Fig. 10.1
Embryological development of the prostate
The differentiation and growth of the prostate gland is androgen dependent [4]. The primary androgen involved is testosterone, the majority of which is produced by Leydig cells of the testes [5] and a smaller proportion is produced in the adrenal cortex. With continued development, the epithelium is organised into two distinct populations of cells; namely, basal cells and luminal cells [2]. Each cell type expresses its own characteristic subset of cytokeratins. Simultaneous to this process of epithelial differentiation, the prostatic stroma differentiates into smooth muscle, which is periductal in distribution [6]. At puberty, as serum testosterone levels rise, the prostate increases rapidly in size and undergoes morphologic changes to bring it to the adult phenotype, reaching an approximate weight of 20 g by 25 years of age [7].
10.3 Anatomy of the Prostate Gland
In the adult male, the prostate is a walnut-shaped gland, approximately 4 cm in maximum dimension [8]. It is situated between the bladder and the penis, and the prostatic base surrounds the bladder neck and the pre-prostatic portion of the urethra [9] (Fig. 10.2). The apex of the gland is in contact with the superior aspect of the urethral sphincter and the deep perineal muscle. The rectum lies posterior to the prostate [8]. The posterolateral portion of the gland is bounded by a dense fibrous capsule which contains neurovascular plexuses. Anterior and apical surfaces are covered by the anterior fibromuscular stroma, which is thinner than the fibrous capsule covering the posterior gland [10]. Bilateral seminal vesicles are located on the superoposterior aspect of the prostate. The seminal vesicle ducts join the vasa deferentia to form the ejaculatory ducts. The ejaculatory ducts then pass anteroinferiorly through the prostate to open into the posterior aspect of the prostatic urethra. The smaller prostatic ducts (20–30 in number) open into the prostatic sinuses, which are located on the posterior wall of the prostatic urethra on either side of the seminal colliculus [8].
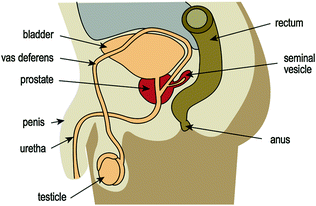
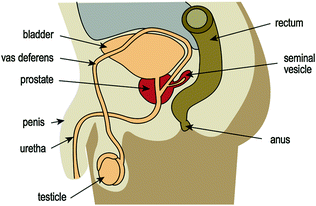
Fig. 10.2
Sagittal view of the prostate and surrounding structures
The prostate comprises several lobes. The anterior lobe is the portion of the gland in front of the urethra and is mainly fibromuscular. The median lobe is situated between the ejaculatory ducts and the urethra. The lateral lobes (right and left lobes) form the main mass of the gland and are separated by the prostatic urethra. The posterior lobe is the area most easily palpable during digital rectal examination [8]. The zones of the prostate include the central, peripheral and transitional zones. The transitional zone surrounds the prostatic urethra and the central zone envelops the ejaculatory ducts, while the peripheral zone provides the bulk of the gland [10] (Fig. 10.3).
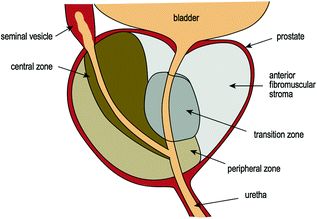
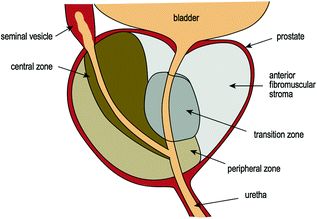
Fig. 10.3
Zones of the prostate
Arterial supply of the prostate is provided by branches of the internal iliac artery; chiefly the inferior vesical artery, but also the internal pudendal and middle rectal arteries [8]. Prostatic veins join to form the prostatic venous plexus, which is located around the basal and lateral aspects of the prostate. The prostatic venous plexus in turn drains into the internal iliac veins. Lymphatic vessels of the prostate terminate predominantly in the internal iliac lymph nodes, and a minor component drains to the sacral nodes. Similar to the other internal male genital organs, the prostate is richly innervated by the autonomic nervous system [11]. Sympathetic fibres arise from T12-L2/3 spinal cord segments, and parasympathetic fibres originate from S2 and S3 segments. Both sympathetic and parasympathetic components join the inferior hypogastric and pelvic plexuses, which innervate the gland. Knowledge of this anatomy is crucial, as nerves can be injured following radical prostatectomy, resulting in erectile dysfunction [12].
10.4 Histology of the Benign Prostate
Microscopically, the prostate parenchyma is best visualised in young males under 50 years of age, when significant distortion by inflammation [13], atrophy [14] and hyperplasia [15] is not yet present. Histologically, the prostate parenchyma consists of two distinct compartments, including the glandular epithelium and the surrounding stromal compartment, which will be discussed separately below.
10.4.1 Benign Glandular Component
The normal glandular epithelium of the prostate (Fig. 10.4) is classically defined as having two cell layers, namely a luminal (or secretory) layer and a basal layer. A third cell type is also present, the neuroendocrine cell, which is usually infrequently seen [16]. The secretory epithelial cells make up the majority of the epithelial volume. These cells are tall and columnar, with clear to pale cytoplasm and basally located nuclei. They are situated on the luminal aspect of the gland, as part of the double layer of epithelium. The nuclei are small and round with fine, evenly dispersed chromatin and nucleoli are usually not prominent. Between the secretory cell layer and the basement membrane lies the basal cell layer. The nuclei of the basal cells are small, and hyperchromatic with scant surrounding cytoplasm. These nuclei are flattened and oriented parallel to the basement membrane. The basal layer is often inconspicuous and incomplete, and it may be difficult to distinguish individual basal cells from underlying stromal fibroblasts in benign prostatic tissue. Recognition of the basal cell layer is, however, important, as these cells are absent in prostatic adenocarcinoma, and should be present in benign conditions that mimic cancer [17]. The final epithelial group, the neuroendocrine cells of the prostate, are irregularly distributed throughout the epithelial compartment [18]. They are inconspicuous, but can occasionally be recognised on haematoxylin and eosin (H&E) staining by deeply eosinophilic fine cytoplasmic granules [16]. The intraglandular contents of normal glands must also be examined and include corpora amylacea [19], (inspissated secretions that appear as pink, concentrically lamellated rings) (Fig. 10.5), calculi and degenerating epithelial cells.
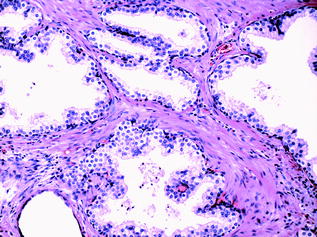
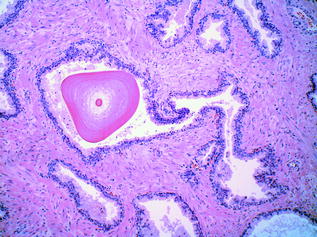
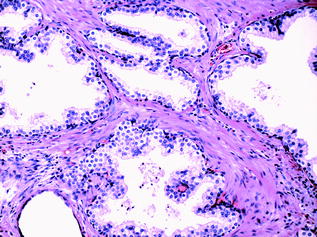
Fig. 10.4
Benign prostatic glands
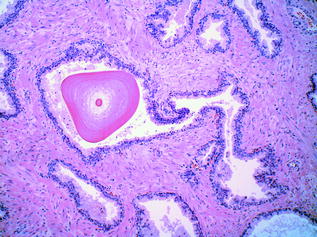
Fig. 10.5
Corpora amylacea
10.4.2 Benign Stromal Component
Histologically, the non-glandular components of the prostate gland include the pseudocapsule, a fibromuscular layer most prominent along the base and posterior portion of the lateral lobes, the fibrous septa, the periprostatic adipose tissue and the stroma. Carcinoma invading adipose tissue is considered to represent extraprostatic extension [20]. Skeletal muscle fibres can also be seen in sections of prostatic tissue, admixed with the stroma at the distal apex of the gland. The prostatic stroma accounts for about half the volume of the prostate gland. The most abundant normal stromal cell type is the smooth muscle cell, derived from the embryonic urogenital sinus. In addition to being the most abundant cell type, the smooth muscle cell appears to be the most important cell type, with respect to homeostasis. Other stromal cells commonly present include fibroblasts, endothelial cells, nerve fibres with associated ganglia, and various immune cells. The extracellular matrix is rich in collagen fibres that intervene between the glandular epithelium. The benign prostatic stroma is affected by ageing, with inflammatory cells becoming more abundant and stromal fibroblasts becoming senescent with age.
10.5 Inflammatory Conditions of the Prostate
Even though in a protected anatomical site, the prostate can be subject to various infections and inflammatory processes, whether of infectious origin or not. Inflammation of the prostate, or prostatitis, is seen commonly in clinical practice, and is usually treated empirically with antibiotics [21]. Histologic examination of specimens removed specifically for prostatitis is therefore uncommon. The inflammatory processes involving the prostate encompass acute and chronic bacterial prostatitis, chronic abacterial prostatitis and granulomatous prostatitis. Acute bacterial prostatitis usually results from similar bacteria to those causing urinary tract infections, namely: Escherichia coli and other gram-negative bacilli, enterococci and staphylococci. Generally, the organisms infect the prostate via reflux of urine from the urethra or urinary bladder. Chronic bacterial prostatitis is caused by similar organisms, and there is often an accompanying history of recurrent urinary tract infections. Mixed acute and chronic prostatitis is depicted in Fig. 10.6. Granulomatous prostatitis is another subtype [22], which may be due to fungal or mycobacterial infection. An attenuated form of the latter may be seen following intravesical therapy with Bacillus Calmette–Guerin (BCG) for bladder cancer. ‘Nonspecific granulomatous prostatitis’ is the most commonly diagnosed granulomatous process within the prostate, and results from an immune-mediated reaction to prostatic secretions from obstructed or ruptured ducts and acini [23]. Lastly, prostatic granulomas are frequent postsurgical sequelae of transrectal biopsy and transurethral resection.
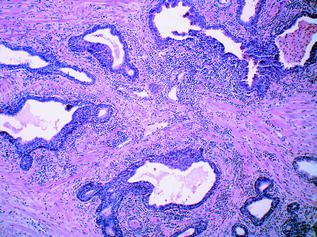
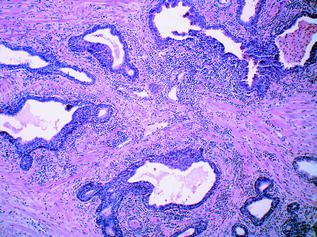
Fig. 10.6
Benign prostatic glands showing mixed acute and chronic prostatitis
10.6 Benign Prostatic Hyperplasia
Benign Prostatic Hyperplasia (BPH) is a common disorder in men over the age of 50 years [21]. It typically affects the central zone of the gland, and is characterised by nodular hyperplasia of either or both stromal and epithelial compartments. The enzyme 5α[alpha]-reductase, located predominantly in stromal cells, converts testosterone to dihydrotestosterone (DHT) [2]. DHT drives the hyperplastic process by increasing stromal proliferation and decreasing epithelial apoptosis. 5α[alpha]-reductase inhibitors (5ARIs) are widely used in the pharmacologic treatment of BPH. Inhibiting DHT production leads to a decrease in prostatic volume, thereby relieving the symptoms and averting the consequences of the disorder. Indeed, the widespread use of 5ARIs (and other medical therapies) in the management of BPH has resulted in a marked shift from surgery to drug therapy. 5ARIs have also been proposed as chemopreventive agents for PCa. Two randomised controlled trials examining the efficacy of 5ARIs for PCa prevention showed a reduction in incidence of up to 25 % when compared with placebo. This decrease was attributed to a reduced incidence of low-grade cancers. However, there was an unexpected concomitant increase of high-grade cancer in the groups treated with 5ARIs; namely, absolute increases of 0.5 and 0.7 % with the use of the inhibitors dutasteride and finasteride, respectively. The Food and Drug Administration (FDA) evaluated the potential risks and benefits of 5ARIs in PCa chemoprevention, and concluded that finasteride and dutasteride do not have favourable risk-benefit profiles. As a result, the use of 5ARIs for PCa chemoprevention has not been endorsed. However, the effect of these drugs on PCa incidence remains controversial [24].
10.7 Premalignant Prostatic Disease
Prostatic intraepithelial neoplasia (PIN) and proliferative inflammatory atrophy (PIA) are the two candidate precursor lesions for PCa [25]. Much of the evidence supporting PIN as precursor to adenocarcinoma is also true for PIA [25]. Compellingly, histologic transitions have been noted between foci of PIA and high-grade PIN (HGPIN), and also between PIA and invasive carcinoma [26]. Both PIN and PIA show particular molecular alterations characteristic of malignancy, which occur in pre-existing epithelium and are confined by the basement membrane. For example, chromosome 8 gain has been observed in PIN, PIA and invasive cancer, lending further support to the precursor status of the former two entities [27, 28]. Although both are precursors of invasive carcinoma, it is unclear whether PIN and PIA represent diseases on the same spectrum or distinct pathways, and they are discussed separately in greater detail below.
10.7.1 Prostatic Intraepithelial Neoplasia
PIN consists of architecturally benign acini lined by cells showing cytologic atypia; namely, nuclear crowding, enlargement, hyperchromasia and prominent nucleolation [29]. There is a surrounding basal cell layer and an intact basement membrane (Fig. 10.7). Most agree that PIN is a precursor of invasive adenocarcinoma, a theory which is supported by the fact that prostates with invasive carcinoma have an increased incidence of PIN, an increase in the number and size of PIN foci, and, on occasion, zones of HGPIN where there appears to be a ‘budding off’ of invasive carcinoma glands. PIN is subdivided into two grades, high and low grades, and the distinction between the two is based on the presence of prominent nucleoli, a diagnostic feature of HGPIN [29]. Most cases of low-grade PIN do not progress, and therefore, many pathologists no longer comment on its presence in biopsy specimens. The presence of multiple foci of HGPIN has a high predictive value for PCa, which develops within 1–2 years in an estimated 30 % of men with multiple cores containing HGPIN [30]. Great variation exists in the literature regarding the incidence of HGPIN on needle biopsy, which is reported to range from 1.5 % to about 16 % [31]. When HGPIN is identified on needle biopsy, a careful search is typically made by the pathologist for invasive cancer in the remainder of the tissue. If no cancer is found, follow-up is unnecessary if only a small amount of HGPIN is present in 1–2 cores. However, if there is a more significant amount of HGPIN, a follow-up protocol is adopted, consisting of serum prostate-specific antigen (PSA) testing, physical examination, and possibly repeat biopsies. However, recommendations for following men with HGPIN have varied widely [32].
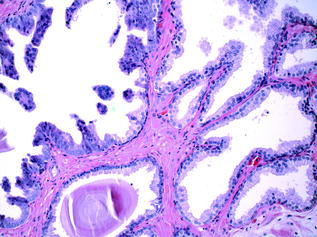
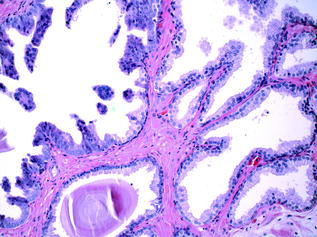
Fig. 10.7
High-grade prostatic intraepithelial neoplasia and adjacent benign glands
10.7.2 Proliferative Inflammatory Atrophy
PIA refers to foci of epithelial atrophy, usually in the periphery of the gland, which show a high proliferative rate, and the presence of mononuclear and polymorphonuclear inflammatory cells within both epithelial and stromal compartments [26]. PIA is associated with chronic prostatitis [26], and it has been hypothesised that repeated tissue injury and cell loss results in proliferative regeneration of damaged epithelium which may subsequently progress to in situ and/or frank invasive carcinoma [33]. The glutathione-S-transferase Pi 1 (GSTP1) gene is located on chromosome 11q13 [21], and plays an important part in cell detoxification [34]. Hypermethylation of the GSTP1 gene (which results in downregulation of GSTP1 protein expression) [21] is seen in PIA, as well as in >90 % of PCa [34]. Importantly, GSTP1 protein detected in free plasma circulating DNA has proved to be a promising biomarker for detection of primary and recurrent PCa [34].
10.8 Histopathology of Prostate Cancer
Grossly, neoplastic prostate tissue can be very difficult to recognise. With widespread PSA screening, a shift towards the detection of smaller tumours and tumour volumes has occurred, making tumours even more subtle. When visible, tumour tissue is nodular and white, and may blend into surrounding benign, tan-coloured parenchyma. Most adenocarcinomas arise in the peripheral lateral or posterior zones, with anterior peripheral localization infrequently occurring. Transitional (20 %) and central zone (5 %) lesions are also less common in comparison to peripheral lesions (70 %). Prostatic adenocarciomas are multifocal in 50–97 % of cases and recent studies now suggest that these lesions are different, in that they are thought to arise from multiple, independent clonal expansions.
10.8.1 Malignant Glandular Component
Histologically, when prostatic adenocarcinoma is referred to without further qualification, it can be assumed to be the common, or acinar, variant [21]. The glands are smaller and more crowded than their benign counterparts under the microscope, and have straight luminal borders rather than papillary infoldings. Having lost the outer basal cell layer, the neoplastic glands are lined by a single layer of cuboidal or low columnar epithelium. The cytoplasm of the malignant cells ranges from amphophilic to the pale/clear cytoplasm seen in benign glands. Nuclei are enlarged and often contain one or more prominent nucleoli. Typically, nuclear pleomorphism and mitotic activity are not prominent. Intraluminal crystalloids or mucinous secretions, when present, are observed mostly in carcinomas, but only rarely identified in benign glands [35]. Although there are a few specific features of malignancy on biopsy (e.g. perineural invasion (Fig. 10.8), which is seen in approximately 20 % of prostate biopsies), the diagnosis is typically made on a combination of architectural and cytologic features on routine H&E. The diagnosis of PCa may be difficult because the clues to malignancy are often subtle, and also because there are several benign mimickers of PCa, such as adenosis, atrophy, and basal cell hyperplasia.
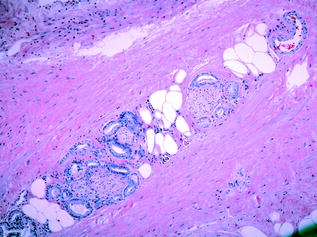
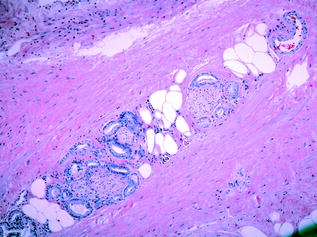
Fig. 10.8
Perineural invasion
10.8.2 Malignant Stromal Component
The majority of prostate tumours are adenocarcinomas arising from epithelial cells lining the prostatic glands. As a consequence, prostatic epithelial cells have been the major focus of histopathological examination and research studies to date. While the development of an altered stromal microenvironment in response to carcinoma is a common feature of many other tumours [36, 37], emerging evidence suggests that changes occur in the surrounding prostatic stroma also, that may serve to enhance the malignant potential of the nearby epithelium [38]. Tumour-associated stroma is referred to as ‘reactive’ in the prostate, as the phenotypic and genotypic alterations seen are similar to those seen in wound repair, including matrix remodelling and altered expression of growth factors and cytokines [39]. The stroma normally consists predominantly of smooth muscle cells, however, during carcinogenesis, these cells are gradually replaced by the activated form of fibroblasts, termed carcinoma-associated fibroblasts (CAFs). There is some debate as to whether CAFs and myofibroblasts represent different cell types, or whether they are the same cell type with different gene expression profiles [40, 41]. It is also unclear as to whether these CAFs are generated by activation of fibroblasts and perivascular cells already present in the prostatic stroma, or whether they are recruited from other locations, i.e. from the bone marrow. CAFs have been shown to affect cancer cells by secreting growth factors [42], extracellular matrix (ECM) components [43] and proteases [44].
Tumour development is also associated with an influx of macrophages [45], lymphocytes and mast cells into the tumour stroma [34]. These inflammatory cells secrete cytokines, which have stimulatory or inhibitory effects on adjacent CAFs, blood vessels and epithelial cells. While inflammatory cells are generally viewed as beneficial, e.g. in wound repair and in preventing the spread of infection, tumour-associated inflammation appears to be associated with an increased rate of growth and the spread of cancer [46, 47]. This large and varied list of stromal cell types may explain the heterogeneity observed in PCa and further research into stromal–epithelial interactions [48] must be performed.
10.8.3 The Use of Immunohistochemistry in Prostate Pathology
The most valuable adjunctive study for the diagnosis of adenocarcinoma of the prostate is immunohistochemistry (IHC), which is used currently in up to 20 % of all prostate needle core biopsies. Any IHC marker, however, carries a risk of false-positive and false-negative results, and therefore must be used in conjunction with the H&E morphology. The immunophenotype of prostatic basal cells is distinctive and can be of diagnostic value, as positive staining with antibodies directed against basal epithelial cells (34β[beta]E12 and p63) rules out the presence of invasive carcinoma [21, 35]. Nuclear basal markers can also be used when the differential diagnosis is between atypia, atypical adenomatous hyperplasia, PIN and atrophy. In some cases, a basal cell marker cocktail can be used to further improve detection of basal cells [49]. In contrast, the normal secretory cells of the prostate stain with pan-cytokeratins, cytokeratins 8 and 18, androgen receptor (AR), PSA and prostate-specific acid phosphatase (PSAP). This immunoprofile distinguishes them from the underlying basal cells. Normal neuroendocrine cells are characterised by their immunoreactivity for synaptophysin, chromogranin or CD56. They variably express AR, an important feature in relation to castration-resistant disease in prostatic carcinoma with neuroendocrine differentiation [50, 51].
Alpha-methylacyl-CoA racemase (AMACR, also known as ‘P504S’ or ‘racemase’) [35] is a commonly used cytoplasmic marker which is selective and very sensitive for carcinoma. While some benign glands (e.g. atrophic glands) and glands showing PIN can also stain positively for AMACR [52], the staining is usually more focal and weaker in comparison to neoplastic glands. Some laboratories combine antibodies in a cocktail also, using a basal marker together with AMACR for definitive diagnosis [53]. A ‘triple stain’ of p63, 34β[beta]E12, and AMACR in prostatic adenocarcinoma can be seen in Fig. 10.9. Stromal tumour markers are not used as routinely in the diagnosis of PCa, however, it is known that decreased expression of smooth muscle cell markers (desmin, smooth muscle actin) and increased fibroblast markers (vimentin) are seen [54]. As no therapeutic targets have currently been identified in PCa, IHC for proteins such as AR, HER2, CD117 (c-Kit), EGFR, etc., are not routinely employed as they are not predictive of response to treatment. Therefore, there is an urgent need to identify molecular signatures to discriminate lethal and indolent PCa and to identify predictive and prognostic biomarkers. While most studies in the past have concentrated on epithelial-based signatures, the stromal microenvironment may hold the answer to this question.
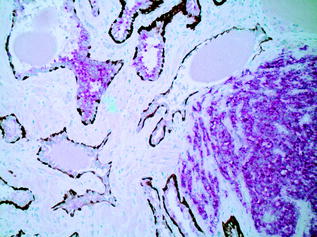
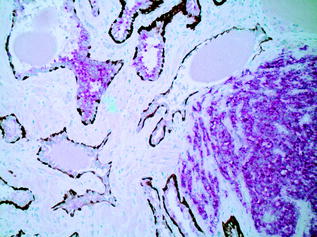
Fig. 10.9
Photomicrograph of a ‘triple stain’ highlighting benign glands, high-grade prostatic intraepithelial neoplasia and prostate adenocarcinoma
10.8.4 Histologic Grading
Histologic grading is the most useful tissue-based prognostic predictor in PCa [55]. The quintessential grading scheme for PCa, the Gleason grading system, is based solely on the architectural pattern of the tumour. Both the predominant (‘primary’) and the second most prevalent (‘secondary’) patterns are identified [56]. Each is assigned a numeric value, and these are added together to yield the ‘Gleason Score’. The grading system originally devised in 1966 by Donald Gleason categorised prostate cancer into five separate grades ranging from 1–5, representing increasingly more aggressive and less differentiated morphologic patterns. In practice, however, grades 1 and 2 are no longer in use, and the primary and secondary patterns of the tumour are generally assigned a value from 3 (well differentiated, with individual, discrete, well-formed glands) to 5 (poorly differentiated/undifferentiated tumour, or tumour with comedonecrosis). A Gleason grade of 4 indicates that the tumour displays an intermediate level of differentiation; poorly formed glands, fused glands, or glands with cribriform architecture [56]. In practice, therefore, the Gleason score on prostatic specimens usually falls within the range of 6–10 [56]. Examples of Gleason patterns (i.e. grades 3+3, 3+4, 4+4 and 5+4) are shown in Figs. 10.10, 10.11, 10.12 and 10.13. In addition, it is advisable to report the percentage of Gleason pattern 4 tumour in a biopsy specimen with an overall Gleason Score of 7; this is in order that clinicians are aware of the approximate extent of the less differentiated (and therefore more aggressive) tumour present in the biopsy material. Increasingly, pathologists also comment on the presence of a ‘tertiary’ component—this situation arises where a third, typically more aggressive pattern is identified. Studies have shown that the presence of a tertiary higher grade component is associated with an increased risk of biochemical recurrence, and it is currently recommended that tertiary grade patterns be recorded in the pathology report in order to accurately reflect the overall grade [57]. The approach to tertiary grading differs between biopsy and prostatectomy specimens. For biopsies, the primary pattern and the pattern of highest grade should be added together to formulate the Gleason score. In the case of a higher grade, tertiary pattern, this would result in the exclusion of the secondary pattern from the overall Gleason score. Conversely, in radical prostatectomy specimens, the Gleason score should be based on primary and secondary patterns, with a comment added on the tertiary pattern [58]. Some special rules apply to Gleason grading of uncommon histologic variants of PCa [55], but these are beyond the scope of this discussion.
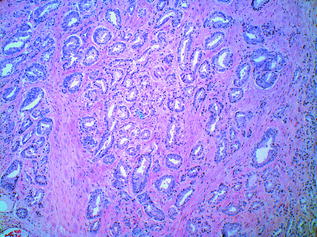
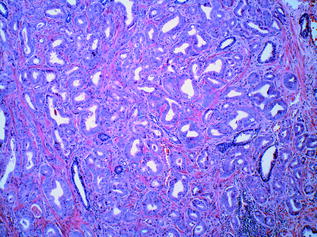
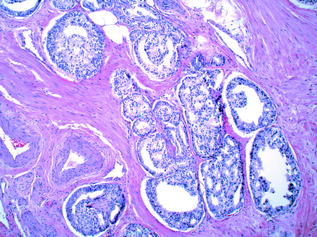
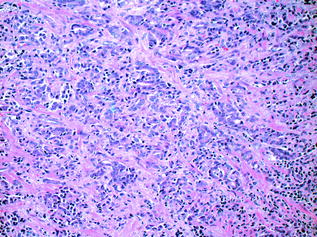
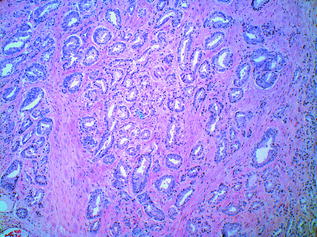
Fig. 10.10
Prostatic adenocarcinoma: Gleason grade 3+3
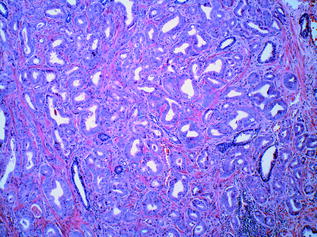
Fig. 10.11
Prostatic adenocarcinoma: gleason grade 3+4
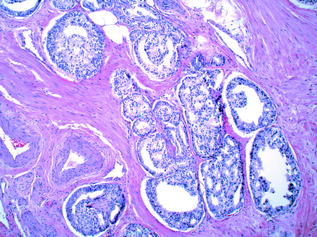
Fig. 10.12
Prostatic adenocarcinoma: gleason grade 4+4
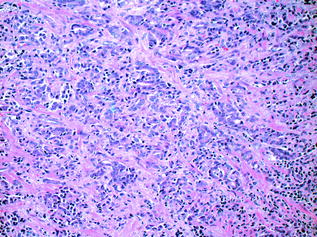
Fig. 10.13
Prostatic adenocarcinoma: gleason grade 5+4
The Gleason grade remains the most reliable prognostic factor in PCa [59], however, there has been a gradual shift in how the Gleason grade is applied in practice, with a general trend towards upgrading. In addition, the Gleason grading system has been adapted over time in order to accommodate changing clinical practices [55]. In particular, a consensus conference which took place in 2005 [60] was organised in order to standardise the perception of the various patterns, as well as the manner in which the information relating to tumour grading was reported. The resultant ‘Modified Gleason System’ reflected this effort at standardisation, and one of its effects was a shift towards upgrading [55]. Studies have shown that needle biopsies using the Modified Gleason System correlated with progression after radical prostatectomy [61] and predicted biochemical recurrence after radical prostatectomy [62]. More recently (November 2014), a second major consensus conference was held with the aim of further updating the Gleason System. Among the parameters examined, an effort was made to standardise the grading of particular morphologic patterns and variants of prostate cancer, including those commonly encountered (e.g. cribriform glands), in addition to relatively uncommon patterns/variants (e.g. glomeruloid glands, mucinous adenocarcinoma, intraductal carcinoma). With regard to the grading of cribriform glands in PCa, according to the updated (2014) guidelines, all cribriform glands are now assigned a Gleason grade of 4, in contrast to the previous iteration of the Gleason System, which designated a Gleason Grade of 3 to small and relatively round/regular cribriform glands. [63] Interestingly, further prognostic information can be gleaned from examining the relative contributions of the individual patterns that ultimately make up the Gleason score. The most striking example of this is the dramatic difference seen between the clinical stages of tumours with a Gleason score of 7, depending on whether there was a primary pattern of 3 and a secondary pattern of 4, and vice versa (3 + 4 = 7, and 4 + 3 = 7, respectively). In one study, 95 % of the 3 + 4 = 7 tumours were clinically staged as pT2, while 79 % of 4 + 3 = 7 cancers were pT3 or pT4 [64]. The prognostic implications of both the overall Gleason score and the relative proportions of the individual component patterns were also discussed at the 2014 consensus meeting, and a novel grading system was proposed. The practice of combining the most common and second most common Gleason patterns to obtain an overall Gleason Score was retained, but the new system stratifies tumours with Gleason Scores of 2–10 into prognostically distinct ‘Grade Groups’ numbered 1–5. Grade Group 1 tumours are composed of only discrete, well-formed glands (therefore with a Gleason Score of 6 or lower), while Grade Groups 2 and 3 comprise tumours of Gleason Score 7 whose component patterns are 3 + 4 = 7 and 4 + 3 = 7, respectively. Grade Group 4 contains tumours of Gleason Score 8, while the most aggressive tumours (Gleason Score of 9 or 10) make up Grade Group 5. These ‘Grade Groups’ are felt to carry greater prognostic value and to more accurately reflect the biology of prostate cancer than the previous Gleason system and various algorithms which had been used for prognostic ‘grouping’ based on Gleason Score. Furthermore, a perceived flaw of the older system was that although 6 was the lowest score assigned to a tumour in practice, a score of 6 out of 10 nevertheless might contribute to patient fear by implying an intermediate rather than an excellent prognosis. It is thought that the new Grade Groups would—for the foreseeable future—be used in conjunction with the Gleason System. The new system and its terminology ‘Grade Groups 1–5’ have been accepted by the World Health Organisation for the 2016 edition of Pathology and Genetics: Tumours of the Urinary System and Male Genital Organs [63].
Lastly, because of heterogeneity and sampling error, some studies report that up to 28 % of cases are upgraded in radical prostatectomies compared to biopsies [65, 66]. This may have a significant impact on clinical decision-making, and can constitute a confusing and problematic factor with regard to the choice of therapeutic approach for a given patient (e.g. active surveillance versus surgery or radiotherapy) [59, 60, 67].
10.8.5 Spread and Staging
Local extension of prostatic carcinoma is usually into periprostatic tissue, seminal vesicles, and bladder base [21]. Cancer staging takes into account whether the cancer is unilateral or bilateral and the presence or absence of invasion beyond the prostate or into surrounding structures. [24] Metastases initially spread via the lymphatics to the obturator nodes, and eventually to the paraaortic nodes. Haematogenous spread is chiefly to the bones, in particular the axial skeleton, proximal femurs and ribs. However, rare cancers can spread widely to the viscera [21]. The reader should refer back to Chap. 3 for an overview of the principles of cancer staging. Organ-specific TNM staging information can be obtained from the current American Joint Committee on Cancer (AJCC) staging manual [68].
10.8.6 Uncommon Histologic Variant: Carcinoma with Neuroendocrine Features
Aside from the common, acinar variant, several distinct morphologic subtypes of prostatic carcinoma exist. These rare subtypes are beyond the scope of this book chapter, but they are described in detail in the current World Health Organisation/International Agency for Research on Cancer’s (WHO/IARC) Classification of Tumours publication relating to the urinary system and male genital organs [69]. Of the uncommon variants of PCa, carcinoma with neuroendocrine (NE) features merits a brief discussion, as it is increasingly recognised as an aggressive variant that is associated with castration-resistant disease [51]. The NE cells of the prostate lack ARs, and NE differentiation increases in the late stages of castration-resistant PCa and in response to androgen deprivation therapy. Transformation to a predominantly AR-negative PCa with increasing NE differentiation may be an important resistance mechanism in castration-resistant disease, and indeed may be more common than was previously recognised. NE tumours of the prostate comprise a heterogeneous group, and the current WHO classification of these cancers includes the following subtypes: Focal NE differentiation in otherwise conventional prostatic adenocarcinoma, carcinoid tumour (well-differentiated NE tumour) and small cell carcinoma (poorly differentiated NE tumour). It has been suggested, however, that this basic classification should be broadened to include three further variants; namely, adenocarcinoma with paneth cell-like NE differentiation, large cell NE carcinoma and mixed NE-acinar carcinoma. Diagnosis of carcinoma with NE features is important, as it is considered an aggressive phenotype of advanced PCa with treatment strategies that differ from those of conventional adenocarcinoma (notably, the use of platinum-based therapies as opposed to AR-targeted treatment). The diagnosis generally depends on histology, as no reliable serum markers are currently available for identification of patients who are transforming to an NE phenotype. However, the detection and molecular characterisation of circulating tumour cells (CTCs) has been suggested for this purpose.
10.9 The Molecular Pathology of Prostate Cancer
PCa is a disease that exhibits great variation in its clinical behaviour. For this reason, prognosis, treatment approach and outcome can vary widely between individuals. The goal of molecular staging in PCa is to identify the genes involved in the pathways that lead to malignancy, and to exploit them as prognostic and/or predictive markers and, as outlined above, to distinguish indolent from aggressive disease. In PCa, as in other malignancies, this field has evolved rapidly in the past few years. Technologies that enable high-throughput analysis have led to a more comprehensive understanding of the progression of the disease, and there has been a dramatic increase in the number of candidate biomarkers for PCa. The identification of these genes and biomarkers uses both serum and tissue-based assays. In the following sections, we examine the resources and techniques presently employed in the molecular pathology of PCa, in addition to the important biomarkers, both current and emerging.
10.10 Resources
Multiple resources are currently utilised to further our understanding of the molecular pathogenesis of PCa, including tissue culture models and mouse models. Examples of tissue culture models include cell lines, organ cultures and organotypic cultures. Cell lines refer to single cell suspensions of cancerous cells, which can be grown for prolonged periods in vitro due to their ability to evade senescence and to continually proliferate [70]. In PCa, the LNCaP cell line, isolated in 1977 from metastatic prostatic carcinoma, has been intensively exploited in research applications [71]. However, cell lines grown as a monolayer in tissue culture dishes lack the same features present in intact tissue. Therefore, three-dimensional representations of tumour microenvironment are now available, using organ cultures, consisting of tissue slices and organotypic cultures consisting of cells grown in an extracellular matrix to recapitulate the biological behaviour of the prostate [72, 73].
Mouse models are another important resource used in PCa research due to the genomic similarities between mice and humans, the relative ease with which genetic modification can be performed, and the fact that mice are comparatively easy to keep and breed [74]. They can be divided into two broad categories, including genetically engineered mouse (GEM) models [75], in which a gene is either knocked out or overexpressed, and mouse xenografts, which involve the injection of human tumour cells into the mouse using various techniques. The main advantage of GEM models is that they reflect tumour progression over time from the initiation of preinvasive lesions, to invasive and metastatic disease. An additional benefit is that this progression is observed within the prostatic microenvironment. The chief disadvantage associated with GEM models is the fact that important biologic differences may exist between murine and human prostates, which may affect model phenotypes [76]. The TRAMP model, one of the original PCa GEM models, was created by inducing the prostate-specific rat probasin promoter (PB) to regulate the SV40 tumour antigens [74]. The TRAMP mice developed epithelial hyperplasia by the time of sexual maturity (approximately 8 weeks of age), followed by PIN at ~18 weeks. By 28 weeks, 100 % had developed invasive tumours with lymphatic metastases, while two-thirds had pulmonary metastases [74]. In addition, this model exhibited hormone-resistant, NE disease after androgen withdrawal. Subsequently, a large number of other GEM models have been developed [77], including Phosphate and Tensin Homolog (PTEN) knockout, Myc overexpression [78], TMPRSS2–ERG fusion, AR manipulation, Rb inactivation [79], APC gene deletion [80], RAS/RAF/MAPK pathway mutations, and TGF-β[beta] signalling loss models, to name a few [76]. The second broad mouse model category are the pure xenograft models, in which fresh human primary PCa tissues are implanted subcutaneously or orthotopically into the prostate of host immunocompromised mice [81]. The latter technique carries the advantage of growth within the prostatic microenvironment, and a number of such models show high rates of metastasis. The LuCaP series is important to note, as it includes a large number of PCa xenograft lines, mostly derived from metastatic disease [82]. Finally, tissue recombination models are another model type, where benign or malignant epithelial cells combined with mesenchymal cells, are implanted subcutaneously or under the renal capsule in immunocompromised host mice [76]. These models represent a powerful method in the study of tumour–stromal interactions in particular.
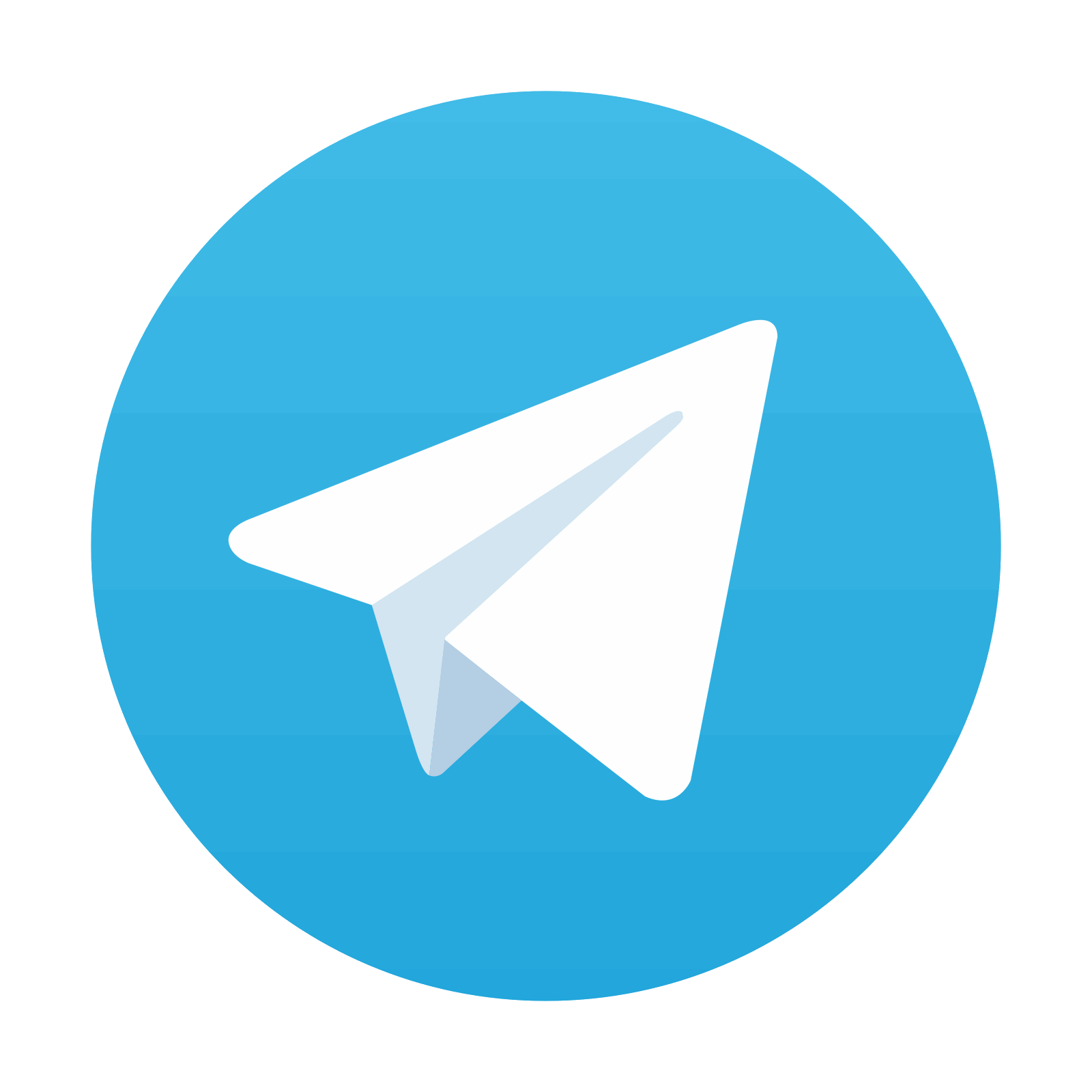
Stay updated, free articles. Join our Telegram channel

Full access? Get Clinical Tree
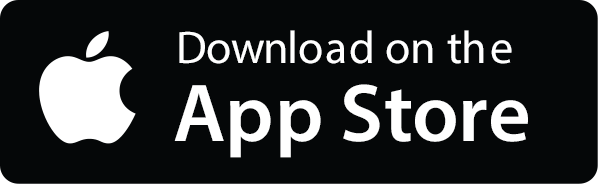
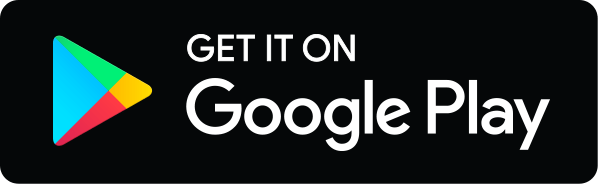