Visit the Endocrine Hypertension: From Basic Science to Clinical Practice , First Edition companion web site at: https://www.elsevier.com/books-and-journals/book-companion/9780323961202 .
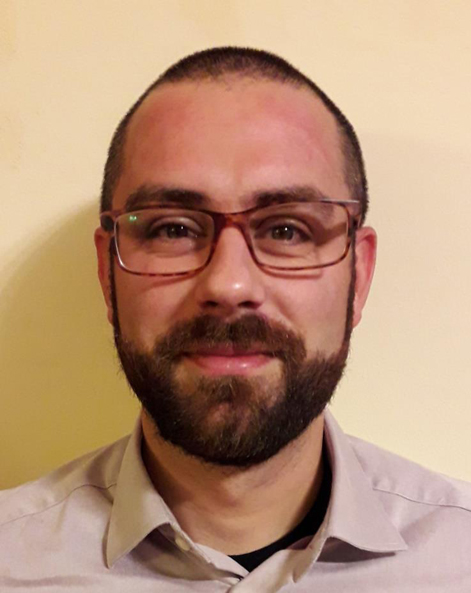
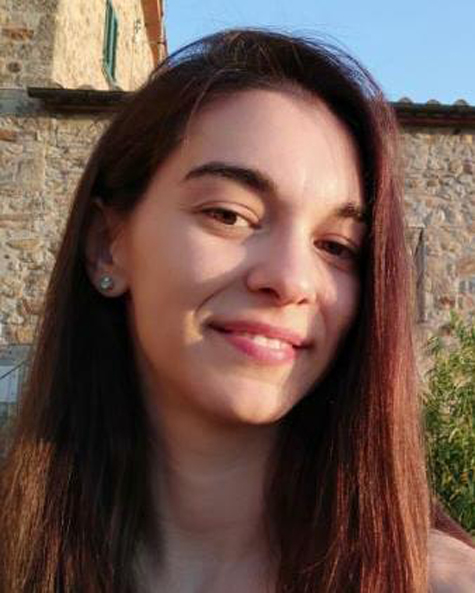
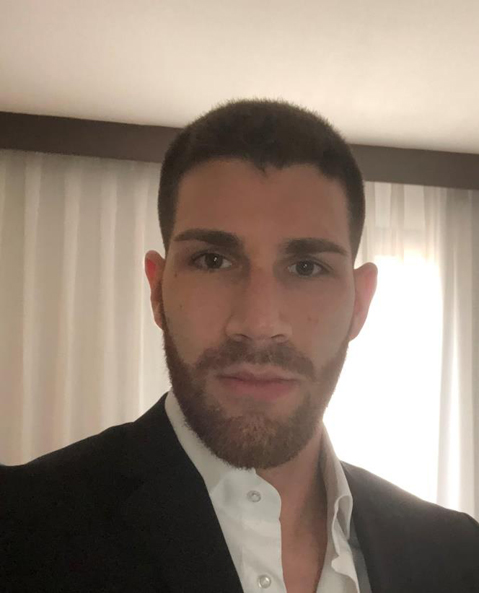
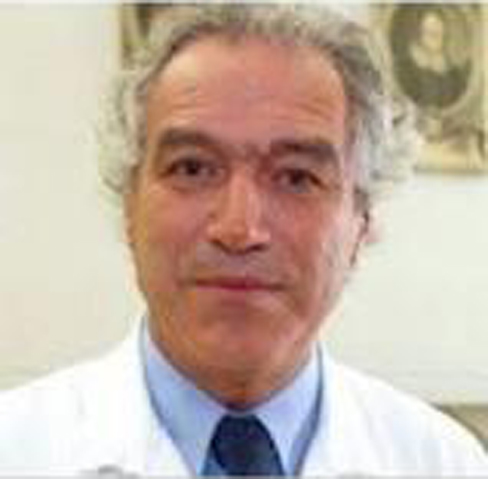
Introduction
Hypertension (HTN) is a highly prevalent condition in the general population (up to 30%–40% in large epidemiological studies, with more than 1 billion adults affected globally) [ , ], with persistently high incidence in central and eastern Europe, and from high-income countries to low-income countries [ ]. The close relationship between blood pressure (BP) and cardiovascular (CV) events is continuous, thus complicating the distinction between normal BP levels and
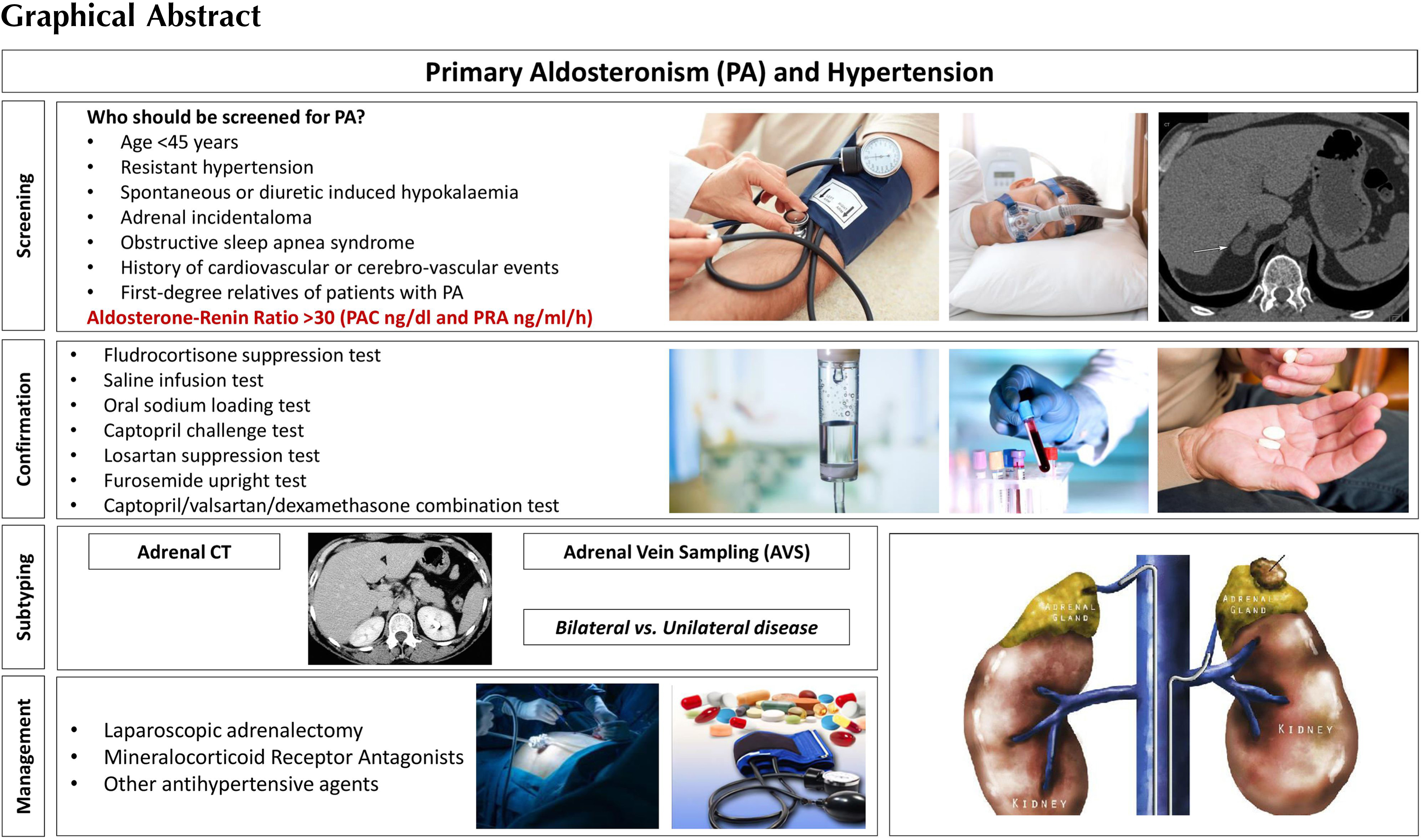
Primary aldosteronism (PA) is the most common form of endocrine hypertension; its prevalence is up to 10% among hypertensive patients and up to 20% of those with resistant hypertension [ , ]. PA is not a single disease, it should be considered as a group of disorders characterized by an inappropriately high aldosterone production for sodium status, not controlled by renin–angiotensin or potassium levels. Such inappropriate production of aldosterone causes sodium retention, hypertension, CV damage, and hypokalemia [ , ].
Common causes of PA are adrenal adenoma (also termed as Conn’s disease/syndrome, first described by Jerome Conn in 1955 [ ]), unilateral or bilateral adrenal hyperplasia, and in rare cases adrenal carcinoma or familial hyperaldosteronism. Patients with PA present an increased risk for cerebrovascular and CV events [ ], due to target-organ damage, higher than in the matched group of patients with essential hypertension [ , , ]. Moreover, glucose metabolism is also impaired in patients with PA, due to increased prevalence of diabetes and metabolic syndrome, with a further increase of the CV risk [ ]. This chapter discusses the pathophysiology, clinical picture, diagnostic evaluation and management of PA.
The renin–angiotensin–aldosterone system
The differentiation of the adrenal cortex into distinct zones has important functional consequences and is thought to be dependent on the temporal expression of peculiar transcription factors.
Beneath the capsule, the zona glomerulosa layer comprises approximately 15% of the cortex (depending upon the sodium intake); aldosterone from this layer is the main mineralocorticoid in humans. Briefly, after cholesterol uptake into the mitochondria, it is cleaved by the cytochrome P450 enzyme for cholesterol side chain cleavage (steroidogenic acute regulatory protein) to pregnenolone. In the cytoplasm, pregnenolone is converted to progesterone and then 21-hydroxylase, derived from CYP21A2 gene, performs 21-hydroxylation of progesterone to deoxycorticosterone (in the zona glomerulosa) or 17-OH-progesterone to 11-deoxycortisol (in the zona fasciculata). The final step is controlled by the enzyme CYP11B2 (aldosterone synthase), expressed only in the zona glomerulosa [ ]. The adrenocortical steroidogenesis pathway is shown in Fig. 7.1 .
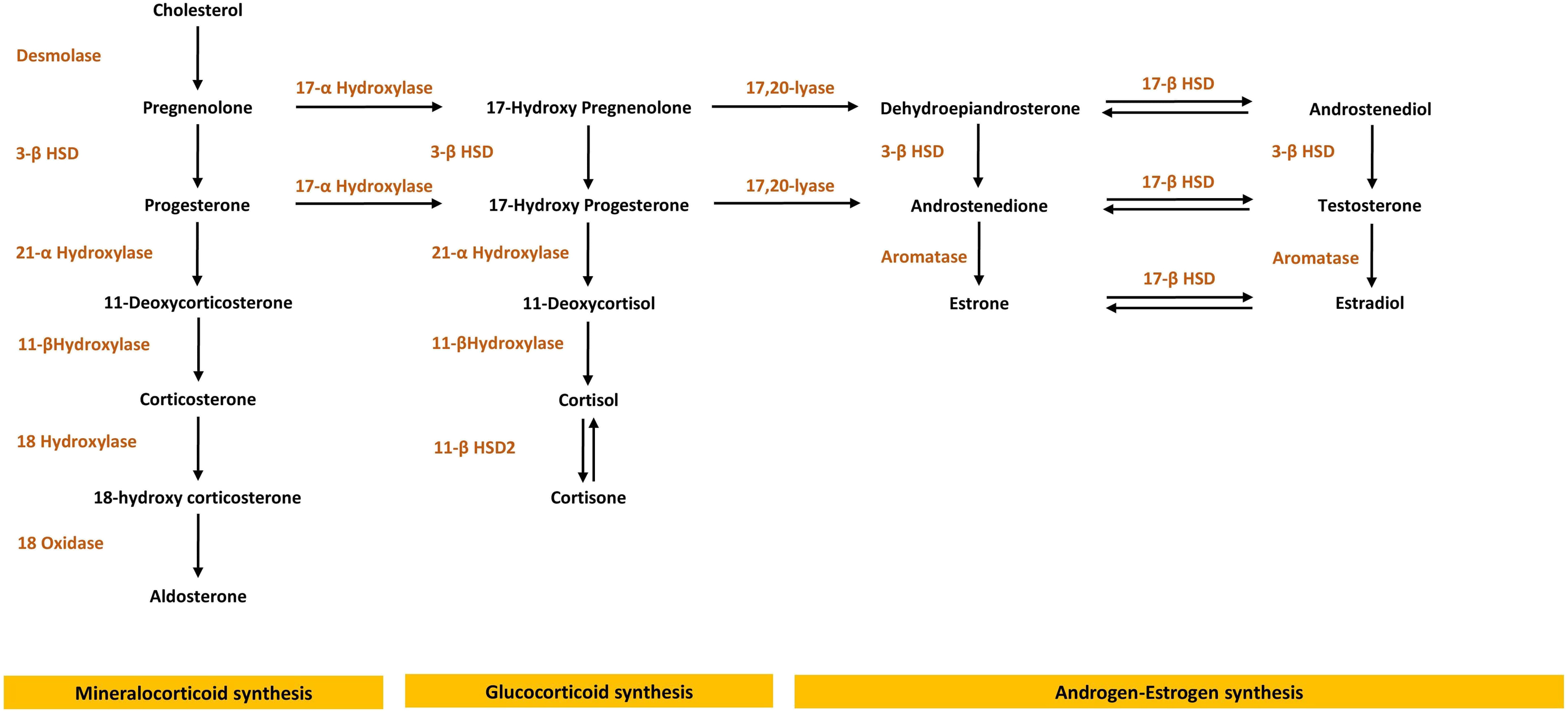
Aldosterone production in the body is controlled by three principal factors: angiotensin II (the so-called renin–angiotensin–aldosterone system, RAAS), potassium, and to a lesser extent by adrenocorticotrophin (ACTH) [ ]. Angiotensin II and potassium stimulate aldosterone secretion principally by increasing the transcription of CYP11B2 through common intracellular signaling pathways, with cyclic adenosine monophosphate (cAMP), intracellular Ca 2+ , and activation of calmodulin kinases. The potassium effect is mediated through membrane depolarization and opening of the calcium channels.
The effect of ACTH upon aldosterone secretion is modest and differs in time: an acute ACTH bolus will increase aldosterone secretion by stimulating the early pathways of adrenal steroidogenesis; on the contrary, chronic continual ACTH stimulation has either no effect, or even can reduce aldosterone production: this inhibitory effect is possibly secondary to receptor downregulation [ ].
50%–70% of aldosterone circulates bound to either albumin or weakly to corticosteroid-binding globulin; 30%–50% of total plasma aldosterone is free, with a short half-life of 15–20 min. Aldosterone is inactivated to tetrahydroaldosterone in the liver. The classical functions of aldosterone are regulation of extracellular fluid volume and control of potassium homeostasis. These effects are mediated by binding of free aldosterone to the mineralocorticoid receptor in the cytosol of epithelial cells, principally in the kidney. Mineralocorticoid receptors have a tissue-specific expression, especially at distal nephron and colon. Aldosterone leads to modification of the apical sodium channel, resulting in increased sodium ion transport across the cell membrane. Glucocorticoids and mineralocorticoids bind equally to the mineralocorticoid receptor: specificity is provided by the presence of a glucocorticoid-degrading enzyme, 11β-hydroxysteroid dehydrogenase type 2, which prevents glucocorticoids from interacting with the aldosterone receptor by converting cortisol to inactive cortisone.
Renin is a 340-aminoacid enzyme produced primarily in the juxtaglomerular apparatus of the kidney, controlled by the macula densa (a group of distal convoluted tubular cells that function as chemoreceptors for sodium) and juxtaglomerular cells (pressure transducers that sense stretch of the afferent arteriolar wall and thus renal perfusion pressure). Also the sympathetic nervous system and potassium levels control renin secretion [ ]. Angiotensinogen, synthesized in the liver, is the substrate for renin and is broken down into angiotensin I. Angiotensin II is formed by cleavage of the two carboxy-terminal peptides of angiotensin I by angiotensin-converting enzyme (ACE), localized to cell membranes in the lung and intracellular granules [ ]. Angiotensin II then stimulates aldosterone secretion.
Aldosterone has additional nonclassic effects primarily on nonepithelial cells. These actions, although probably genomic and therefore mediated by activation of the cytosolic mineralocorticoid receptor, do not include modification of sodium–potassium balance. Aldosterone-mediated actions include the expression of several collagen genes, growth factors, plasminogen activator inhibitor type 1, or genes mediating inflammation. These actions lead to microangiopathy and fibrosis in various tissues such as heart, blood vessels, and kidney. Increased levels of aldosterone are not necessary to cause this damage; an imbalance between the volume or sodium balance state and the level of aldosterone appears to be the critical factor [ ].
For further reading on RAAS, please refer Chapter 5 .
Who should be screened? (The epidemiology of primary aldosteronism)
The diagnostic pathway of PA consists of three main steps: screening tests, confirmatory tests, and identification of PA subtype [ ]. The high-risk category of hypertensive patients that should be screened for PA, recommended by the Endocrine Society (ES) Clinical Practice Guideline [ ] are those with: age <45 years, spontaneous or diuretic-induced hypokalaemia, adrenal incidentaloma, history of CV or cerebro-vascular events, first-degree relatives of patients with PA, resistant hypertension, and obstructive sleep apnea syndrome (OSAS).
In patients with resistant hypertension, the prevalence of PA varies from 11% to 30% [ , ]. The high prevalence of PA in patients with resistant hypertension reflects that the use of mineralocorticoid receptor antagonists (MRAs) would be particularly effective in such cases [ ].
Prevalence of PA is also reported high in patients with hypertension and atrial fibrillation [ ]. Elevated aldosterone levels predispose to atrial fibrillation [ ]. The aldosterone-induced inflammation, myocardial necrosis, cardiac collagen deposition, and fibrosis can induce atrial arrhythmias [ ]. Also diabetic patients are at high-risk for PA: in a recent study published in 2020, 99 out of 256 patients (39%) presented a positive screening test, and 49 (19%) were diagnosed with PA after a confirmatory captopril challenge test [ ].
One of the novel high-risk conditions for PA is OSAS: it is a chronic condition characterized by frequent episodes of upper airway collapse resulting in hypoxemia while sleeping, and can lead to severe complications if left untreated. OSAS prevalence is 3%–7% in the general population, with some groups in the general population carrying a higher risk [ ]. Aldosterone excess may play a pathophysiological part in the relationship between hypertension and OSAS, since higher plasma aldosterone concentrations (PACs) and OSAS were seen in patients with refractory hypertension [ ], and the prevalence of OSAS was higher in subjects with PA than in patients with essential hypertension [ ]. In 2004, Calhoun et al. studied the renin–aldosterone axis in more than 100 patients with OSAS: subjects at high risk of OSAS were almost twice as likely to have PA and tended to have lower renin levels [ ].
For more detailed reading on the relationship between OSAS and PA, please refer Chapter 17 .
Despite the high prevalence of PA in the hypertensive population, its occurrence among patients with an adrenal incidentaloma is relatively low, ranging from 1.5% to 7% only [ , ]. Obviously, adrenal lesions of patients investigated with abdominal imaging in search of the causes of secondary hypertension are not “incidentalomas” [ ]. The question regarding the cost-effectiveness of exploring aldosterone secretion in all patients with adrenal incidentaloma or only in hypertensive subjects is a matter of ongoing debate [ , ]. Subclinical hyperaldosteronism can exist in an adrenal incidentaloma, especially in those with increased diastolic blood pressure levels [ ].
Aldosterone–renin ratio as screening test
Aldosterone–renin ratio (ARR) is recommended as the initial test to screen for PA. ARR has been proposed as a screening test since early 1980s [ ]. Its application is recommended in the current Endocrine Society guidelines [ ] and leads to an increased detection of PA, especially among hypertensive normokalaemic patients. The routine use of ARR as a screening test led to a 5 to 15-fold increase in the PA identification [ ]. A recent metaanalysis in 2021, in which the performance of ARR to detect PA in 4110 participants (ten studies) was evaluated, reported that the sensitivity of ARR ranged from 10% to 100% and the specificity ranged from 70% to 100%, and that no single ARR could be recommended for definitive diagnosis [ ].
The concentrations of aldosterone and renin are affected by several drugs, especially those directed to RAAS, as well as by clinical conditions. Consequently, special attention should be paid about antihypertensive treatment and potassium levels of the patients with suspected PA. In Table 7.1 we describe drugs or other clinical conditions that could affect the measurement of aldosterone and renin.
Aldosterone | Renin | ARR | |
---|---|---|---|
Drugs | |||
Beta-adrenergic blockers | ↓ | ↓↓ | ↑ |
Central agonist (clonidine) | ↓ | ↓↓ | ↑ |
NSAIDs | ↓ | ↓↓ | ↑ |
K+-wasting diuretics | →↑ | ↑↑ | ↓ |
K+-sparing diuretics | ↑ | ↑↑ | ↓ |
ACE inhibitors | ↓ | ↑↑ | ↓ |
ARBs | ↓ | ↑↑ | ↓ |
Renin inhibitors | ↓ | ↓(PRA)↑(DRC) | ↑ ↓ |
Ca 2+ blockers (DHPs) | →↓ | ↑↑ | ↓ |
Ca 2+ blockers (non DHPs) | → | → | → |
Alpha1-adrenergic blockers | → | → | → |
Antiepileptic drugs | → | → | → |
Rifampin, rifapentine | → | → | → |
Pioglitazone | → | → | → |
Itraconazole, ritonavir, fluoxetine, cimetidine, aprepitant | → | → | → |
Estrogens | ↑ | ↑ ↑ | ↓ |
11β-HSD2 inhibitors (licorice) | ↓→ | ↓→ | ↓→ |
Potassium status | |||
Hypokalemia | ↓ | ↑ | ↓ |
Potassium loading | ↑ | → ↓ | ↑ |
Dietary sodium | |||
Sodium restriction | ↑ | ↑↑ | ↓ |
Sodium loading | ↓ | ↓↓ | ↑ |
Advancing age | ↓ | ↓↓ | ↑ |
Premenopausal women (vs. male) | → ↑ | ↓ | ↑ |
Other conditions | |||
Renal impairment | → | ↓ | ↑ |
Pregnancy | ↑ | ↑ ↑ | ↓ |
Renovascular HTN | ↑ | ↑ ↑ | ↓ |
Malignant HTN | ↑ | ↑ ↑ | ↓ |
The concentrations and units are different for aldosterone and renin (either direct renin concentration, DRC, or plasma renin activity, PRA), and this must be considered while calculating the ARR. In this regard, it was decided not to establish a rigid cut-off for ARR in consideration of the variability of the RIA assays for aldosterone and renin and the different criteria for entering the values of the two parameters in various centers. In fact, some believe it is appropriate to use the PAC absolute values when the PRA or DRC values are extremely low (cut-off for PAC values greater than 10 ng/dL or 280 pmol/L). A raised ARR value resulting from an extremely low PRA or DRC values in the presence of a PAC value that is low-normal is inconsistent with PA. Thus, ARR should be used when PRA is not lower than 0.2 ng/mL/h or DRC is not lower than 6 mU/L. One of the better combinations in diagnostic accuracy considering ARR cutoff, as the best in sensitivity and specificity, is 30 (PAC in ng/dL and PRA in ng/mL/h) or 3.7 (PAC in ng/dL and DRC in mU/L) [ ].
Blood sample has to be collected in the morning, after the patient has been up (sitting, standing, or walking) for at least 2 h and seated for 5–15 min. It is advisable to correct potassium depletion and ask the patient not to limit sodium intake in the days before blood sampling. Medical wash-out period should be 4 weeks for MRAs (spironolactone, potassium canrenoate, and eplerenone), diuretics and liquorice; and ideally at least 2–3 weeks before the sampling for nonsteroidal antiinflammatory drugs, β-adrenergic blockers, ACE-i, ARBs, dihydropiridine calcium-channel antagonists, and renin inhibitors. Other antihypertensive agents that do not affect the RAAS such as slow-release verapamil, hydralazine, and the α1-selective adrenergic blockers are recommended for HTN control if needed [ , ].
If discontinuation of all the confounding agents is not possible (e.g., severe hypokalemia or uncontrolled hypertension), an ARR should be performed and the results interpreted in light of the potentially interrupting factors. However, careful attention must be paid not only to the ARR, but also to plasma renin and aldosterone concentrations. ARR is a ratio, and therefore, a very low denominator value (renin) leads to an increased ARR, despite normal or even low aldosterone levels. Consequently, a minimum aldosterone concentration is suggested (10–15 ng/dL or 280–420 pmol/L) to suspect PA biochemically [ ].
Notably, the results of an ARR during interfering medications can still be informative: a suppressed renin during medical treatment that should increase renin (as ACE-i, ARBs, diuretics, or MRAs) is highly suggestive of PA. Moreover, ARR cutoffs are different if aldosterone is measured with liquid chromatography coupled with tandem mass spectrometry (LS-MS/MS) [ ].
Confirmatory test after positive ARR
ARR is a screening test, with a high sensitivity (68%–94%), high negative predictive value (100%), but only a moderate specificity. Therefore, false-positive results (i.e., increased ARR in patients who do not have PA) are common, and a second-line confirmatory test is often suggested to confirm or exclude PA [ , ].
It is recommended that during the few days before the confirmatory test, patients have a normal sodium intake and also receive potassium supplementation in order to correct hypokalemia. Saline infusion test is the most widely used, followed by the captopril challenge test, which is simple and effective, and the oral sodium loading test which is less often used. Other tests including the losartan suppression test, the furosemide upright test, and the captopril/valsartan/dexamethasone combination test have also been described [ ].
Indeed, fludrocortisone suppression test (oral 0.1 mg every 6 h over 4 days) is considered by some authors as the most effective method; unsuppressed aldosterone (>6 ng/dL; 167 pmol/L) is highly likely to suggest PA, especially in a case of suppressed renin. However, this test is cumbersome and requires hospitalization. A more feasible and commonly used suppression test (with an acute load of 2 L of isotonic saline over 4 h) is saline infusion test: aldosterone <5 ng/dL (140 pmol/L) after 4 h excludes PA, while >10 ng/dL (280 pmol/L) confirms PA, and levels between 5 and 10 ng/dL (140–280 pmol/L) are considered a “gray zone” and may be seen in patients with idiopathic adrenal hyperplasia [ ]. The saline infusion test has a sensitivity of 88% using the aldosterone cut-off of <5 ng/dL to exclude PA. If a patient is at risk of volume overload, the captopril challenge test is preferred among confirmatory tests: 25–50 mg of captopril is administered orally, and aldosterone, plasma renin activity (or direct renin concentration) are measured after 2 h. After captopril challenge test, a decrease in PAC by <30% and suppressed renin confirms PA (other aldosterone cutoff thresholds between 246 and 390 pmol/L could be considered).
Actually, based on abundant retrospective data, solid evidence is emerging from several authors that in many cases, in which clinical and basic endocrine evaluation are strongly in favor of hyperaldosteronism without any dispute, confirmation tests can be avoided. Of course, this choice, albeit with some risk, could greatly simplify the diagnosis of this pathology. As a matter of fact, confirmatory tests might be redundant if the clinical likelihood of PA is high as in patients with resistant hypertension, low potassium levels, high aldosterone concentration, and suppressed renin.
Subtyping in primary aldosteronism: adrenal vein sampling or imaging?
The subtype diagnosis is pivotal in identifying patients who are suitable for unilateral adrenalectomy. The two subtypes of PA are unilateral or bilateral, although true unilateral PA without any contralateral hypersecretion is less common [ ].
The first step of subtype detection is adrenal computed tomography (CT), which can identify unilateral or bilateral adenomas, micro- or macronodular adrenal hyperplasia, or the rare unilateral carcinoma. CT will not be able to identify the secretory nature of the nodules, neither with CT Hounsfield units (HU) measurement nor with contrast washout characteristics [ ]. Except in selected cases (such as a single adrenal nodule in a young-onset PA), adrenal vein sampling (AVS) is a reasonable choice, especially in the modern concept of multidisciplinary approach to adrenal diseases [ ].
Since the correct localization of adrenal vein is of utmost importance in the AVS interpretation, the interventional radiologist should be skilled and must examine the CT imaging for better planning of AVS. Moreover, incidentally discovered adrenal adenomas are more prevalent in older patients (peak incidence over 65 years); therefore, the accuracy of CT imaging in the diagnosis of subtyping in these patients is lower (i.e., the presence of an adrenal incidentaloma contralateral to the source of aldosterone secretion). On the contrary, due to the low prevalence of adrenal adenomas in the young population, finding a small unilateral adrenal adenoma in a young patient with PA is more likely to suggest ipsilateral disease [ , ]. Moreover, CT imaging is discordant with AVS in 40% of patients with PA [ , ]. Magnetic resonance (MR) is a second choice for imaging adrenal nodules because it’s inferior to CT due to its lower spatial resolution [ ].
AVS is considered the gold standard for subtyping of PA and it is recommended by all international guidelines. It is an essential investigation in order to provide appropriate treatment in patients with PA who have a high probability of unilateral aldosterone-producing adenoma and are willing to have a potential surgical cure [ , ].
AVS is not widely available because it is a challenging procedure, poorly standardized, and has to be performed by an interventional radiologist with great expertise. However, in experienced hands, the technical success rate can be near 90%. It is done by a percutaneous femoral approach and adrenal veins are catheterized simultaneously or sequentially [ ]. Blood samples are obtained from both adrenal veins and from the inferior vena cava (IVC), and assayed for aldosterone and cortisol concentrations. The cortisol concentrations from the adrenal veins and IVC are used to confirm successful catheterization. Adrenal vein to IVC cortisol ratio should be at least 2:1. Dividing the right and left adrenal vein aldosterone concentration values by their respective cortisol concentration provides cortisol-corrected ratios. Then, divide the higher cortisol-corrected aldosterone ratio to the lower one provides the lateralization index. A lateralization index >4 indicates unilateral aldosterone excess, whereas a lateralization index <3 may suggest bilateral aldosterone excess; values between 3 and 4 are indeterminate and may be defined as in “gray zone” [ , ].
According to a recent study, 46% of centers use synthetic ACTH (cosyntropin; ATCH 1–24) infusion during AVS. The logic behind using ACTH is to minimize the stress-induced fluctuations in aldosterone secretion during nonsimultaneous AVS, to maximize cortisol gradient from adrenal vein to IVC and thus confirming accurate sampling, and to maximize the secretion of aldosterone from aldosterone-producing adenomas [ ]. Complications of AVS are uncommon and these include groin hematoma, adrenal hemorrhage, and adrenal vein dissection [ ]. It is noteworthy that AVS can be performed regardless of concomitant medications only if plasma renin activity remains suppressed [ ]. In some patients with PA there is concomitant cortisol hypersecretion, but in most cases, this cortisol excess is mild. Steroid metabolome analysis reveals prevalent glucocorticoid excess in PA [ ]. In these cases, a low-grade cortisol cosecretion has a limited impact on ACTH-stimulated AVS parameters in PA [ ]. Recently, it has been reported that simultaneous androstenedione or metanephrine assays outperformed the cortisol correlation for ascertaining AVS success [ ].
Regarding the role of imaging and AVS in the diagnosis of subtyping PA, one prospective randomized trial comparing AVS with CT-based decision on treatment (SPARTACUS trial) found no significant differences between the postoperative need for antihypertensive therapy and quality of life in 184 patients with PA [ ]. On the contrary, another multicenter international retrospective nonrandomized study reported biochemical remission ratio in 80% (188 of 235) cases after a CT-based treatment decision vs 93% (491 of 526) after an AVS-based treatment decision. In conclusion, patients diagnosed by imaging study only are less likely to achieve complete biochemical remission compared with those diagnosed with AVS [ ].
These data were confirmed by a recent multicenter international study on 1311 patients, which found that imaging alone did not provide an accurate diagnostic value in PA, especially in unilateral PA. In fact in this cohort, cross-sectional imaging did not identify a lateralized cause of disease in around 40% of PA patients and failed to identify the culprit adrenal in 28% of patients with unilateral PA [ ]. Aldosterone-producing cell clusters (APCCs) are one of the main pitfalls of AVS. To describe this briefly, APCCs are group of cells positive for CYP11B2 expression (aldosterone synthetase gene) focally in the subcapsular portion of the human adult adrenal cortex; they produce and secrete excessive aldosterone, but are too small that even modern imaging techniques are unable to localize them [ ]. For further reading on adrenal imaging studies, please refer Chapter 20 .
Laparoscopic surgery and peri-operative management
Surgical treatment of PA aims to remove the source of aldosterone excess and to treat hyperaldosteronism-related comorbidities such as hypertension, hypokalemia, CV, and kidney damages.
Unilateral adrenalectomy is the gold standard treatment for patients with unilateral PA. Laparoscopic adrenalectomy is the preferred surgical approach. However, this should be performed by an expert adrenal surgeon. The duration of hospitalization and the rate of clinical and surgical complications are lower compared with open adrenalectomy [ ]. Adrenalectomy of the entire gland is the treatment of choice. Adrenal sparing approach (cortical sparing partial adrenalectomy) should be avoided as additional aldosterone producing micronodules or APCC clusters, even in case of AVS lateralization, could be present unilaterally. Moreover AVS is usually able to identify which adrenal is responsible for overproduction of aldosterone, but not which part of the gland; hence, nodulectomy of the single lesion alone might not be always curative [ ].
In the recent years, the improvement of surgical techniques, especially with close collaboration between surgeons and endocrinologists, allowed the reduction of perioperative complications. According to the Endocrine Society’s guidelines, blood pressure and potassium levels should be normalized in patients with PA before surgery [ ]. The experience of surgical team and anesthetic support is fundamental, since during surgery a 20 mmHg blood pressure increase and 0.5–1 mEq/L plasma potassium level drop may be observed [ ]. During the first day after surgery, the blood pressure and potassium levels must be strictly monitored. Withdrawal of MRAs and potassium supplementation should be considered in order to avoid postsurgical hypotension and hyperkalemia [ ].
The improvement of blood pressure occurs in a time lapse of 1–6 months after surgery; thus, the concomitant antihypertensive drugs must be downtitrated. An evidence-based approach is not available, so potassium levels and blood pressure should be determined within 3 months after surgery to identify whether to reduce or discontinue the antihypertensive drugs and to optimize potassium supplementation if necessary [ ]. After surgery, the reasonable endpoints are the reduction (47%) or withdrawal (37%) of antihypertensive therapy, and the normalization of serum potassium and ARR (94%) [ , ].
During the first few weeks after surgery, hypoaldosteronism must be anticipated due to chronic suppression of the contralateral adrenal gland, and therefore, a large sodium intake is suggested. Physicians should also pay attention to the development of hyperkalemia, which is reported in 16% of patients; postoperative increased creatinine and microalbuminuria are significant predictors of postsurgical hyperkalemia [ ]. It should be remembered that a minor proportion of patients (close to 5%) who underwent unilateral adrenalectomy could present with persistent hypoaldosteronism requiring further mineralocorticoid replacement therapy [ ], probably due to long-term renin suppression.
In some cases, signs of postsurgical adrenal insufficiency are due to cosecretion of cortisol by aldosterone producing adenomas. In these cases, adrenalectomy could unmask a glucocorticoid insufficiency of the contralateral adrenal gland [ ]; therefore, glucocorticoid substitution treatment should be considered after adrenalectomy.
Medical therapy of primary aldosteronism
The goals of medical treatment of PA are the normalization of blood pressure and potassium levels and the reduction of CV risk. Medical therapy is usually recommended in patients with bilateral adrenal hyperplasia, bilateral aldosterone producing adenomas, and genetic forms of hyperaldosteronism; it is also the treatment of choice in patients with unilateral PA who refuse surgery or in whom surgery is contraindicated [ ].
MRAs are effective in controlling blood pressure and the systemic effects of aldosterone excess. Nowadays, there are two classes of MRAs available in the market, viz. spironolactone and eplerenone.
Spironolactone is a potassium-sparing diuretic which exerts a competitive inhibition on the mineralocorticoid receptor. After oral administration, it’s converted to other active metabolites with long half-lives, such as canrenoate, which are responsible for the majority of pharmacological effects [ ].
The starting dose of spironolactone should be 12.5–25 mg/day; and the dose might be gradually uptitrated until the lowest effective dose is reached, with a maximum ideal dose suggested at 100 mg/day [ ]. Doses between 25 and 50 mg/day had been reported effective in lowering systolic blood pressure by 15 mmHg and diastolic by 8 mmHg, and half of the patients had a blood pressure lower than 140/90 mmHg with spironolactone monotherapy. However, the onset of action of the drug is slow, and it requires many weeks to reach the maximum effects in blood pressure reduction [ ]. Spironolactone often causes some dose-related side effects including gynecomastia and impotence in males and spotting/menstrual irregularities in females. In males, gynecomastia was reported in 10% of cases at a dose of 25 mg/day, while at a dose greater than 150 mg/day its incidence increased to about 52%. The incidence of menstrual irregularities, instead, is not well known. Monitoring potassium levels is suggested though hyperkalemia during spironolactone therapy is rare in patients with PA.
Eplerenone is a newer steroid-selective MRA without significant antiandrogen and progesterone agonist effects. Eplerenone has a 3–6 h half-life and it requires twice daily administration. Due to its receptor selectivity, the side effects are lower compared with spironolactone; however, it has only 50% of the antagonist potency of spironolactone. The main indication of eplerenone is left ventricular dysfunction after myocardial infarction, while its use for PA and hypertension is not approved in every country. Despite its better tolerability, the efficacy in controlling blood pressure could be lower and eplerenone is much more expensive than spironolactone [ ].
Third-generation MRAs (as selective as eplerenone, as potent as spironolactone, and with a nonsteroidal chemical structure) are in development: finerenone has a balanced distribution between the heart and the kidney, equinatriuretic doses of finerenone show more potent antiinflammatory and antifibrotic effects on the kidney in rodent models than spironolactone. Overall, nonsteroidal MRAs appear to demonstrate a better benefit–risk ratio than steroidal MRAs where risk is measured as the propensity for hyperkalaemia. Finerenone reduced chronic kidney disease progression, kidney failure, or kidney death when added to the standard of care, especially in diabetic patients [ ].
MRA treatment can be complimented with other drugs like amiloride, calcium channel blockers, or thiazide diuretics. The use of ACE-i or ARBs could be considered because aldosterone in some adenomas and especially in bilateral hyperplasia is angiotensin responsive; furthermore, after an effective blockade of MR receptor, renin levels are no longer suppressed reexposing RAAS to pharmacological inhibition [ ].
Surgical treatment of PA seems to be more effective than therapy with MRAs not only in controlling blood pressure and reducing the number of antihypertensive drugs, but also in lowering the risk of atrial fibrillation, kidney diseases, and in reversing left ventricular hypertrophy. Moreover, adrenalectomy appears to be superior in reducing long-term mortality than the treatment with mineralocorticoid receptor blockade [ , ].
Fig. 7.2 summarizes the evaluation and management of patients with sporadic PA.
