- Cancer is a condition that can affect nearly every organ system in the body. Its prognosis is enormously variable, from nearly 100% survival to almost 100% mortality depending on the site of the primary cancer.
- The majority of patients with cancer amenable to a curative therapy can maintain nutritional status with a normal diet, with or without the need for oral nutritional supplements. Some patients will require nutritional support in the form of enteral or parenteral nutrition at some stage of their disease in order to meet their nutritional needs.
- Many studies have examined the effects of enteral and parenteral nutrition on the outcome of cancer in different settings, with differing results.
- Modern approaches to the optimisation of nutritional status in cancer include the use of appetite stimulants, the modulation of nutrients, and supplementation with immunonutrients or anabolic/anticatabolic agents.
- The prevalence of weight loss observed in people with cancer varies enormously, from 30 to 90%. There is a relationship between weight loss and poor quality of life, and high morbidity and mortality rates have been demonstrated in both medical and surgical malnourished cancer patients.
- Cancer cachexia is a syndrome that occurs in approximately 70% of cancer patients during the terminal course of the disease and may be evident as a clinical presentation in 10–30% of subjects.
22.1 Introduction
Cancer needs to be considered differently from most other challenges in clinical nutrition. As opposed to diseases affecting the bowel, liver, kidney, or lung, which have been discussed in detail in Chapters 11, 12, 14, and 16, cancer can exert its effect on nearly every organ of the body. In addition, cancer and its clinical management need to take into account many other variable factors, such as age, widely variable survival rates, and differing therapeutic options. Because of this variability, cancer serves to illustrate an important principle in standards of clinical nutrition.
Given this level of variability, it is extremely difficult to conduct studies in which the outcomes are not confounded by a wide variety of factors. For this reason, this chapter will set out to demonstrate the evidence supporting the use of nutritional support in this heterogeneous group of patients.
This chapter is laid out in two main sections. The first concentrates on cancer cachexia, outlining the factors thought to be involved in the development of this condition. The second will deal with nutritional management in cancer, including the effects of nutritional support on nutritional status and clinical outcome, and the different modes of nutritional support given to patients with cancer.
22.2 Cancer cachexia
Definition and prevalence
The word ‘cachexia’ is derived from the Greek words ‘kakos’ meaning ‘bad’ and ‘hexis’ meaning ‘state’. Cancer cachexia is a syndrome that occurs during the terminal course of the disease in approximately 70% of cancer patients and may be evident at clinical presentation in 10–30% of subjects. A recent, consensus-based definition of cancer cachexia was published in the Guidelines for Parenteral Nutrition on behalf the European Society for Clinical Nutrition and Metabolism (ESPEN): ‘Cancer Cachexia is a complex syndrome characterized by a chronic, progressive, involuntary weight loss which is poorly or only partially responsive to the common nutritional support and it is often associated with anorexia, early satiation and asthenia. It is usually amenable to two main components: a decreased nutrient intake (which may be simply due a crucial involvement of the gastrointestinal tract by the tumour or by cytokines or similar anorexia-inducing mediators) and metabolic alterations due to the activation of systemic proinflammatory processes.’
Resulting metabolic derangements include insulin resistance, increased lipolysis, and normal or increased lipid oxidation with loss of body fat, increased protein turnover with loss of muscle mass, and an increase in production of acute-phase proteins.
These cytokine-induced metabolic alterations appear to prevent cachectic patients from regaining body cell mass during nutritional support, are associated with a reduced life expectancy, and are not relieved by exogenous nutrients alone.
Quite recently, other definitions of cancer cachexia have been proposed: Fearon et al. (2011) define cancer cachexia as ‘a multifactorial syndrome characterized by an ongoing loss of skeletal muscle mass (with or without loss of fat mass) that cannot be fully reversed by conventional nutritional support and leads to progressive functional impairment. Its pathophysiology is characterized by a negative protein and energy balance driven by a variable combination of reduced food intake and abnormal metabolism. The agreed diagnostic criterion for cachexia was weight loss greater than 5%, or weight loss greater than 2% in individuals already showing depletion according to current bodyweight and height (body-mass index <20 kg/m(2)) or skeletal muscle mass (sarcopaenia).’
Although both these definitions detail the main clinical and metabolic features of cancer cachexia, they are not yet validated for routine use in clinical practice. Only two definitions are validated in the literature. One defines cancer cachexia as being characterised by three main factors: body weight loss ≥ 10%, nutrient intake ≤ 1500 kcal/day, and level of C-reactive protein (CRP) ≥ 10 mg/l. This definition is supported by a strong clinical and pathophysiological rationale, is prognostically validated, and represents the first true attempt to define cachexia according to objective criteria.
Nevertheless it has some limitations. First, it defines cachexia but it does not classify it in different stages of severity. Second, there is an objective difficulty in assessing the energy content of the diet of cancer patients in a nonspecialistic setting and without the help of a dietitian, and, furthermore, this definition requires a blood examination, and consequently the patient must be assessed twice. Finally, this definition was validated in a population of patients with pancreatic cancer only.
More recently, Bozzetti & Mariani (2009) have defined cancer cachexia as ‘a complex syndrome characterized by a severe, chronic, unintentional and progressive weight loss, which is poorly responsive to conventional nutritional support, and may be associated with anorexia, asthenia and early satiation.’
Unlike the previous definitions, this pragmatic statement focuses mainly on clinical self-evident features and emphasises some specific aspects (chronic, unintentional, and progressive weight loss, unresponsiveness to conventional nutritional support). Furthermore, it includes anorexia and early satiety or fatigue, not only for their high prevalence in weight-losing cancer patients but also because their pathogenesis is closely related to the same factors (interleukin-6 (IL-6), tumour necrosis factor alpha (TNFα), etc.) responsible for the derangement of metabolism and for the weight loss. Hence their presence might reflect the role of some common specific mechanisms underlying both the clinical appearance and the metabolic picture of cancer cachexia.
Depending on the degree of the weight loss and the presence/absence of one/all of these symptoms, it is possible to stratify patients in four different classes (Figure 22.1). These classes (from 1 to 4) represent stages of progressive severity of the cachexia. Moving from ‘asymptomatic precachexia’ (class 1) to ‘symptomatic cachexia’ (class 4), there are statistically significant trends (P < 0.0001) in the percentage of gastrointestinal versus non-gastrointestinal tumours, severity of cancer stage, percentage of weight loss, number of symptoms per patient, Eastern Cooperative Oncology Group (ECOG) performance status, and nutritional risk score.
Figure 22.1 Classification of cancer cachexia proposed by SCRINIO (SCReenIng Nutritional status In Oncology). Modified from Bozzetti & Mariani (2009), copyright © 2009 by SAGE Publications. Reprinted by permission of SAGE Publications.
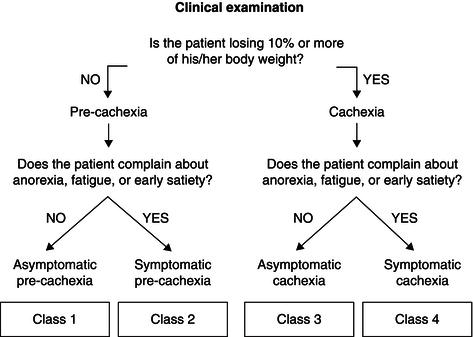
Other characteristics of cancer cachexia include anaemia, depletion, and alterations in body compartments, disturbances in water and electrolyte metabolism, and the progressive impairment of vital functions. Specific investigations into the composition of the body compartments of cancer patients have shown an increase in the ratio of total body water to total body potassium compared with normal subjects. A recent study in cachectic patients, which determined total body water and total body potassium isotopically, concluded that total body water can be accurately predicted, whereas measured values of total body potassium were significantly lower than predicted. Total body water, therefore, significantly overestimated the metabolically active tissue in weight-losing cancer patients.
Unlike patients with simple anorexia nervosa, in whom weight loss accounts for a more or less proportional decrease in the size of all organs, patients with malignant cachexia have preferential sparing of the liver, kidney, and spleen, as well as significant involvement of other parenchyma. Lieffers et al. (2009) recently reported that increases in mass and in the proportion of high-metabolic-rate tissues (liver, spleen, and tumour) represent a cumulative incremental resting energy expenditure (REE) of approximately 17 700 kcal during the last 3 months of life and may contribute substantially to cachexia-associated weight loss.
Patients with malignant tumours have the highest prevalence of malnutrition of any segment of the hospitalised population. The prevalence and severity of cachexia are not always directly related to calorie intake, histological variety, or type of tumour spread; nor are they related to tumour size, since weight loss may be present even when the tumour represents less than 0.01% of the total body weight. In some cancer patients, weight loss may be the most frequent presenting symptom, affecting up to 66% of patients during the course of their disease. The overall prevalence of weight loss may be as high as 86% in the last 1–2 weeks of life.
A weight loss greater than 10% of the pre-illness body weight may occur in up to 45% of hospitalised adult cancer patients. In a recent prospective investigation aiming to define the nutritional status of cancer outpatients, Mariani et al. (2012) reported the degree and prevalence of malnutrition in 1500 patients (Table 22.1).
Table 22.1 Prevalence and severity of weight loss in different primary cancers. GI, gastrointestinal. Reprinted from Mariani et al. (2012), © Springer.
Primary % weight loss (median) | % of patients with ≥10% weight loss | |
Upper GI | 6.6 | 35 |
Oesophagus | 15.1 | 66 |
Stomach | 11.7 | 57 |
Pancreas | 14 | 64 |
Small bowel | 4.4 | 21 |
Colon rectum | 4.8 | 28 |
Lung | 6.4 | 35 |
Upper respiratory | 5.4 | 25 |
Others | 8.1 | 45 |
Pathophysiology
Our understanding of the aetiopathogenesis of cancer cachexia is limited and based more on knowledge of abnormalities in nutritional behaviour and metabolic patterns than on the identification of specific mediators. Three theories have been suggested:
Metabolic-competition theory
The metabolic-competition theory suggests that neoplastic cells compete with host tissues for amino acids, functioning thereby as a sort of ‘nitrogen trap’. This may be true in experimental tumours, where neoplastic tissue accounts for a very high percentage of the carcass weight, but it is unlikely to be an important mechanism in human tumours, where the common experience is the opposite. There are cases of both cachectic patients with tumours of only a few grams and patients with a rather good general status with huge abdominal masses. Therefore, such a theory can only explain a very small fraction of cases of nutritional deterioration in cancer patients.
Under-nutrition
The second theory supports the role of under-nutrition as the main cause underlying the development of cancer cachexia. The reason for the reduced intake of nutrients in patients with lesions of the upper digestive tract is clear. A study carried out by the Istituto Nazionale Tumori, Milan, showed that the prevalence of subjective anorexia ranged from 33 to 40% of patients (depending on the type and site of the tumour), and that most of the nutritionally related variables were significantly worse in anorectic patients than in nonanorectic patients. Studies in the 1970s and 1980s found that anorexia is seen as a presenting symptom of malignancy in around 14–50% of newly diagnosed cancer patients, since abnormalities of food intake and feeding patterns occur. More recent studies report a prevalence of anorexia of about 60–64%, and up to 85% in patients with advanced disease.
However, regardless of the tumour’s location, anorexia is the most common cause of reduced intake and usually consists of a loss of appetite and/or a feeling of early satiety. Anorexia often precedes the development of malnutrition and may be a presenting symptom of malignancy in 25% of patients. However, Bosaeus (2008) has reported that weight loss cannot be accounted for by the diminished dietary intake, since energy intake in absolute amounts is not different and intake per kilogram body weight is higher in weight-losing patients than in weight-stable patients, although long-term dietary intake may be difficult to assess precisely.
The presence of anorexia adversely affects quality of life: in systematic analyses of symptoms of advanced cancer, anorexia, together with pain and fatigue, is consistently among the 10 most prevalent and one of the top five most distressing symptoms reported by patients.
The mechanisms involved in the onset of anorexia are poorly understood. Older studies supported the role of intermediate metabolites (lactate, ketones, low-molecular-weight peptides, oligonucleotides) coupled with a state of relative hypoinsulinaemia.
Parabiotic experiments, in which a nonmetastasising tumour is implanted in an animal whose circulation is connected surgically to that of another non-tumour-bearing animal, demonstrate that anorexia, metabolic changes, weight loss, and cachexia occur in the non-tumour-bearing animals as well, despite the lack of evidence of metastatic tumour at necroscopy. Since the two surgically connected animals only share 1.5% of their total circulation, this suggests the presence of a tumour factor such as a cytokine.
Increased serotoninergic activity within the central nervous system (CNS) has been proposed as a possible cause of anorexia. Such activity is secondary to the enhanced availability of tryptophan to the brain, since a close relationship between elevated plasma-free tryptophan and anorexia was observed in cancer patients with a reduced food intake. The uptake into the brain of tryptophan is competitive with that of branched-chain amino acids (BCAAs), and administration of BCAAs by increasing the plasma levels of these competitors at the level of the blood–brain barrier may lead to a decrease in the occurrence of anorexia.
Cytokines may have a pivotal role in long-term inhibition of feeding, through mimicking the hypothalamic effect of excessive negative-feedback signalling from leptin. This can be achieved by persistent stimulation of anorexigenic neuropeptides such as adrenocorticotropic hormone (ACTH)-releasing factors, as well as by inhibition of the neuropeptide Y orexigenic network, which consists of opioid peptides and galanin, in addition to the newly identified melanin-concentrating hormone orexin and agouti-related peptide.
Leptin, the hormone produced by fat that suppresses appetite and increases energy expenditure to maintain weight stability, has been found in appropriately low levels in weight-losing cancer patients during initial studies.
Reduced intake has also been related to the presence of dysgeusia – a distortion or absence of the sense of taste. The decreased ability to perceive sweet flavours has been linked to anorexia, whereas a decrease in the threshold for bitter flavours has been linked to an aversion to meats rich in bitter substrates (amino acids, purines, polypeptides). Altered smell perception is also related to aversion to food.
In underfed patients there are secondary changes in the gastrointestinal tract, such as a decrease in secretions and atrophy of the mucosa and musculature, which may be responsible for the feeling of fullness and delayed emptying, the defective digestion, and the poor absorption of nutrients. Delayed gastric emptying and gastroparesis are also common in patients with advanced cancer. A comprehensive review on cancer anorexia was published by Laviano et al. (2003).
Alterations of metabolic pathways
The third theory underlying the pathogenesis of cancer cachexia relates to metabolic abnormalities. These span overall energy balance, the individual macro- and micronutrients, and the acute-phase response.
Energy balance
It is generally accepted that resting energy expenditure (REE) in patients with cancer is more variable than in normal subjects and is frequently increased by 50–300 kcal/day. The metabolic basis of this increase is poorly understood. One possibility concerns the mass and metabolic rate of malignant tissue. The mass of human tumours rarely exceeds 4% of body weight, so if it is assumed that the associated metabolic rate (per kilogram of tissue) of this tissue is similar to that of the rest of the body, a large increase in REE will not be expected. However, information about the energy expenditure of human malignant tissue in vivo is lacking. It is possible that a metabolically active tissue might contribute disproportionally to REE. By analogy, four organs (brain, kidney, heart, and liver), which account for about 5% of body weight, are responsible for about half of the REE in a healthy adult. Another possibility concerns the energy costs of metabolic processes induced by the tumour, such as increased gluconeogenesis, increased glucose–lactate recycling, and protein synthesis, all of which require adenosine triphosphate (ATP). This increased requirement will be associated with increased heat production.
A further explanation for the alteration in energy expenditure is based on the observation that cancer patients tend to oxidise a higher quantity of non-esterified fatty acids (NEFAs) than control subjects, even in the presence of other energy substrates. This indirectly implies a channelling of carbohydrate intermediates towards lipogenesis before oxidation, which represents a costly metabolism in terms of the consumption of ATP. It has also been reported that protein turnover can account for up to 50% of REE, with the liver usually accounting for 20–25% of overall oxygen consumption. Investigations in humans have shown that while the synthesis of muscle protein is diminished in cancer patients, the hepatic synthesis of secretory proteins, acute-phase reactants, fibrinogen, glycoproteins, and immunoglobulins can be increased.
The activity of the sympathetic nervous system may also contribute to basal hypermetabolism. It is difficult to explain the large variability in REE by a single metabolic process and it is likely that a combination of metabolic processes is involved, and that the combination varies with the type of cancer and stage of disease.
Total energy expenditure (TEE), which includes REE plus the physical-activity energy expenditure, may not be increased in cancer patients, as shown by tracer studies in free-living patients with cancer and other techniques in other malignancies. In fact, TEE may appear decreased in advanced cancer patients when compared to predicted values for healthy individuals, mainly because of a reduction in the physical activity. Recent data with the use of a wearable device, the SenseWear Armband (SensorMedics Italia Srl), would indicate that the TEEs of weight-stable leukaemic patients and of weight-losing bedridden patients with gastrointestinal tumours are about 24 and 28 kcal/kg/day, respectively.
Table 22.2 Studies on resting energy expenditure (REE) in cancer patients. PEE, predicted energy expenditure. Bozzetti F. Nutrition support in patients with Cancer. In: Artificial Nutrition Support in Clinical Practice (J Payne-James, G Grimble, D Silk, eds) 2001, Greenwich Medical Media, now published by Cambridge University Press, reproduced with permission.
In conclusion, the decrease in physical activity, which is very common in advanced malignant diseases, offsets or more than offsets any increase in REE. This implies that the loss of energy stores which may eventually lead to cancer cachexia is more likely to be due to a decrease in energy intake than an increase in energy expenditure.
Data on the prevalence and severity of hypermetabolism are shown in Tables 22.2 and 22.3.
Carbohydrate metabolism
Carbohydrate metabolism can be considered as whole-body and skeletal-muscle glucose metabolism, hepatic glucose metabolism, and tumour glucose metabolism. In this context, the main alterations in carbohydrate metabolism include decreased oral glucose tolerance, which occurs in 37–60% of tumour-bearing patients. By using the intravenous glucose tolerance test, it has been demonstrated that head and neck cancer patients have reduced first-phase insulin response, similar to that seen in type 2 diabetes, and a reduced glucose disposal. This result seems to be directly correlated, at the multiple-regression analysis, not only with acute insulin response but with triiodothyronine concentration. The reduced glucose disposal indicates that there is insulin resistance, with reduction in the normal ability of the insulin-sensitive tissues (e.g. muscle, gut) to take up glucose.
Investigations using the euglycaemic hyperinsulinaemic clamp technique have generally shown that there is a significant reduction in glucose utilisation when the insulin concentration is in the physiological range, and that this effect is not overcome with administration of supraphysiological insulin concentrations. Fasting serum insulin is normal or low. On the whole, these studies imply that glucose may not be utilised in the whole body of cancer patients as well as lipids or amino acids. Since glucose oxidation appears to only be mildly reduced, the major defect in glucose utilisation probably resides in non-oxidative glucose disposal or in the synthesis of glycogen.
Table 22.3 Resting energy expenditure (REE) and type of tumour. Bozzetti F. Nutrition support in patients with Cancer. In: Artificial Nutrition Support in Clinical Practice (J Payne-James, G Grimble, D Silk, eds) 2001, Greenwich Medical Media, now published by Cambridge University Press, reproduced with permission.
Hepatic glucose metabolism can also be altered during cancer cachexia. Approximately 75% of studies report an increase in the rate of hepatic glucose production in cancer patients. Gluconeogenesis from several precursors (lactate, alanine, glycerol) has been reported to be increased, as have total glucose production, turnover, and recycling. Cancer patients also have an increased glucose flux, which could consume up to 40% of the carbohydrates ingested and may contribute to the weight loss. The outpouring of lactic acid by some tumours leads to an increase in the conversion of lactate to glucose by the liver. This process – the Cori cycle – is energy-consuming, because the conversion of 2 moles of lactate to glucose requires 6 moles of ATP, whereas only 2 moles of ATP are recovered in the reconversion of glucose to lactate, and it has been estimated to account for 300 kcal of energy loss per day.
Although the Cori cycle normally accounts for 20% of glucose turnover, this rises to 50% in cachectic cancer patients and accounts for disposal of 60% of the lactate produced. Both glucose production rates were found to be higher in malnourished cancer patients than in non-cancer patients with comparable weight loss. The increase in glucose recycling, equivalent to 40% of the daily glucose intake of the cancer patient, has been estimated to lead to a potential loss of 0.9 kg of body fat per month.
The tumour itself can contribute to changes in carbohydrate metabolism. There is some evidence that a disproportionate requirement for glucose by the tumour accounts for the increase in glucose turnover and consumption. Perfusion experiments determined that glucose uptake by tumour tissue in patients with soft-tissue sarcomas was approximately 160 mg/minute (230 g glucose/day) when uptake remained constant for 24 hours. Glucose uptake is proportional to tumour size and corresponds to approximately 1.4 g/day/gram of tumour, a value which would account for the increase in glucose metabolism (170 g/24 hours) observed in sarcoma-bearing patients.
Most solid tumours, either because of isoenzyme alterations or because of their poor vascularisation and hence hypoxic nature, rely almost exclusively on the anaerobic metabolism of glucose as their main energy source, with most being converted to lactate. Glucose uptake and lactate release by human colon carcinoma have been found to exceed the peripheral tissue exchange rate by 30-fold and 43-fold, respectively. This extra requirement for glucose by the tumour is accompanied by a marked decrease in glucose utilisation by host tissue, particularly the brain, which resembles the situation found in starvation.
In conclusion, tumours have been demonstrated to affect the rate of glucose utilisation in a number of tissues. Given that there are only 1200 kcal stored in the body as liver and muscle glycogen, blood glucose levels would be expected to fall. This does not occur, because of the parallel increase in the hepatic glucose production. This production of endogenous glucose cannot be suppressed by the administration of exogenous glucose, which would be effective in healthy volunteers. In fact, studies in hepatic cell cultures from tumour-bearing subjects have demonstrated a dose-related increase in glucose synthesis from physiological concentrations of lactate and alanine (and a further increase in the presence of an excess of precursor), which probably takes place through a mechanism of induction of the gluconeogenic enzymes.
Hypoglycaemia has been reported in patients with carcinoma of the stomach, cecum, or bile ducts, with pseudomyxoma, and with paraganglioma, sometimes occurring before the presence of a tumour is suspected. Hypoglycaemia was originally thought to be a result of high glucose consumption by the tumour, but recently it has been suggested that it arises through the ability of some tumours, other than insulinoma, to produce insulin or insulin-like substances. Since insulin levels have been shown to be low in cases of tumour-associated hypoglycaemia, the production by a tumour of an insulin growth factor has been suggested as the cause of enhanced peripheral glucose uptake. The most likely candidate for the pathogenesis of extrapancreatic tumour hypoglycaemia is the production of a high-molecular-mass (15–25 kDa) insulin-like growth factor type 2 (IGF-2) by the tumour. Thus, patients with cancer have an increased glucose production and turnover, which may be enhanced by the production of IGF-2. Such changes contribute to an increased energy expenditure by the host.
The main abnormalities of carbohydrate metabolism are summarised in Box 22.1.
- decreased glucose tolerance;
- insulin resistance in insulin-sensitive tissues (muscle, gut, etc.);
- defect in non-oxidative glucose disposal or in glycogen synthesis;
- increase in gluconeogenesis from lactate, alanine, and glycerol;
- increase in total glucose production, turnover, and recycling.
- depletion of fat stores and lipid content in muscle;
- increased lipolysis and fat oxidation;
- increased fatty acid and glycerol turnover.
- unchanged or decreased muscle protein synthesis;
- unchanged or increased protein breakdown;
- increased hepatic synthesis of acute-phase proteins;
- specific alterations of plasma amino acid profile.
Lipid metabolism
Under normal conditions of feeding and fasting, two adipose-tissue enzymes, hormone-sensitive lipase and lipoprotein lipase, control the uptake and breakdown of fat from adipose tissue. During early starvation, decreased insulin levels and increased glucagon and adrenaline result in cyclic adenosine monophosphate (cAMP) activation of a protein kinase, which phosphorylates and activates the hormone-sensitive lipase. This enzyme hydrolyses the lipid droplet of the adipocyte into NEFAs to be released into the circulation. Conversely, the activated lipoprotein lipase hydrolyses the core of the circulating triacylglycerol-rich lipoproteins into NEFAs and monoacylglycerol. These NEFAs are the major source of substrate for adipocyte triacylglycerol (TAG) synthesis, since adipocytes synthesise very small amounts of fatty acids de novo. This mechanism seems to be disrupted in anorexic weight-losing cancer patients. In patients with cancer cachexia there is an overall depletion of fat stores and lipid content in muscle samples. The rates of depletion are equal, as suggested by the relative increase in fractions (phospholipid and free cholesterol) of polyunsaturated fatty acid (PUFA). The increased serum concentration of NEFAs, when present, could explain the decreased post-receptor insulin resistance. Fasting plasma glycerol concentrations have been shown to be higher in weight-losing cancer patients than in weight-stable cancer patients, thus providing evidence for an increase in lipolysis. The main abnormalities of lipid metabolism are summarised in Box 22.1.
Some patients, especially if undernourished, exhibit a clear state of hyperlipidaemia and have a decrease in plasma lipoprotein lipase. The elevated plasma lipid level may be immunosuppressive, and thereby affect ultimate survival. Several clinical studies have observed an increased mobilisation of fatty acids before weight loss occurs, suggesting the production of lipid-mobilising factors (LMFs) either by the tumour or by host tissue. This could contribute to tumour growth. Patients with ovarian or endometrial tumours were found to have lower concentrations of linoleic acid in subcutaneous adipose tissue than cancer-free subjects, suggesting mobilisation to supply lipid to the tumour. Linoleic acid has been found to act as a stimulator of tumour growth both in vitro and in vivo. Whole-body lipid metabolism was found to be increased in cancer patients with weight loss. The increase of lipolysis may be due to an increase in the hormone-sensitive lipase.
Cancer patients with weight loss have an increased glycerol and fatty acid turnover compared with normal subjects and cancer patients without weight loss. There is also an increased oxidation of fat, which is proportional to the stage of disease and to the severity of the malnutrition, and an increased rate of removal of infused lipids from the blood, suggesting that host tissues may increase their utilisation of fatty acids as an energy source even in the presence of high plasma glucose concentration. It is interesting that the administration of glucose fails to inhibit fat mobilisation and oxidation, as usually occurs in normal patients.
Protein metabolism
Abnormalities of protein metabolism in cancer patients (shown in Box 22.1) include nitrogen depletion (lean body mass and visceral protein) in the host, changes in hepatic and muscular protein turnover, an increase of gluconeogenesis from amino acids, and abnormal patterns of plasma amino acids. Investigations into protein kinetics have shown conflicting results. Whole-body protein turnover has been reported as being not significantly different from control, or even increased. Discrepancy in absolute values among different studies may reflect heterogeneity in the investigative methods, whereas differences between cancer patients and controls may be related to the type of cancer population and choice of controls. The exceptionally high protein turnover rate found in some patients with hepatocellular carcinoma has been demonstrated to be a consequence of elevated endogenous protein breakdown and oxidation of amino acids.
Some authors have reported specific alterations of plasma amino acid profile. A recent extensive collective review by Lai et al. (2005) has summarised the alterations in the levels of the single amino acid and, interestingly, has reported that in patients with cancer of the head and neck or of the gastrointestinal tract (i.e. the patient population which most often requires nutritional support), the plasma level of all of the essential amino acids (except threonine) is always decreased.
Compared with normal colon tissue, human colon tumours were observed to have a specific requirement for serine and for BCAAs.
There is some discrepancy in the results of the literature in the area of protein metabolism in muscle.
The more consistent finding is that there is a decrease in protein synthesis, but normal and increased rates have also been reported. The negative net balance is explained by a normal breakdown concurrent to a decreased synthesis, or by an increase in breakdown.
Recent research supports the concept that muscle wasting is associated with an increased gene expression and the activity of the calcium/calpain and ubiquitin/proteasome proteolytic pathways in addition to the classic proteolytic mechanisms due to the lysosome cathepsins B, H, D, and L. Calcium/calpain-regulated release of microfilaments from the sarcomere is an early and perhaps rate-limiting component of the catabolic response in muscle. Released microfilaments are ubiquinated in the N-end rule pathway, regulated by the ubiquitin-conjugating enzyme E214k and the ubiquitin ligase E3α, and degraded by the 26S proteasome. Several proinflammatory cytokines, including TNF, IL-1 and IL-6, and glucocorticoids, stimulate production of ubiquitin mRNA. In muscle loss, pale fibres are affected more than red fibres, and predominantly myofibrillar proteins are involved, as shown by measurements of 3-methylhistidine.
As regards hepatic protein metabolism, studies with radiolabelled leucine have shown that while in normal subjects 53% of hepatic protein synthesis derives from muscle, it accounts for only 8% in malnourished cancer patients. Synthesis of structural liver proteins is normal, and that of the acute phase is increased.
There is a correlation between hypoalbuminaemia and tumour bulk. Studies with labelled precursors have reported a reduction in albumin synthesis, which is partially corrected by increased protein intake, and an increase in the catabolised fraction. However, recent investigations have shown that synthesis of negative acute-phase reactants such as albumin is maintained despite reduced circulating concentrations, whereas synthesis of positive acute-phase reactants (e.g. fibrinogen) is significantly increased. There is also an increase in transcapillary escape, with a decrease in the intravascular and extravascular ratio.
In rare cases, hypoalbuminaemia is also caused by its sequestration in pathological compartments or by loss to the outside (nephrotic syndromes, protein-losing enteropathy, aminoaciduria in acute leukaemias). Such findings could explain the frequent occurrence of hypoalbuminaemia even when degradation is normal and synthesis is increased, as reported in recent investigations. Chronic administration of TNF in tumour-bearing animals results in a reduction of whole-body protein synthesis and net loss of skeletal protein, but in an increase in liver protein synthesis.
The tumour itself can interfere with protein metabolism. The metabolic-competition theory suggests that the tumour acts as a ‘one-way nitrogen trap’, and that this may have an influence on body economy when the mass accounts for 20% of the body weight of the host. However, it has been found that experimental tumours containing less than 6% of total body nitrogen have a protein metabolism equal to 35% of all protein synthesis. In humans it has been demonstrated that when the tumour is localised and resectable (i.e. in colorectal cancer), there is no substantial impact on the overall protein kinetics, while measurement of the difference in arteriovenous concentrations of amino acids in tumour-bearing limbs versus normal extremities has shown that less than half of the output of amino acids is released in the circulation by the tumour-bearing limb.
Some studies have demonstrated that the amino acid appearance rate is related to tumour bulk. Measurements in tumour tissue of protein metabolism have established that the tissue has a very high fractional protein synthesis rate of 50–90% per day. This is very similar to the liver and contrasts with a rate of 1–3% for skeletal muscle. In a recent study it was found that those cancer patients who had an elevated plasma amino acid appearance rate survived and those with a normal rate died. Although this issue is controversial, it would appear that an adequate acute-phase response to tumour may reflect a more effective fight against cancer.
Acute-phase response
The development of cancer cachexia may elicit an acute-phase response to malignancy, which is a basic defensive and phylogenetically primitive response of the body against injury. This includes reductions in serum iron and zinc levels, alterations in amino acid distribution and metabolism, and increases in acute-phase globulin synthesis and gluconeogenesis, negative nitrogen balance, and sometimes fever. This process is due to cytokines secreted by macrophages at the tissue site of the tumour, which inhibit albumin synthesis and stimulate the synthesis of acute-phase proteins.
These include:
- CRP, which promotes phagocytosis, modulates the cellular immune response, and inhibits the migration of white blood cells into the tissues.
- Alpha-1-antichymotrypsin, which minimises tissue damage due to phagocytosis and reduces intravascular coagulation.
- Alpha-1-macroglobulin, which forms complexes with proteases and removes them from circulation, maintains antibody production, and promotes granulopoiesis and synthesis of other acute-phase proteins.
An acute-phase protein response is seen in a significant proportion of patients bearing tumours that are frequently associated with weight loss (e.g. pancreas, lung, oesophagus) and is more frequent in the advanced stages of disease. The presence of such a response in patients with pancreatic or renal cancer is associated with a shortened survival, perhaps due to the exaggerated muscle breakdown required to supply amino acids for the acute-phase-response protein synthesis.
Vitamin and mineral deficiencies
Deficiencies in vitamins may be present in some undernourished cancer patients. The most significant include reductions in plasma levels of folate, vitamin A, and vitamin C. Between 19 and 52% of hospitalised patients have been noted to have reduced serum folate levels and 18–35% of patients who die of cancer and subsequent infections have severe liver deficiency of vitamin A at autopsy. Recently it has been found that many cancer patients have low levels of 25(OH)D (<50 nmol/l); such low levels are associated with a high incidence of cancer and are a marker of poor prognosis.
Mineral deficiencies can occur in some cancer patients as part of the cytokine-mediated inflammatory response (in addition to being a consequence of common causes such as poor oral intake, increased dietary requirements, and excessive urinary and stool losses). Zinc concentrations in the blood can drop as an early response to cytokines, which may be elevated in cancer patients. In cancer patients who are hospitalised, a low serum zinc (<0.7 mg/l) is not uncommon and usually normalises after a 3-week administration of 50 mg zinc/day. Serum copper is normal or elevated in cancer patients, with a pattern similar to that of serum zinc. Serum iron levels usually fall as result of cytokine-mediated response.
Hormonal milieu
Many patients develop insulin-resistance syndrome as a result of cancer and exhibit a small but significant elevation in serum insulin concentration. As a consequence, as in the case of diabetes, they have reduced glucose utilisation, loss of first-phase insulin response, and sometimes increased fasting hepatic glucose production. As already mentioned, the weight-losing cancer patients frequently have an increase in fatty acid oxidation and plasma fatty acid appearance rate. The rate of TAG hydrolysis is much higher than that of fat oxidation, so that albumin-bound fatty acids are partially utilised for energy, but many are utilised for re-esterification or substrate cycling back to TAGs.
Multiple studies have failed to demonstrate elevation in the counter-regulation of hormones, with the exception of a mild elevation in urinary free cortisol secretion. Catecholamines and glucagon are usually normal, but growth hormone (GH) was found to be increased at 24-hour analysis and by random sampling in malnourished cancer patients. Levels of GH are further increased by the infusion of arginine and insulin. Hypogonadism has been reported in male cancer patients and free triiodothyronine and triiodothyronine concentrations can both be low.
As a normal response, to save energy in the injured subject, the body’s ability to convert the stored form of a thyroid hormone (thyroxin or T4) into the active form of thyroid hormone (triiodothyronine or T3) becomes impaired and T4 is converted to an inactive form known as reverse-T3 hormone (rT3). This process can occur in aggressive cancers, when the patient’s response is similar to that of an injury response. There is no evidence of increased thyroid hormone levels during refeeding.
Mediators
Cytokines
The cytokines which were identified as mediators of cancer cachexia include TNF, IL-1, -2, -4, and -6, and interferon α, β and γ. These cytokines are produced by immunocytes as an endogenous immune response to the tumour and regulate many of the nutritional and metabolic disturbances that occur in the host with cancer, leading to:
- decreased appetite;
- stimulation of the basal metabolic rate (BMR);
- stimulation of glucose uptake;
- stimulation of the mobilisation of fat and protein stores;
- reduction in adipocyte lipoprotein lipase activity;
- enhanced muscle amino acid release;
- stimulation of hepatic amino acid transport activity.
Elevated concentrations of tissue and circulating cytokines have been found in cancer patients and enhanced hepatic cytokine gene expression has been found in tumour-bearing animals.
Recent studies have reported that in patients with different solid tumours, TNF polimorphism was associated with anorexia (lung cancer), IL-1β gene polimorphism was associated with cachexia (gastric cancer), IL-1β genetic polymorphisms were shown to have a possible modulatory role in the cancer anorexia/weight-loss syndrome in patients with metastatic gastric and oesophageal cancer, and pro-inflammatory cytokine haplotypes (IL-6 CC, IL-10 GG, TNFα) were associated with adverse prognosis in pancreatic carcinoma.
Table 22.4 Cytokine-mediated effects on protein, carbohydrate, and lipid metabolism. TNF, tumour necrosis factor; IL, interleukin; LPL, lipoprotein lipase; IFN, interferon.
A comprehensive review on this topic can be found in Seruga et al. (2008).
The clinical effects of the administration of some cytokines to experimental animals are reported in Table 22.4 and are covered in the next section.
Both animal and human studies show that administration of TNFα causes an initial drop in body weight followed by a decreasing response with repeated administration. Acute administration of TNFα causes effects similar to those seen in cancer cachexia, including an increase in metabolic rate (by 30%), plasma TAGs, glycerol turnover (by more than 80%), and NEFA turnover (by more than 60%), as well as in temperature, heart rate, and epinephrine and ACTH levels. Similar effects were seen on protein metabolism, where whole-body protein turnover, total amino acid efflux, and acute-phase protein response were increased. It is noteworthy that in human studies where TNF was given to cancer patients intermittently, it did not cause weight loss, perhaps because it was not possible to consistently elevate TNF levels. In the studies, these responses were blunted by ibuprofen despite the absence of changes in the plasma levels of TNFα. However, in chronic administration the alterations were resolved, despite continuous administration of TNFα.
Moreover, in no phase I clinical investigation of TNFα was cachexia a major side effect; instead, fluid retention through damage to the vascular endothelium and increased capillary permeability were reported. Only hypertriacylglycerolaemia persisted despite the development of tachyphylaxis, but this seems to be unrelated to cachexia, since, for instance, hypertriacylglycerolaemic AIDS patients maintain their weight for prolonged periods of time.
Experimental studies suggest that the increase in serum TAGs due to TNFα administration is mainly due to hepatic synthesis and secretion of very low-density lipoproteins (VLDLs) rather than adipose tissue. Depletion of skeletal muscle would be due to the induction of oxidative stress and nitric oxide synthase, and consequent decreased myosin creatine phosphokinase expression and binding activity. It is possible that naturally occurring TNFα inhibitors in the circulation may hide TNFα production by the host’s monocytes. In fact, these inhibitors were found to be circulating freely in healthy volunteers and to be increased in individuals with ovarian cancer. The inability to detect TNFα in cachectic patients has led to the suggestion that it acts as a paracrine/autocrine mediator rather than as the circulating messenger in cachexia.
Production of TNF by isolated peripheral blood mononuclear cells has been shown to be elevated in weight-losing pancreatic cancer patients with an acute-phase protein response, suggesting that local rather than systemic production may be the more relevant.
Some investigators did not find elevated TNFα levels in weight-losing or weight-stable cancer patients and this may be due to the presence of circulating inhibitors or to their action as paracrine/autocrine inhibitors. Nevertheless, there was a twofold increase in the relative levels of messenger ribonucleic acid (mRNA) for hormone-sensitive lipase in the adipose tissue of cancer patients compared with controls. These findings suggest that TNFα may not contribute to the development of cachexia in cancer, but indicate a stimulation of lipolysis in adipose tissue. Anti-TNFα antibodies have had minimal effects in tumour-bearing animals, even if in a human squamous cell carcinoma of the maxilla, grown as a xenograft in nude mice, anti-TNFα antibodies partially reversed, but did not completely normalise, body weight.
IL-1 has been shown to have many effects similar to those of TNFα, including suppression of lipoprotein lipase and enhancement of intracellular lipolysis. Elevated IL-1 plasma levels are seldom found in cancer patients, but antibodies against an IL-1 receptor mitigate cachectic symptoms in tumour-bearing mice to a similar degree to anti-TNFα antibodies.
Unlike TNFα and IL-1, increased levels of IL-6 are measured in the serum of cancer patients. IL-6 is the main cytokine involved in the induction of acute-phase proteins and fibrinogen synthesis, and elevated levels have been reported in 39% of patients with lung or colon cancer and an ongoing acute-phase protein response. However, since all of the patients had lost weight, it is difficult to determine whether IL-6 was elevated in cachectic patients alone. Antibodies to IL-6 administered to patients with AIDS-related lymphoma produced weight gain in those losing weight and stabilised the levels of the acute-phase-reactant CRP. The increase in circulating IL-6 is closely related to tumour burden and is thought to originate from tumour cells as well as from various tissues of the cancer-bearing host (e.g. liver, kidney, small intestine, etc.) and to be induced by the IL-1 production of tumour-infiltrating macrophages. Again, local production of IL-6 by peripheral blood mononuclear cells is important, and its production seems to be elevated in patients with pancreatic cancer and an acute-phase protein response.
Interferon gamma (IFNγ) is known to have effects on fat metabolism similar to those of TNFα, namely the inhibition of lipoprotein lipase and of protein synthesis, and polyclonal antibodies anti-IFNγ partly mitigate cancer-induced anorexia and weight loss. Increased serum IFNγ levels have been found in 53% of patients with multiple myeloma. However, no association was observed between the level of IFNγ and the clinical parameters of the disease. These findings suggest that IFNγ alone may not be responsible for the induction of wasting. In the long term, a vicious cycle is activated that results in anorexia and widespread abnormalities in carbohydrate, protein, and lipid metabolism (Figure 22.2).
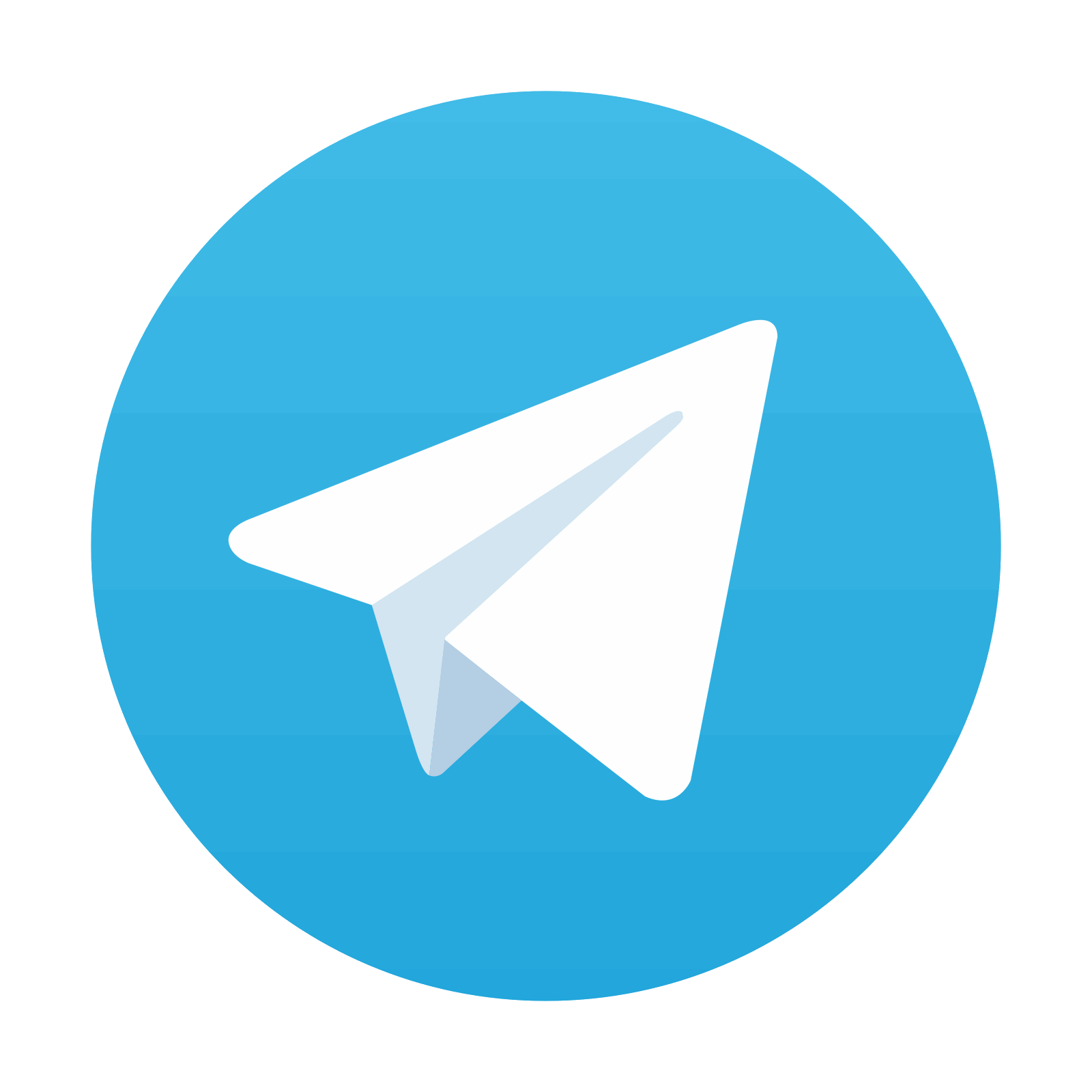
Stay updated, free articles. Join our Telegram channel

Full access? Get Clinical Tree
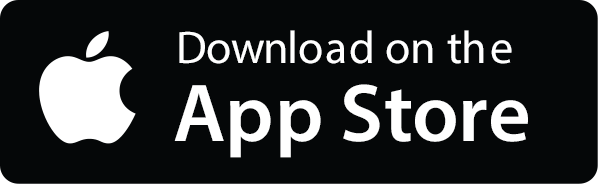
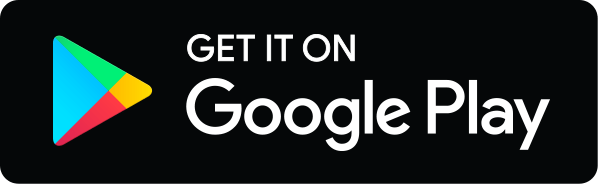