Principles of dose, schedule, and combination therapy
William N. Hait, MD, PhD Joseph P. Eder, MD
Overview
The discovery and development of oncology drugs are complex and associated with a high failure rate. For example, the chance of a new drug that enters clinical trial has an approximately ten percent chance of achieving regulatory approval and ultimately becoming available for patients. Determining the proper dose and schedule of a drug are arguably the two most important determinants of both safety and efficacy, the primary determinants of regulatory approval. Understanding how to best estimate the proper dose and schedule for the many types of therapeutic agents now widely available and in development is a critical skill for those in the drug discovery and development field. This area of expertise has been made more complex with the addition of biological agents such as monoclonal antibodies, alternative protein scaffolds, and vaccines to that of hormones and small molecule platforms. In this chapter, we review the basic principles that underlie the appropriate selection of dose and schedule with a major focus on cytotoxic chemotherapy. In addition, we discuss the many variables that can underlie the dose-response relationship including characteristics of the tumor, the tumor microenvironment, the host including enzymes of drug metabolism and mechanisms of drug clearance. Finally, we review the clinical trial designs that have been successfully used to properly select dose and schedule for oncology drugs.
Introduction
The identification of novel, clinically active agents has been central to progress in cancer chemotherapy. Table 1 presents examples of new agents and of cellular pathways and targets being explored for new therapeutic targets. Dose is a significant determinant of the antitumor activity and toxicology for the established, “classical” cytotoxic chemotherapeutic agents.1 These agents are DNA damaging agents, directly or indirectly, or inhibitors of cell division, and a quantitative relationship between target interaction and cell lethality is unknown. High-dose chemotherapy (4–10 times the baseline dose), made possible by hematopoietic stem cell transplantation, has proved curative for selected hematologic neoplasms. The FDA is quite explicit in the centrality of the dose–response relationship of any agent for any purpose “Exposure-response information is at the heart of any determination of the safety and effectiveness of drugs. That is, a drug can be determined to be safe and effective only when the relationship of beneficial and adverse effects to a defined exposure is known.”2
Table 1 Molecular targets for cancer treatment
The cell cycle
|
Differentiation
|
Apoptosis
|
Angiogenesis
|
Signaling cell surface receptors
|
Metastasis
|
Intracellular signaling elements
|
Nuclear transcription factors
|
Potential targets
|
Cell surface antigens
|
The effect of dose for biologically therapeutic agents such as the interferons, interleukins, monoclonal antibodies, hormones, and for molecularly targeted tyrosine kinase inhibitors is complicated, and there is not the same unequivocal evidence for a dose–response effect with these agents. Contemporary targeted agents have a much more specific relationship to the extent of target interaction, such as receptor occupancy (RO) for monoclonal antibodies or extent of phosphorylation inhibition for kinase inhibitors (proof of mechanism) and a measured pharmacodynamics effect (proof of principle), such as phosphorylation inhibition expected to correlate with clinical efficacy in the proper molecularly selected patient segment (proof of concept) than dose alone implies, although in clinical medicine this is the surrogate used.
The schedule of drug administration may be important to the therapeutic index independent of dose. Cytokinetic studies related to drug schedule have led to the improved use of agents such as cytosine arabinoside (cytarabine, ara-C) in both experimental and clinical leukemia (see the section titled “Cytokinetics of bone marrow”).3, 4 Most of the molecularly targeted agents, whether small molecules or monoclonal antibodies, are dosed to provide a continuous effect, which markedly changes the clinical toxicity profile.
Combination chemotherapy has been crucial in the development of curative regimens for hematologic malignancies, pediatric solid tumors, testicular cancer, and ovarian cancer and for the adjuvant regimens for breast, lung, and bowel cancer, and for osteosarcomas.5, 6 The rationale for combination chemotherapy is discussed under the various topical headlines. The principal rationales include (1) the empiric: almost all therapy that has proven curative in the clinic involves the use of agents in combination (Table 2); (2) the fact that genetic instability results in tumor cell heterogeneity, which manifests as drug resistance in cancer therapy6–8; and (3) signal transduction inhibitors molecularly targeted to specific cancer driver mutations, with the possible exception of imatinib in some patients with chronic myelogenous leukemia (CML),9 invariably induce resistance that can be circumvented by either combining a second agent (dabrafenib and trametinib in melanoma)10 or using second-generation agent with a broader spectrum of targets specific for the mutations conferring resistance which develop (ceritinib in EML4/ALK mutant NSCLC)11, 12 to have significant therapeutic benefit. Although dose and combination chemotherapy are generally considered separately, they have an important and complex relationship.13, 14 There is an impressive increase in the number of putative molecular targets for cancer treatment in development (Table 1). A major clinical research challenge will be not only to maximize the effectiveness of individual agents but also to integrate drugs into optimal combination strategies.
Table 2 Number of agents and curative treatment for childhood acute lymphoblastic leukemia (ALL)
Number of chemotherapeutic agents | ||||||||
1 | 2 | 3 | 4 | 5 | 6 | 7 | 8 | |
Year | 1948 | 1954 | 1956 | 1960 | 1965 | 1974 | 1985 | 1988 |
Agent | Methotrexate | MP | Prednisone | Vincristine | Methotrexatea | Adriamycin | Asparagine | ara-C |
CR (%) | 20–40 | 40–92 | 80–95 | >95 | >95 | >95 | >95 | >95 |
Cure (%) | 0 | 0 | 0 | 15 | 5–35 | 55 | 75 | 80 |
a Intrathecal methotrexate.
Abbreviations: CR, complete response; MP, 6-mercaptopurine.
Dose
In controlled experimental systems, such as established tumor cell lines in culture, the relationship between dose and tumor cytotoxicity may be close to linear-log (i.e., exponential).15 For example, a linear increase in the dose of selected chemotherapeutic agents causes a log reduction of MCF7 human breast cancer cells in culture.16 When the dose is expressed as multiples of the IC90 (i.e., the dose or concentration that reduces the number of tumor cells by 90%), a very good response in terms of tumor regression in a patient is obtained. The estimated total tumor burden for patients with clinically evident cancer is 5 × 1011 ± 101 (11 ± 1 logs). Thus, a dose that produces a good partial remission (e.g., 50–90% tumor regression) produces at most a 1-log reduction, which is <10% of the “exponential iceberg.” Numerous factors influence the dose effect. They are presented in the subsections that follow.17, 18
Factors influencing the dose effect
Class of antineoplastic agent
The ideal therapeutic agent would maintain a linear relationship between dose and log tumor cell reduction (log-TCR) down through multiple logs of tumor cell death. Ionizing radiation comes closest to this ideal. As a group, alkylating agents maintained dose/log-TCR superior to the other chemotherapeutic agents. DNA damaging alkylating agents exhibit major activity during the S and M periods of the cell cycle, but unlike other chemotherapeutic agents, they maintain activity throughout the cell cycle. Although comparative studies demonstrate a dose effect in chemotherapy-sensitive tumors such as the leukemias and lymphomas, the effect of dose is less evident in solid tumors, particularly those tumors of epithelial origin.19, 20 Purine- and pyrimidine-targeted antimetabolites are active mainly in proliferating cells, and therefore pharmacokinetic resistance occurs that is overcome less by increasing dose than by increasing the duration of exposure, allowing more cells to enter the proliferative compartment. This also applies to the DNA-damaging topoisomerase I and II directed agents and microtubule-interacting agents, where the target must be encountered in the setting of DNA synthesis or mitotic spindle assembly to produce antineoplastic cytotoxicity.21, 22
Agents directed at hormone receptors, growth factor receptors and intracellular kinase signaling targets have a different relationship as once receptor/kinase interactions are saturated, further dose increases will produce no further effect.23 Hence, these agents are analogous to antimetabolites in that dose escalation once saturation levels of drug are achieved produces no further benefit. Higher doses of imatinib in chronic phase or accelerated phase CML with a suboptimal response to standard dose can benefit a subgroup of patients, but the percentage is low and the duration of response brief.24, 25
Tumor factors
Intrinsic tumor cell sensitivity
The more sensitive the tumor is to a given agent, the steeper the dose effect. Thus, if a unit dose produces a 0.5 log-TCR, then doubling that dose may produce up to a 1.0 log tumor cell kill—which clinically represents only a partial remission. In a chemotherapy-sensitive tumor, where a unit dose produces a 3 log-TCR, doubling the dose may produce up to a 6 log-TCR, depending on the degree of tumor cell heterogeneity and drug resistance (see the section titled “Drug resistance”). A 6 log-TCR would produce a major clinical achievement in terms of complete response, duration of complete response, and, most important, an approach to tumor cure or eradication. Thus, for patients with a metastatic common epithelial tumor, such as breast cancer, the most that can be achieved with standard single-agent chemotherapy is a partial response (<1 log-TCR) in about 30% of patients. Combinations result in higher partial response rates and a low (10–20%) complete response rate. Alternatively, combination chemotherapy regimens in patients with chemotherapy-sensitive tumors (e.g., non-Hodgkin’s lymphoma, Hodgkin’s disease, and germ cell tumors) may achieve multi-log-TCR as a result of combination chemotherapy.26
Tumor burden
Tumor burden is a consistent adverse prognostic factor for response to chemotherapy. This finding was first demonstrated for transplanted tumors in mice; in these animals, macroscopic (i.e., palpable) tumors often respond minimally to chemotherapy. The same tumor, at a microscopic tumor burden size, may be much more responsive and potentially curable.26, 27
These observations in mice are consistent with the parallel observation that adjuvant cancer treatment can be curative for patients with breast cancer but not for those with overt metastatic breast cancer. Postulates for the delay in growth of microscopic metastases include a balanced rate of cell loss (i.e., apoptosis) and cell production and inability to support tumor neovascularization (angiogenesis). Resistant microscopic tumor may persist in most long-term survivors, an observation that has major implications for therapeutic strategy (see the section titled “Cytokinetics of the tumor—The growth fraction”).
The study of microscopic metastases in patients may become increasingly possible with molecular techniques for detection and characterization of minimal residual tumor.26 The kinetics of microscopic disease can be inferred from adjuvant chemotherapy studies (see the section titled “Adjuvant chemotherapy”).
Drug resistance
In the laboratory, drug resistance is usually produced by “selection pressure”—that is, by exposing target cells to progressively increasing concentrations of the selecting agent. Drug resistance is usually expressed as the concentration of drug that is required to produce 50% inhibition in a colony or growth assay (IC50) for the resistant cell line, divided by the concentration required (IC50) for the parent sensitive cell line. For a detailed presentation of drug resistance, see the section titled “Combination chemotherapy” and 63.
Cytokinetics of the tumor—The growth fraction
The growth fraction (GF) of the tumor and the dose of cell cycle-specific agents have a major effect on the log-TCR of tumor cells. The generation time of cycling (i.e., mitotically active) cells is much shorter than the volume doubling time.28–32 Thus, many cells within tumors are dying or “noncycling”—that is, in G0/G1. The GF of a tumor is the ratio of the cycling cells to the total number of tumor cells.
For the common epithelial solid tumors, the GF is often <5%.33, 34 A solid tumor with a GF of 5% would be minimally responsive to cell cycle-specific agents and variably sensitive to other chemotherapeutic agents. Repetitive treatments, however, might “recruit” cells into cycle by allowing dormant, noncycling cells access to necessary growth conditions and thus enabling them to be more effective. Prolonged exposure to cell cycle-specific agents might be effective in low—GF tumors. In contrast, a high-GF tumor such as Burkitt’s lymphoma might have a multilog response with the same treatment or even the same dose over a shorter period.1 A recent challenge to the long-standing clonal evolution model of cancer evokes specific cancer progenitor cells (CPCs) as responsible for the continued proliferation of a tumor. By this model, self-renewing CPCs give rise to all progenitor and differentiated cells within a tumor but remain a small proportion of all tumor cells.35 CPCs, as with normal tissue stem cells, are extremely resistant to chemotherapy and radiotherapy.36 The difference between curative therapeutic regimens and those that are only palliative may be attributable to the relative sensitivities of CPCs and the progenitor and differentiated cancer cells incapable of self-renewal. Identification of therapeutic targets within the CPC population should offer significant opportunities for more effective therapies.
Tumor hypoxia
Hypoxia commonly occurs in both experimental and clinical solid tumors, a condition presumably resulting from inadequate angiogenesis and high metabolic activity (oxygen consumption). Oxygen distribution within tumors is heterogeneous and even a small fraction of hypoxic cells can profoundly affect chemotherapy responsiveness. The farther cells are from blood vessels, the lower the concentration of chemotherapeutic agents in those cells. Cellular proliferation decreases as a function of distance from blood vessels, with a significant fraction of nonproliferating cells conferring kinetic resistance to cell cycle-specific agents. Certain cancer chemotherapy agents require oxygen as an intermediate in toxicity or metabolism.37 Finally, hypoxia produces altered gene expression. Hypoxia-inducible factor 1 (HIF1) stops proliferation and prevents apoptosis in the hypoxic fraction of cells by increasing angiogenesis. Hypoxia increases the production of hepatocytes growth factor (HGF) and its receptor MET, with a further increase in angiogenesis, and increased incidence of metastasis and drug resistance.38, 39 There may be increased expression of adenosine triphosphate-binding cassette (ABC) proteins such as p-glycoprotein (PgP) that may confer resistance to chemotherapeutic agents. Hypoxia also selects for TP53 mutants with a reduced apoptotic response to DNA damage or cell cycle arrest targeted agents.40 Exploiting hypoxia as a target in cancer therapy with bioreductive alkylating agents such as mitomycin C and the nitroimidazoles, which can serve as electron acceptors in lieu of oxygen, has been tried in numerous clinical circumstances with no or minimal benefit.41, 42 Impaired chemosensitivity is not universal, and in selected cell lines (i.e., renal), some drugs appear even less effective in normoxia than hypoxia.43 Radiotherapy in particular requires molecular oxygen for cytotoxicity.44
Oncogene addiction-growth factor signaling
The maintenance of the transformed state results in significant metabolic and genetic stress on cancer cells. Maintaining viability under these conditions requires positive anti-apoptotic signaling factors and interfering with these survival pathways can result in tumor cell lethality. In particular, mutant oncogenes such as BCR-ABL in CML, or mutant growth factor receptors such as EGFR (ERBB1) in lung cancer, BRAF in melanoma, and CKIT in gastrointestinal stromal tumors (GIST) provide essential survival signals and interruption of these pathways produces significant clinical benefit in affected patient. The emergence of diagnostic molecular testing enables identification of patients and permits selection of appropriate therapy.
Host factors
Cytokinetics of bone marrow
Because of the bone marrow’s proliferative activity and relative lack of DNA repair capacity, myelosuppression is dose limiting for many chemotherapeutic agents. Exploiting the cytokinetic difference between marrow and tumor has been a basis for the construction of selected clinical strategies.4, 28
Normal marrow recovers within 1–2 weeks after cytarabine, with little cumulative myelosuppression. For many patients, recovery of acute myelogenous leukemia (AML) cells as compared with normal marrow cells between courses of cytarabine is incomplete. AML cells in vitro are less susceptible to growth factors such as G- and GM-CSF than are the cells of the normal marrow.45 Thus, when marrow CSFs increase in homeostatic response to cytarabine-induced myelosuppression, the interval recovery of normal marrow may be more rapid than that demonstrated by the AML cells, a factor that should, with successive dosing, result in a cumulative effect—the therapeutic advantage.
Similar changes occur in other proliferative tissues, including the gastrointestinal tract, where drugs cause mitotic arrest with loss of epithelial surface cells, including cells involved in the adsorption of fluids. This requires a recovery period just as the marrow does.46
Pharmacokinetics
Pharmacokinetic factors commonly affect the dose–response curve. If an inactivating enzyme for the drug becomes saturated, both toxicity and antitumor effect may increase disproportionately, an effect observed with certain dose schedules of 5-fluorouracil (5-FU) for example.47, 48
The opposite effect may occur if a drug activation system becomes saturated. Ifosfamide, a prodrug, is activated by the cytochrome P450, oxygen-dependent, drug-metabolizing enzymes in the liver to the biologically active 4-hydroxyl derivative. The 3-day conventional dose of ifosfamide is higher (1200 to 2400 mg/m2 daily) than that for cyclophosphamide (600 mg/m2) because the rate of P450 activation of ifosfamide is relatively slow. With increasing doses of cyclophosphamide, a constant fractional conversion to active 4-hydroxyl cyclophosphamide occurs. However, for ifosfamide, once the P450 enzyme system becomes saturated, a decreasing proportion of ifosfamide is converted into the active form, with a consequent loss of antitumor effect at higher doses.37
Clinical trials and the dose effect
Dose selection in patients
Clearance determines the total drug exposure (area under the curve of concentration multiplied by time [AUC]), and AUC in mice correlates with toxicity in that species. This relationship of AUC with drug exposure and toxicity also holds true in humans and can be predicted from mouse data.49 The process for initial dose selection in phase I clinical trials is detailed in 45. In most circumstances, the dose is determined in phase I trials and in other situations is individualized to the body surface area (BSA), weight or exposure (AUC) of the patient.
The Dubois BSA formula is useful in allometric scaling of drug-dose selection between species. The expectation was that similar adjustments would reduce the variability in clearance between patients and is often used in determining the initial dose in first time in human (FTIH) trials. BSA may be helpful in selecting the dose of cytotoxic agents in childhood leukemia and was subsequently incorporated into standard usage.50, 51 Recent reviews of the literature and individual institutional experience can find no significant correlation between BSA and clearance variability with investigational or commonly used anticancer drugs except for paclitaxel, oral busulfan, and possibly temozolomide.52, 53
BSA may correlate with glomerular filtration rate, blood volume, and basal metabolic rate.52 However, the variability in drug clearance introduced by these factors is small (<25%) compared with that induced by hepatic metabolic enzymes, and there is no correlation between BSA and metabolic activity.52
Cytochrome P450 3A4 (family 3, subfamily A, and polypeptide 4) is the most prevalent metabolic enzyme in humans and is responsible for more than 55% of drug metabolic clearance.54 Recent studies utilizing noncancer drugs as indicators of CYP3A4 metabolic activity, such as midazolam clearance,55 suggest potential clinical utility with agents that are metabolized by this pathway—for example, docetaxel.56 Enzymatic pathways are responsible for the clearance of 5-FU and 6-mercaptopurine (6-MP) and for glucuronidation via UGT1A1 for clearance of SN-38, the active product of irinotecan (see individual chapters). Each of these enzymes has a significant incidence of polymorphisms that affect drug disposition and correlate with toxicity. Persons with the UGT1A1*28 homozygous 7/7 genotype have a reduced capacity for glucuronidation as the major metabolic pathway of SN-38 and higher drug exposure (AUC). Several studies have confirmed a significant correlation among the SN-38 exposure, 1A1*28 genotype, and severe neutropenia and diarrhea.57 Pharmacogenetic profiling offers the prospect of individualized dose selection in the future and is a frequent component in early clinical drug development. These DNA-based tests are not commercially available yet and have no clinical role at the present time.
Glomerular filtration rate as estimated from serum creatinine does correlate with toxicity for topotecan, etoposide, and carboplatin. Indeed, calculated AUC from serum creatinine (Calvert formula) is now used to dose carboplatin.51 At present, these alternatives (except for carboplatin AUC dosing) remain under investigation, and BSA-based dosing remains the standard of clinical practice for classical chemotherapy agents.
All the molecularly targeted tyrosine kinase inhibitors approved for clinical use as well as the many more in clinical development are administered as a flat dose with no adjustment for weight or BSA. Dose selection of monoclonal antibodies used in cancer medicine also does not follow a classical dose escalation to toxicity. RO of a surface glycoprotein target is a quantitative measure of an appropriate dose of agents directed at surface proteins (i.e., anti PD 1 immune checkpoint agents) or depletion of a circulating protein (VEGF (vascular endothelial growth factor) and bevacizumab).
Real-time pharmacokinetics and patient safety
Pharmacokinetic studies provide important information regarding the dose effect. Such studies indicate substantial variation in serum drug levels and in the AUC per given dose. For methotrexate and 6-MP in acute leukemia and for high-dose busulfan and carmustine bis-chloroethylnitrosourea (BCNU) in the transplant setting, the AUC level of drug or its active metabolites (or both) correlates with toxicity and therapeutic effect.58–61
Plasma levels of methotrexate used in high doses (>1 gm/m2) in selected settings of ALL or DLBCL are clinically tested and are used to determine the duration and dose of leucovorin rescue in these patients.62 Substantial variation in the AUC per given dose of paclitaxel was also observed in patients with solid tumors. Real-time adjustment of dose on subsequent days significantly reduced mucositis requiring morphine administration and decreased the duration of hospital stay.63
Dose effect in sensitive tumors
Few clinical studies have included dose intensity as an independent, randomized variable. In a Cancer and Leukemia Group B (CALGB) study, 596 patients with AML were randomized to receive four 5-day courses of cytarabine at one of three dose schedules: (1) 100 mg/m2 daily (standard arm), (2) 400 mg/m2 by continuous infusion, or (3) 3 g/m2 in a 3-h infusion every 12 h (twice daily) on days 1, 3, and 53. For patients 60 years of age or younger, the probability of remaining in continuous complete remission after 4 years was 24% in the 100 mg/m2 group; 29% in the 400 mg/m2 group; and 44% in the 3 g/m2 group (p = 0.002), indicating a better response with increased dose. Elderly patients were less responsive. In acute lymphocytic leukemia (ALL), the dose rate of maintenance chemotherapy had a major impact on the duration of response.64 Similarly, in studies of combination chemotherapy in small cell lung cancer, the dose effect was significant, albeit with outmoded therapy.65
Increased doses of anthracycline combined with standard dose cytarabine in the initial therapy of patients with AML resulted in an increase in CR rate and PFS (progression free survival) in both young and elderly patients.66
Dose–response effects are less well studied in the new kinase inhibitors, which tend to be dosed at the maximum tolerated daily dose, leaving little opportunity for significant increases. Increasing doses of imatinib in patients with chronic phase or accelerated phase CML and in GIST show an increase in response rate in a subgroup of patients. In cases where resistance to imatinib is due to increased metabolic clearance, increased activity of PgP or amplification of the BCR-ABL gene, this might be expected although responses were seen even in patients with mutations in the BCR-ABL kinase itself.24, 25, 67, 68
In treating individual patients, dose is a key factor if cure is possible. Thus, for leukemias, lymphomas, testicular cancer, childhood solid tumors, and conventional-dose adjuvant treatment of breast cancer, dose should not be compromised even at the risk of significant toxicity. For more resistant tumors, where palliation is the goal, dose should be adjusted primarily on the basis of toxicity.
Peripheral blood stem cell and marrow transplantation
Allogeneic bone marrow transplantation produces disease-free survival plateaus (i.e., cures) in patients with acute and chronic leukemias and lymphomas, but because of the effect of graft versus leukemia, the component contributed by dose cannot be independently evaluated.
The most compelling evidence regarding dose–response is in high-dose, autologous stem cell rescue studies in patients with relapsed lymphoma.69, 70 Alkylating agents and total body radiotherapy-based regimens are commonly used because their dose-limiting toxicity is myelosuppression. Depending on the agent, dose can be escalated between 3 and 20 times baseline before nonmyelosuppressive toxicity becomes dose limiting. Given the considerable overlap of AUCs for serum levels of drugs at dose escalations of 2–4 times baseline, the escalations possible with stem cell support allow better comparisons of the effect of dose. High-dose therapy with autologous stem cell rescue produces high complete response rates and cures in non-Hodgkin’s lymphoma and testicular cancer.70, 71 However, because toxicity can be lethal, high-dose therapy should be limited to specialized centers.72
Adjuvant chemotherapy
Randomized studies
Cytokinetics provides an experimental basis for many therapeutic designs. A brief review of the related history follows.
Skipper et al.15 established the fundamental exponential relationship between drug treatment and surviving tumor fractions. It is the fractional TCR that is constant for a given dose and drug. Although this exponential relationship is modified by other factors, such as drug resistance and microenvironment, it remains the fundamental tenet of cytokinetics and chemotherapy. Norton and Simon73 applied Gompertzian theory and analysis to treatment during remission and demonstrated the potentially greater effectiveness of late intensification. Goldie and Coldman74 introduced the mutation-to-resistance theory, relating tumor burden and inherent mutation rate to potential for cure. Hryniuk and colleagues found a significant dose–response effect not only in the leukemias and lymphomas but also in the relatively less chemosensitive tumors, such as breast cancer.75–77
The adjuvant setting, where the tumor burden is microscopic, should be ideal for demonstrating a dose effect. There, many factors that could reduce tumor cytotoxicity (tumor size, decreased and abnormal vascularity, low GF, hypoxia, and increased tumor heterogeneity) and contribute to chemotherapy resistance are less evident in the microscopic tumor (adjuvant) setting. Combination chemotherapy with cyclophosphamide, methotrexate, and 5-FU (CMF) or with cyclophosphamide, doxorubicin (Adriamycin), and 5-FU (CAF), which produces only transient partial and a few complete responses in metastatic breast cancer, reduces relapse and mortality rates by 20–30% in the adjuvant breast cancer setting.78 Similar effects are seen in colon cancer.79
Attempts to improve disease-free survival by increasing the adjuvant chemotherapy dose in breast cancer have produced mixed results. The first statistically robust positive study was conducted by the CALGB.19 Patients with node-positive breast cancer were randomized to one of three CAF regimens. The high-dose arm involved four courses of CAF at doses of 600 cyclophosphamide, 60 doxorubicin, and 600 mg/m2 5-FU every 3–4 weeks; in the low-dose arm, the doses were 300, 30, and 300 mg/m2, respectively. A 10% difference in the relapse-free curve developed by 2 years and persisted through 10 years. That result represents an approximately 20% reduction in mortality. The dose effect was seen most prominently in the 20% of patients whose tumors overexpressed ERBB2 (HER2/neu). For tumors without ERBB2 overexpression, no significant dose effect was seen.20, 80, 81 This subset effect would not have been identified in the absence of the molecular marker.
Two other studies conducted by the National Surgical Adjuvant Breast and Bowel Project (NSABP) failed to show that a 2 or 4 times increase in the dose of cyclophosphamide alone affected response in terms of relapse or survival in adjuvant breast cancer.80, 81 Thus, in the comparative study of CAF, the dose of doxorubicin was probably important; however, in another study of adjuvant breast cancer, patients randomized to three doses of doxorubicin82(60, 75, and 90 mg/m2, all given with the standard dose of cyclophosphamide) showed no difference in disease-free or overall survival (OS). The 60 mg/m2 is probably a threshold dose, above which no further benefit accrues.
The basis for the seemingly discordant results of dose in major clinical trials has been the subject of preclinical and mechanism-of-action studies, but it remains unexplained. It can be speculated how often in the analysis of large comparative studies an important effect has been missed within a subset not known to exist at the time. That possibility is an important limitation in the interpretation of negative studies.
Dose-dense chemotherapy
The concept behind dose-dense chemotherapy is to increase the intensity of drug administration by shortening the interval between treatments without increasing the total dose of drug administered. This increase is accomplished by escalating the dose or the number of cycles in a given period of time. The use of neutrophil-colony-stimulating factors is an essential requirement for dose-dense therapy. Interim reports on the CALGB 9741 study, the use of dose-dense therapy with cyclophosphamide, doxorubicin, and paclitaxel in adjuvant breast cancer, either concurrently or sequentially in 2-week as opposed to the standard 3-week cycles significantly reduced the annual risk of recurrence or death. The DFS (risk ratio [RR] = 0.74, P = 0.10) and OS (RR = 0.69, P = 0.013) were prolonged in the dose-dense arms. There was no difference in either DFS or OS between sequential and concurrent therapy. There was no interaction between dose-density and sequence. This preliminary report supported the concept of dose-dense intensification as a means to enhance chemotherapy efficacy, albeit at a cost of increased toxicity.82 Definitive randomized trials have not confirmed these initial reports, although not all the studies are completed.83
Summary
In the clinic, the effect of dose correlates generally with the basic chemosensitivity of the tumor. Thus, Burkitt’s lymphoma, ALL, and testicular cancer are all highly sensitive and highly responsive to dose intensity, including achievement of cure. On the other hand, relatively insensitive tumors such as gastrointestinal and lung cancers respond poorly to chemotherapy and are not significantly affected by dose. There are clearly unknown factors at work in the human cancer patient, which continue to defy simple explanation, among them the complex milieu of the inherent genetic background in the particular cell type and patient in which cancer arises, the effect of somatic and nontransformed stromal cells, the emerging role of cancer stem cells and other unappreciated factors not accounted for by the reductionist in vitro and in vivo
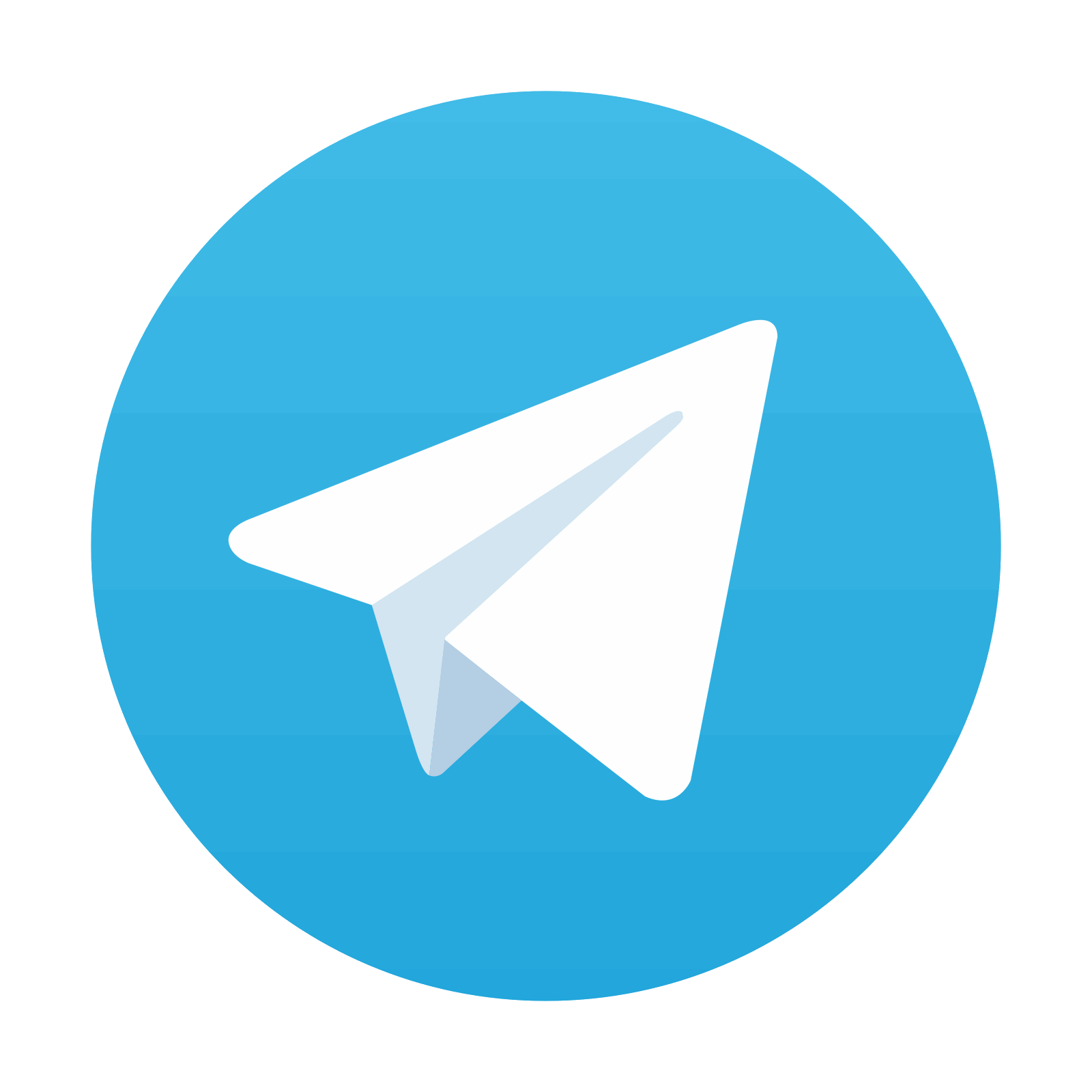
Stay updated, free articles. Join our Telegram channel

Full access? Get Clinical Tree
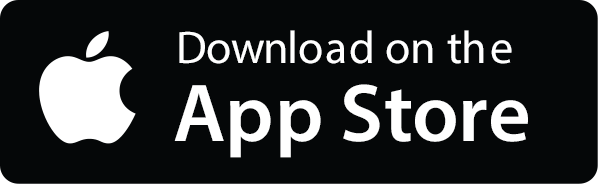
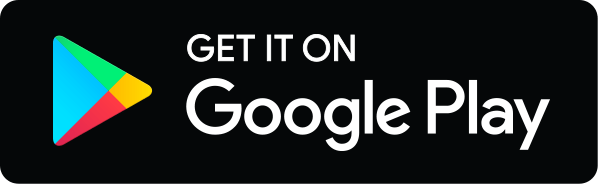