Pharmacogenomics of Systemic Therapy
James M. Rae
INTRODUCTION TO PHARMACOGENETICS AND PHARMACOGENOMICS
Over the past half century, a large number of studies on the genetic diversity of human drug response have shown that a person’s unique genetic makeup is a major determinant of idiosyncratic adverse drug reactions and therapeutic failure (1). This field, designated pharmacogenetics or pharmacogenomics, is defined as the study of inherited genetic polymorphisms (mostly single nucleotide polymorphisms [SNPs]) in genes that code for proteins responsible for a drug’s pharmacokinetic (absorption, distribution, metabolism, and excretion) and pharmacodynamic (target interaction and signaling alterations) properties. The first groundbreaking pharmacogenetic studies identified gene variants that had profound effects on gene function and these variants had major effects on patient drug response. The advent of high throughput and cost effective DNA sequencing technologies have ushered in the new era of “pharmacogenomic” investigations which use a multi-gene or whole genome approach to identify the germline genetic causes of inter-patient variable drug response.
Pharmacogenetics and pharmacogenomics are shaping the future of personalized medicine, the concept of tailoring the type and dose of drug based on a patient’s unique genetic makeup. This approach promises to have a significant impact in the field of oncology, in which the currently available drugs exhibit substantial variability in their pharmacokinetics and pharmacodynamics and only a limited number of generic therapeutic strategies are employed for all patients. The concept of personalized medicine embraces the goal of “finding the right drug at the right dose for the right patient.”
For the most part, the practice of personalized medicine in breast cancer treatment has been based on using the somatic changes acquired by the cancer during the malignant process to select a therapeutic strategy most likely to be of benefit. Perhaps the two best examples of this approach are the use of estrogen receptor (ER) over-expression and HER-2 over-expression for the selection of anti-estrogen and anti-HER-2 therapies, respectively. A superior understanding of the estrogen-sensitive biology of the tumor (2) has meant that the treatment of breast cancer has led the treatment of many other cancers. The use of tumor-based markers such as the estrogen and progesterone receptors to guide endocrine therapy, first with tamoxifen worldwide and now with the aromatase inhibitor class of drugs, is the standard of care worldwide (3). These advances have been supplemented by the advent of HER2 based therapy in the form of trastuzumab aimed at tumors that express the HER2 receptor (4). The targeting of therapy based on tumor estrogen, progesterone, and human epidermal growth factor receptor 2 expression has recently been supplemented by the use of RNA expressionbased biomarkers that can be used to predict the benefits of chemotherapy with the best example being the clinically used Oncotype Dx™ 21 gene array assay (5).
While approaches based on tumor biology have clearly resulted in improvements in recurrence free survival, the morbidity and mortality associated with breast cancer remain unacceptably high, and the toxicity of current therapies results in unacceptably low levels of compliance. As a result, the need for further improvements in targeting and treatment remains high, and the possibility that inherited variants in genes that control the metabolism, distribution and elimination of drugs, and that code for their targeted receptors and signaling pathways might be important predictors of drug effects and toxicity in breast cancer. The availability of high throughput genomic technologies that are able to screen for hundreds of thousands of individual variants at once has provided insights into the pathogenesis of breast cancer (6), and is now poised to identify inherited genetic associations with responses to specific therapies.
It seems particularly important to explore the inherited germline for clues to therapeutic response, when it is clear
that the treatments now available have been tested and validated in primarily Caucasian populations. Our ability to treat breast cancer in African-Americans, in whom the incidence of breast cancer is 20% lower than that in Caucasians, but the mortality is 20% higher, would appear more limited. Similarly, the notably increasing rates of lethal breast cancer in Asian populations (7) demands serious attention to the question of whether we can ignore differences in response to therapy that might be based not on tumor biology, but on the inheritance of factors that might alter drug efficacy and toxicity. There is widespread precedent for the concept of pharmacogenomics in other fields of medicine where, for example, it is recognized that 7% of the population experience little analgesic benefit from codeine because they lack the ability to metabolize codeine to morphine via the CYP2D6 enzyme (8), or experience an increased risk of intracranial bleeding while being treated with warfarin due to variants in the CYP2C9 or VKCOR genes (9). In addition, a number of pharmacogenomic tests are already available in other areas of cancer treatment.
that the treatments now available have been tested and validated in primarily Caucasian populations. Our ability to treat breast cancer in African-Americans, in whom the incidence of breast cancer is 20% lower than that in Caucasians, but the mortality is 20% higher, would appear more limited. Similarly, the notably increasing rates of lethal breast cancer in Asian populations (7) demands serious attention to the question of whether we can ignore differences in response to therapy that might be based not on tumor biology, but on the inheritance of factors that might alter drug efficacy and toxicity. There is widespread precedent for the concept of pharmacogenomics in other fields of medicine where, for example, it is recognized that 7% of the population experience little analgesic benefit from codeine because they lack the ability to metabolize codeine to morphine via the CYP2D6 enzyme (8), or experience an increased risk of intracranial bleeding while being treated with warfarin due to variants in the CYP2C9 or VKCOR genes (9). In addition, a number of pharmacogenomic tests are already available in other areas of cancer treatment.
PHARMACOGENOMICS IN MEDICAL ONCOLOGY
Thiopurine S-methyltransferase and the Metabolism of Mercaptopurines
Perhaps one of the best studied examples for the application of pharmacogenomic strategies to prevent adverse drug reactions is the polymorphism of the thiopurine S-methyltransferase (TPMT) gene (9). TPMT catalyses the S-methylation of thiopurine drugs such as mercaptopurine and its prodrug azathioprine. These drugs are successfully used to treat acute lymphoblastic leukemia (ALL), and gastroenterologists prescribe thiopurine drugs as second-line (off-label) therapy for Crohn’s disease and ulcerative colitis. Because methylation by TPMT is the predominant pathway for inactivation of thiopurines, patients with TPMT deficiency accumulate active thioguanine nucleotides and this can lead to severe and life-threatening hematological toxicity. TPMT activity in erythrocytes is trimodally distributed among Europeans, European-Americans, and African-Americans and this distribution corresponds well to the genotypes or the respective presence of 2, 1, or 0 functional TPMT alleles. Twenty mutant alleles of TPMT have been associated with low TPMT activity and 3 of these variants (TPMT*2, TPMT*3A and TPMT*3C) account for approximately 95 % of low TPMT activity phenotypes. Approximately 1 in 150-300 individuals is homozygous for inactive TPMT alleles, approximately 10% of patients are heterozygous and have intermediate activity and approximately 90% are normal or high methylators in a Northern European Caucasian population (10).
Because of the strong genotype-phenotype concordance and the severe toxicity associated with high concentrations of thioguanine nucleotides, several cancer centers routinely genotype patients for TPMT mutant alleles and use genotypederived algorithms for dosing. Intermediate metabolizers receive approximately 65% and poor metabolizers 5% to 10% of standard doses of mercaptopurine (11). Dose reductions in patients with variant TPMT alleles lead to similar or superior survival compared to patients with wild-type alleles.
Of major concern is a report of an increased incidence of secondary brain tumors after radiotherapy in children with decreased TPMT activity phenotypes and/or high concentrations of thioguanine nucleotides in blood cells (12). The implications for therapeutic decisions regarding prophylactic radiotherapy in ALL, therefore, must be further investigated.
Uridine Diphosphate glucuronosyltransferase (UGT) 1A1 and the Metabolism of Irinotecan
Results from several recently published trials suggest that patients who are homozygous for a UGT gene variant known as UGT1A1 *28 (the “7/7” genotype) are at greater risk for irinotecan-induced severe diarrhea or neutropenia (13). Irinotecan is a camptothecin analogue and acts on cancer via inhibition of topoisomerase. The disposition of irinotecan is complex and involves numerous metabolic enzymes and transport proteins. SN-38 is the active metabolite of irinotecan and is eliminated via UGT1A1 conversion to SN-38G, an inactive glucuronide cleared via biliary excretion. Reduced activity of UGT1A1 is linked to an approximately four fold increased risk of severe toxicity, including dose-limiting diarrhea and neutropenia. Significant correlations between patients carrying one or two copies of the UGT1A1*28 allele and reduced UGT1A1 expression and reduced SN-38 glucuronidation are now been well documented.
More than 50 mutations in UGT1A1 have been reported, many of which are found in patients with Gilbert’s syndrome, a form of mild nonhemolytic unconjugated hyperbilirubinemia. The most common mutant gene is UGT1A1*28, which contains 7 dinucleotide repeats in the TATA box of the promoter (A(TA)7TAA) instead of the normally 6 repeats, and leads to approximately 70% reduction of transcriptional activity. Many rare mutations also lead to Gilbert’s syndrome, and individuals with this syndrome are predisposed to SN-38 initiated toxicity. Although, as always, a number of additional factors influence the toxicity of SN-38 in the intestine and bone narrow, assessment of the presence of the UGT1A1*28 allele in patients prior to irinotecan treatment may allow lower starting doses or change to alternative therapies. Although there are pharmacogenetic studies that support this position, it is also clear the association of this toxicity with UGT1A1 genotype is irinotecan dose-dependent (14). As a result, many oncologists prefer to simply adjust the irinotecan dose and avoid UGT1A1 genotyping.
PHARMACOGENOMICS STUDIES IN BREAST CANCER
The study of pharmacogenomics in breast and other cancers has been impaired in part by the lack of availability of germline DNA from properly consented patients in the majority of large prospective clinical cancer trials. This is in contrast to other medical disciplines, where the collection of blood, buccal swabs, or saliva to allow extraction of germline DNA has been routine for many years. This obstacle in cancer trials has been overcome, in part, by the key demonstration that effective germline genotyping can be carried out on DNA extracted from paraffin blocks (15). The technical ability to genotype germline DNA from paraffin opens up the possibility of testing pharmacogenomic hypotheses in a large number of clinical studies that have available archived paraffin-embedded specimens with associated well annotated clinical outcomes data. As a result, significant progress has been made in studies designed to examine germline genetic associations with both the efficacy and toxicity of treatments for breast cancer (16).
Genetic Variants Associated with Toxicity of Agents Used to Treat Breast Cancer
Cyclophosphamide Activation by Cytochrome P450 Enzymes
A majority of chemotherapeutic regimens that have been used to treat all stages of breast cancer have used cyclophosphamide as an integral component. Cyclophosphamide
is a prodrug that requires metabolic activation by cytochrome P450 (CYP) enzymes to 4-hydroxycyclophosphamide. Multiple CYPs have been implicated in this activation, including CYP2A6, 2B6, 2C19, 2C9, 3A4, and 3A5 (17). Interestingly, CYP2C19 appears to be the predominant enzyme responsible for cyclophosphamide activation, particularly at low drug concentrations. CYP2C19 is a genetically polymorphic enzyme that is expressed primarily in the liver and that has been shown to be responsible for the metabolism of a wide range of important therapeutic agents (18). The genetic variants in CYP2C19, which have now been studied in a wide range of human populations, results in complete absence of enzyme activity in 3% to 5% of Caucasians and in 15% to 25% of Asian populations. In addition, the CYP2C19*17 allele, which is present in 18% of Caucasians and Africans, and 4% of Asians, codes for a significantly more active enzyme, and interestingly, has been associated with a decreased risk for breast cancer (19). It is reasonable to hypothesize that those individuals who do not carry active CYP2C19 alleles may experience less prodrug activation and, therefore, decreased cyclophosphamide efficacy and treatment related side effects. Furthermore, those individuals who carry the more active CYP2C19*17 allele may experience exaggerated drug response at standard doses.
is a prodrug that requires metabolic activation by cytochrome P450 (CYP) enzymes to 4-hydroxycyclophosphamide. Multiple CYPs have been implicated in this activation, including CYP2A6, 2B6, 2C19, 2C9, 3A4, and 3A5 (17). Interestingly, CYP2C19 appears to be the predominant enzyme responsible for cyclophosphamide activation, particularly at low drug concentrations. CYP2C19 is a genetically polymorphic enzyme that is expressed primarily in the liver and that has been shown to be responsible for the metabolism of a wide range of important therapeutic agents (18). The genetic variants in CYP2C19, which have now been studied in a wide range of human populations, results in complete absence of enzyme activity in 3% to 5% of Caucasians and in 15% to 25% of Asian populations. In addition, the CYP2C19*17 allele, which is present in 18% of Caucasians and Africans, and 4% of Asians, codes for a significantly more active enzyme, and interestingly, has been associated with a decreased risk for breast cancer (19). It is reasonable to hypothesize that those individuals who do not carry active CYP2C19 alleles may experience less prodrug activation and, therefore, decreased cyclophosphamide efficacy and treatment related side effects. Furthermore, those individuals who carry the more active CYP2C19*17 allele may experience exaggerated drug response at standard doses.
Thus, in a study conducted in lupus nephritis patients taking cyclophosphamide monotherapy, it was found that CYP2C19*2 is a predictor of premature ovarian failure and progression to end-stage renal disease (20). These findings have been validated by other investigators; however, not all studies to date have been able to reproduce the association (21). While the precise mechanism of this effect is unclear, these observations suggest that a line of in vitro and clinical investigations are necessary to determine the cause of ovarian toxicity, and thus to prevent it in women at risk, an example of “reverse” translational research. Although these studies suggest that there is clinical relevance to the role of CYP2C19 in the use of cyclophosphamide in other settings, studies to test whether these variants in CYP2C19 or variants in other candidate genes are associated with cyclophosphamide outcomes in breast cancer are not available at present.
Vincristine Inactivation by Cytochrome P450 Enzymes
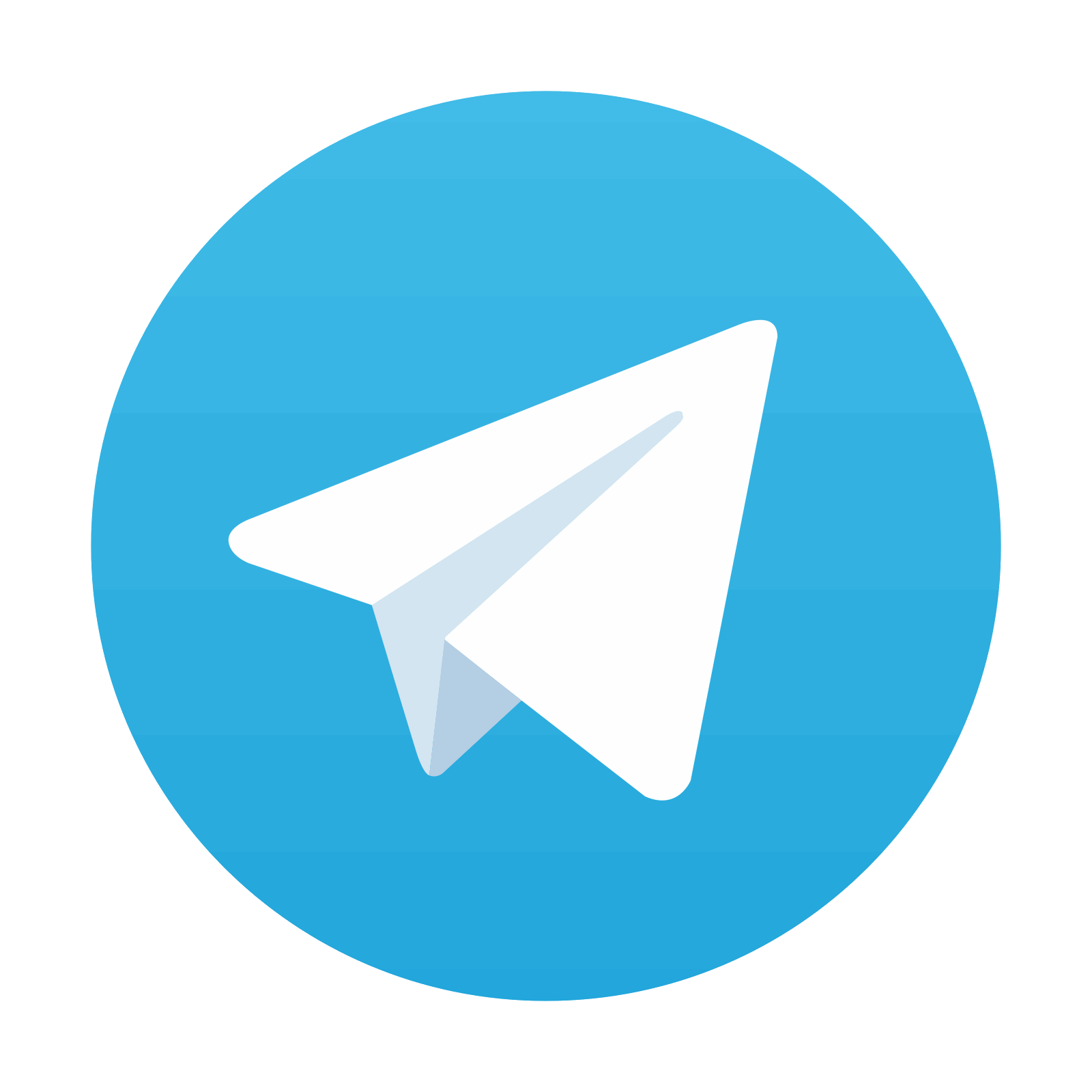
Stay updated, free articles. Join our Telegram channel

Full access? Get Clinical Tree
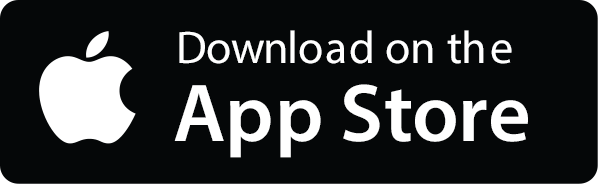
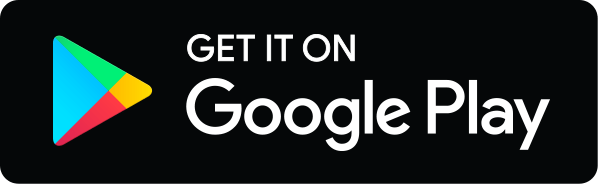
