milk are modest and within the normal range of physiologic variation observed in lactating women who are not taking contraceptive steroids. A recent, short-term randomized trial of the effects of a progestin-only minipill (norethindrone 0.35 mg daily) versus a cyclic estrogen-progestin contraceptive (35 µg ethinyl estradiol plus 1 mg of norethindrone in a 21/7 regimen) on breastfeeding reported no differences in the mother’s perception of an adequate milk supply by contraceptive regimen. In this study, consistent with other studies, the growth of the infants was similar with the two contraceptive methods.
The development of the newborn does not appear to be significantly affected by the changes in milk composition and volume associated with the use of combination estrogen-progestin oral contraceptives. In one study, the weight and neurologic development of newborns of lactating mothers who took estrogen-progestin contraceptives were similar to those whose mothers did not. A comparative study of the effects on lactation of a progestin-only contraceptive implant versus a copper intrauterine device (IUD) reported that infant growth was similar for both treatments. Medicated and nonmedicated IUDs do not appear to affect the growth of breast fed children. The combination of lactation plus a progestin-only contraceptive is likely to be associated with a prolonged interval of amenorrhea compared with lactating women who use an IUD for contraception.
Suppression of Lactation
Prolactin secretion is crucial to the maintenance of lactation. Suppression of prolactin secretion with dopamine agonists such as bromocriptine will suppress lactation. However, there are case reports of severe hypertension, ischemic stroke, myocardial infarction, and cerebral angiopathy in postpartum women who used bromocriptine. Consequently, dopamine agonists such as bromocriptine are not recommended for suppression of lactation. In most mothers, lactation suppression can be achieved with minimization of the mechanical stimulation of the breast and nipple, ice packs, and antiprostaglandin treatment. Milk stasis decreases the production of STAT5, the main intracellular activator of lactogenesis.
Induction of Lactation
Induced lactation is defined as breastfeeding in the absence of a recent pregnancy. On occasion, adoptive mothers desire to breastfeed. In countries where access to formula is limited, induced lactation in a surrogate may be important if the biologic mother cannot continue to breastfeed. The key hormone involved in lactation is prolactin. Medications that raise prolactin levels can be used to help induce lactation. For example, metoclopramide, 10 mg 3 times daily, will cause an increase in prolactin secretion. When metoclopramide is coupled with nipple stimulation every few hours using an electronic breast pump, lactation can be established in the majority of women. In many cases, the amount of milk produced may be insufficient to breastfeed the baby exclusively. Agents such as sulpiride (50 mg daily) and chlorpromazine can also be used to induce lactation, but these agents may be associated with drowsiness and extrapyramidal symptoms.
Recombinant prolactin has been reported to effectively treat insufficient milk production in mothers with documented prolactin deficiency and mothers who cannot pump sufficient quantities of breast milk to feed their preterm children. At a prolactin dose of 60 µg/kg administered subcutaneously every 12 hours, prolactin levels and breast milk volume significantly increased in both groups of women. The prolactin treatment also caused breast milk lactose and calcium concentrations to increase.
Breastfeeding and Endometriosis
- ◆
Breastfeeding may reduce the mother’s risk of endometriosis, type 2 diabetes mellitus (T2DM), heart disease, breast cancer, and ovarian cancer.
Women who are exclusively breastfeeding and amenorrheic have circulating estradiol levels in the range of 20 to 40 pg/mL and progesterone concentrations less than 3 ng/mL. In contrast, normally cycling women have a preovulatory estradiol concentration in the range of 250 to 300 pg/mL and peak progesterone concentration greater than 10 ng/mL. Endometriosis is stimulated by estradiol and prolonged breastfeeding, by reducing estradiol levels, may influence the risk of developing endometriosis. In one study of the relationship between lactation and the risk of developing laparoscopically proven endometriosis, a lifetime duration of lactation of greater than 23 months resulted in an 80% decrease in the risk of being diagnosed with endometriosis. The protective effect of lactation was no longer apparent 5 years after the woman’s last birth.
Breastfeeding and the Risk of Breast Cancer and Ovarian Cancer
Breast cancer risk increases with age, nulliparity, early age at menarche, late menopause, late first full-term pregnancy, and alcohol consumption. Lactation is associated with a reduced lifetime risk of developing breast cancer ( Fig. 10.3 ). The magnitude of the benefit is increased with prolonged duration of breast feeding. Parity is an exposure that confounds the relationship between lactation and reduced risk of breast cancer. For every 12 months of breastfeeding, there is an approximately 4% reduction in the relative risk of breast cancer and each live birth is associated with a 7% reduction in the risk of breast cancer. Breastfeeding appears to reduce the risk of estrogen receptor-negative breast cancer but not the risk of estrogen receptor-positive breast cancer. In contrast, increasing parity reduces the risk of estrogen receptor-positive breast cancer, but not estrogen receptor-negative cancer. This suggests that lactation and parity work through distinct biologic mechanisms.
