- Bone health is the result of complex interactions between genetic determinants of peak bone mass, lifestyle factors (including nutrition and exercise), the hormonal milieu, and vitamin D status.
- Adequate sun exposure is the most important determinant of vitamin D status.
- Vitamin D deficiency due to poor sun exposure in at-risk individuals, leading to rickets in infants and children and osteomalacia in adults, is entirely preventable.
- Age-specific issues need to be considered with regard to nutritional requirements in order to ensure normal bone growth during infancy, childhood, and adolescence, for the maintenance of bone mass throughout adulthood and minimisation of bone loss during senescence.
- Osteopaenia and osteoporosis are frequent complications of chronic systemic disease and may be exacerbated by dietary mineral ion and protein under-nutrition and vitamin D deficiency.
- Bone metabolism is integrally linked with other organ systems in the body and involves osteocyte function, which is modulated by weight-bearing and shear forces on bone. These cells are also integrally involved with phosphate metabolism. Recently, osteocalcin, produced by osteoblasts, has been shown to be a vital link between metabolic processes and bone health, including modulation of insulin sensitivity.
19.1 Introduction
The skeleton is a complex structure, comprising mineral ions, predominantly calcium and phosphorus, which precipitate to form the crystalline structure of hydroxyapatite, which itself precipitates on the helical collagen framework of bone-matrix proteins to form the rigid, strong structure characteristic of normal bone. This chapter will review the basic concepts of calcium homeostasis and bone metabolism, with particular emphasis on relevant dietary aspects in health and disease, from conception to senescence.
Linear bone growth during infancy, childhood, and adolescence ceases once bone growth plates fuse; however, ongoing bone turnover, with resorption of old bone and formation of new bone, continues throughout life. Normal mineral-ion homeostasis involves multiple organ systems, namely the parathyroid glands, skin, gastrointestinal system, liver, and kidney. Perturbations of any of these organ systems may affect mineral-ion homeostasis and bone metabolism. Consequently, nutritional deficiencies and various chronic diseases may have profound effects on skeletal health. The complex interactions between mineral-ion nutrition, protein/calorie nutrition, and vitamin D status will be discussed in this chapter, together with aspects of diagnostic imaging techniques and laboratory testing relevant to assessment of the skeleton.
19.2 Overview of bone metabolism and mineral-ion homeostasis
Bone metabolism
Throughout life, bone, like skin and the lining of the gut, is continually turned over, in processes referred to as ‘modelling’ in growing children and ‘remodelling’ in adults. Bone-resorbing cells (osteoclasts) resorb bone; their action is coupled with that of bone-forming cells (osteoblasts), which lay down new bone (Figure 19.1); osteoblast/osteoclast coupling is mediated via a complex paracrine hormonal interplay between these two cell types, and involves hormones such as receptor activator of nuclear factor kappa-B ligand (RANKL), fibronectin, and osteoprogerin. Osteocytes, derived from the osteoblastic lineage, are the most numerous type of bone cell. They intercommunicate via cellular processes, to act as ‘strain gauges’, sensitive to weight-bearing and shearing forces applied to bone, and have a critical role in stimulating new bone formation. Weight-bearing exercise is an important determinant of bone strength; conversely, immobilisation due to prolonged bed rest, or in more recent years, ‘weightlessness’ in prolonged space travel, has been shown to result in bone loss. Osteocytes also produce fibroblast growth factor 23 (FGF23) and phosphate-regulating neutral endopeptidase (encoded on the X chromosome) (PHEX), which act on the kidney and are major regulators of phosphate homeostasis.
- genetic influences;
- hormonal factors, including sex hormones (oestrogen and testosterone from puberty through to middle age), bone-morphogenic proteins (BMPs), and insulin-like growth factor 1 (IGF-1);
- dietary calcium intake and vitamin D status;
- lifestyle factors such as weight-bearing exercise, protein/calorie nutrition, alcohol intake, and tobacco smoking.
Figure 19.1 Cellular interactions involved in endochondrial bone formation. Canalis E et al. Mechanisms of Anabolic Therapies for Osteoporosis, New England Journal of Medicine 2007; 357: 905–916. Copyright © Massachusetts Medical Society.
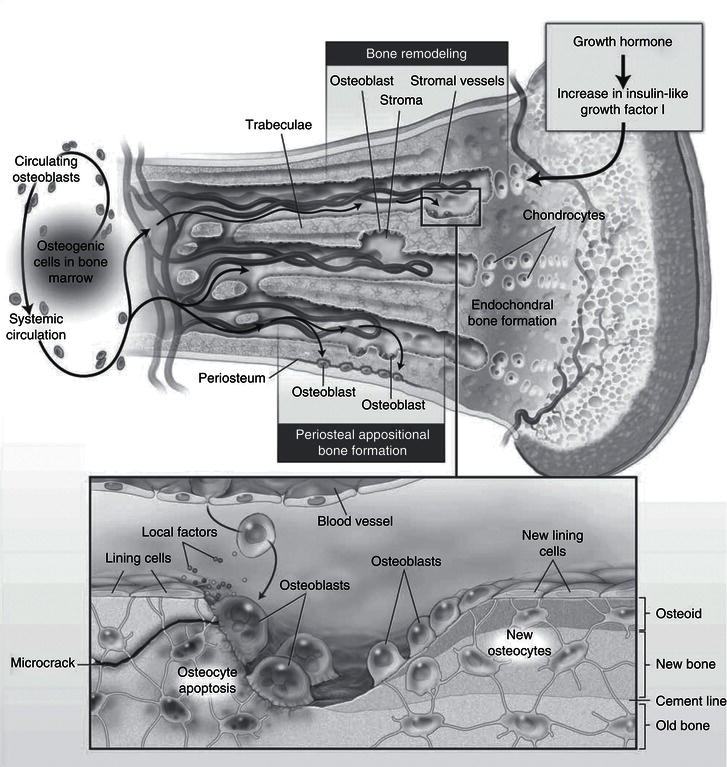
During normal growth in childhood, bone formation exceeds net bone resorption, and bone mass steadily increases through to adolescence, during which approximately 40% of bone mass accrual occurs. By the beginning of the third decade of life, peak bone mass has been attained and bone mass reaches a steady state, and in otherwise healthy young adults bone resorption occurs in balance with bone formation until menopause in women and late middle age in men, when bone resorption starts to exceed net bone formation, leading to osteoporosis over the ensuing decades.
The major determinants of peak bone mass are listed in Box 19.1.
The nutritional and hormonal (autocrine, paracrine, and endocrine) influences on bone formation and resorption are complex. Hormonal influences include parathyroid hormone (PTH), 1,25-dihydroxy vitamin D3 (1,25(OH)2D3), IGF-1 and 2, BMPs, transforming growth factor beta (TGFβ), FGF, osteocalcin, sex hormones, and various cytokines and lymphokines. Other factors, including (i) nutritional factors such as obesity, malabsorption syndromes, and anorexia nervosa, (ii) chronic systemic diseases such as cystic fibrosis and chronic renal failure, and (iii) pregnancy and lactation, also affect bone metabolism. In recent years the hormone osteocalcin, produced by osteoblasts, has been identified as an important link between regulators of nutrition, such as insulin, and bone metabolism.
Mineral-ion homeostasis
Mineral-ion homeostasis is important for both normal metabolism and structural skeletal rigidity. The major ions concerned are calcium and phosphorus. Bone osteoid comprises bone-matrix proteins, predominantly collagen, which are laid down in a complex helical structure and provide tensile strength to bone. The mineral ions calcium and phosphorus precipitate within this helical structure as hydroxyapatite crystals (Ca10(PO4)6(OH)2) to form a strong, rigid structure characteristic of mature bone.
In growing infants and children, this process predominantly occurs at the metaphyseal growth plates, within the long bones. Once these growth plates have fused and linear growth has ceased, bone formation and resorption occur in a coupled fashion throughout the healthy adult skeleton. During senescence, new bone formation diminishes with ongoing bone resorption, leading to osteoporosis. Unmineralised osteoid, which occurs in rickets in children and osteomalacia in adults, results in soft, pliable bones. Defective mineralisation will result as a consequence of either calcium or phosphate depletion, or a combination of both.
Calcium
The adult skeleton contains 1–1.5 kg calcium (20–25 mg/kg fat-free tissue) and represents 99% of total body calcium. Less than 1% of calcium circulates in a soluble form, and it is this fraction that plays a vital role in neuromuscular and cardiovascular function, in coagulation, and as an intracellular second messenger for cell-surface hormone action, in addition to its roles in gene transcription and cellular growth and metabolism. In the presence of dietary calcium insufficiency, circulating blood calcium is maintained at normal concentrations, primarily through the actions of PTH, which maintains circulating calcium at the expense of bone mineralisation (Figures 19.2 and 19.3).
Figure 19.3 Vitamin D/calcium metabolism: PTH, parathyroid hormone, produced by the parathyroid glands as shown; [Ca], serum calcium; [P], serum phosphate; UCa, urinary calcium; UP, urinary phosphate.
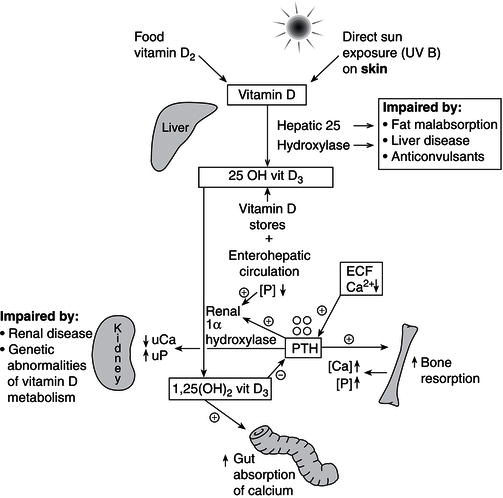
Dairy foods are the richest, most bioavailable natural food sources of calcium. The recommended daily intakes (RDIs) for calcium are listed in Table 19.1 and dietary sources in Table 19.2. Infant RDI is almost always met, as breast milk or formula feeds are the sole dietary intake of infants under 3 months of age, and should continue to form the bulk of their diet until 9–12 months of age. Calcium intake beyond this age may be inadequate due to cultural food practices, food faddism, or exclusion diets due to cow’s-milk allergy or lactase deficiency. Fortification of fruit juices, bread, and cereal with calcium may provide alternative dietary sources of calcium for those on such exclusion diets. In the event that the RDI for calcium cannot be met, there are a number of commercially available preparations of calcium supplements.
Table 19.1 Estimated average requirement (EAR) and recommended dietary intake (RDI) for calcium. Adapted from Australian National Health and Medical Research Council (2006).
EAR | RDI | |
Children and adolescents | ||
All | ||
1–3 years | 360 mg/day | 500 mg/day |
4–8 years | 520 mg/day | 700 mg/day |
Boys | ||
9–11 years | 800 mg/day | 1000 mg/day |
12–13 years | 1050 mg/day | 1300 mg/day |
14–18 years | 1050 mg/day | 1300 mg/day |
Girls | ||
9–11 years | 800 mg/day | 1000 mg/day |
12–13 years | 1050 mg/day | 1300 mg/day |
14–18 years | 1050 mg/day | 1300 mg/day |
Adults | ||
Men | ||
19–30 years | 840 mg/day | 1000 mg/day |
31–50 years | 840 mg/day | 1000 mg/day |
51–70 years | 840 mg/day | 1000 mg/day |
>70 years | 1100 mg/day | 1300 mg/day |
Women | ||
19–30 years | 840 mg/day | 1000 mg/day |
31–50 years | 840 mg/day | 1000 mg/day |
51–70 years | 1100 mg/day | 1300 mg/day |
>70 years | 1100 mg/day | 1300 mg/day |
Pregnancy | ||
14–18 years | 1050 mg/day | 1300 mg/day |
19–30 years | 840 mg/day | 1000 mg/day |
31–50 years | 840 mg/day | 1000 mg/day |
Lactation | ||
14–18 years | 1050 mg/day | 1300 mg/day |
19–30 years | 840 mg/day | 1000 mg/day |
31–50 years | 840 mg/day | 1000 mg/day |
The effectiveness of calcium absorption from commercial preparations is to some degree dependent on the type of calcium salt, timing in relation to meals, and number of doses. Calcium citrate-malate (CCM) preparations have been found to be absorbed better than calcium carbonate salts, but CCM is not universally available and is unobtainable in some countries. However, overall the benefits of one calcium salt over another are not substantial.
The efficiency of calcium absorption is affected by pH and other components of food, such as oxalates and phytates, and calcium supplements are best taken in multiple doses rather than as a single daily dose, to maximise absorption efficiency. However, as the number of daily doses of supplements increases, subject compliance is also likely to decrease.
Reduced gastric acid secretion secondary to atrophic gastritis, which is commonly seen in elderly populations, may also contribute to dietary calcium deficiency in the elderly, and it is important that calcium carbonate supplementation is actually taken with meals to optimise calcium bioavailability under these circumstances.
Calcium retention may be suboptimal due to either decreased calcium absorption, as in vitamin D deficiency, or excessive urinary excretion due to high-protein or high-salt diets. High-oxalate (e.g. spinach) and high-phytate (e.g. chapatis) diets also reduce calcium absorption. Carbonated drinks with high phosphoric acid content may impair calcium absorption, and may also be preferred to milk drinks. Aluminium in antacids taken in excess may increase urinary calcium excretion. Dietary factors that impair calcium retention are listed in Box 19.2.
Phosphorus
The human body contains about 0.8–1.0 kg of phosphorus (11–14 mg/kg fat-free mass), and, like calcium, less than 1% of this circulates in a soluble form. Unlike calcium, however, only 85% of total body phosphorus is in the skeleton, because it also has a major role in soft-tissue growth, with 15% present in muscle and other body tissues. In addition, phosphorus has wide-ranging influences on metabolism, and is an important component of basic molecules such as phospholipids, phosphoproteins, and nucleic acids. Phosphate is also integral to normal muscle function, and has an important role in the synthesis and storage of chemical energy as high-energy phosphate bonds such as adenosine triphosphate (ATP).
Table 19.2 Dietary sources of calcium. Prepared by members of the Department of Dietetics, Monash Medical Centre, Clayton, Victoria, Australia.
Phosphate is an almost ubiquitous component of food, so dietary deficiency is uncommon unless total dietary intake is severely restricted, as may occur in anorexia nervosa or other causes of severe malnutrition, including untreated insulin-dependent diabetes mellitus, or when demand outstrips supply, as may occur in sick, extremely low-birth-weight infants. When it does occur, phosphate depletion has major consequences, affecting neuromuscular, cardiovascular, renal, haematological, and bone metabolism.
Vitamin D metabolism
Reduced calcium gut absorption
- very high-fibre diets;
- excessive carbonated drinks;
- too much alcohol.
Increased calcium urinary excretion
- large servings of protein foods such as meat, chicken, eggs, fish;
- high sodium (salt) intake;
- high caffeine intake (more than 5 cups of coffee or tea daily);
- thiazide and loop diuretics.
Renal 1,25 (OH)2 vitamin D3 production is dependent on multiple organ systems, namely the skin, liver, kidney, gut, and bone. In populations where there are no vitamin D-supplemented foodstuffs, approximately 90% of vitamin D is obtained from vitamin D3 synthesised in the epidermis from 7-dehydrocholesterol (7-DHC), in a reaction catalysed by ultraviolet B (UVB) light with a wavelength of 288 nm. For this reaction to take place, there must be direct exposure to sunlight or other sources of UVB, as this wavelength of UV radiation is not transmitted through glass or clothing. The efficiency of vitamin D synthesis in the skin tends to decrease with ageing, and is a contributory risk factor for vitamin D deficiency in the elderly. The remaining 10% of vitamin D is derived from dietary sources such as cod liver oil, eggs, and oily fish. Skin production of vitamin D is dependent on: (i) skin pigmentation, (ii) latitude, (iii) clothing practices, and (iv) time spent outdoors in direct sunlight. Skin pigmentation influences the efficiency of vitamin D3 production from sunlight exposure, and vitamin D production in the skin is inversely proportional to melanin production, as melanin competes with 7-DHC for UVB protons. Consequently, black-skinned individuals need approximately six times more sunlight exposure than fair-skinned individuals to maintain equivalent vitamin D status. The amount of UVB exposure from sunlight is also inversely proportional to latitude, so that UVB exposure is greater in equatorial regions than in regions at higher latitudes. Furthermore, UVB exposure is greater during summer than winter at higher latitudes, so that the greatest risk for vitamin D deficiency in such regions is in late winter and early spring. The incidence of Vitamin D deficiency is rising in urbanised areas, since as mentioned UVB is not transmitted through glass or clothing, and time spent doing indoor sedentary activities is increasing. These lifestyle changes have also contributed to the rising epidemic of obesity, another risk factor for vitamin D deficiency, likely to be mediated through increased vitamin D catabolism within fat. In the skin, vitamin D3 is bound to vitamin D-binding protein and is transported in the bloodstream to the liver, where it is rapidly hydroxylated.
Both dietary vitamins D2/D3 and skin-synthesised vitamin D3 are hydroxylated in the liver to form 25-hydroxy vitamin D3 (25OH D3), which is the storage form of vitamin D. This hydroxylation step is largely unregulated and vitamin D stores can remain replete for many months without further sun exposure. Vitamin D3 and its metabolites are fat-soluble vitamins and undergo enterohepatic circulation by cosecretion of 25OH D3 and bile salts into the duodenum, with subsequent reabsorption of 25OH D3 within the gut. This reabsorption process may be inhibited by binding of 25OH D3 to dietary phytates and fibre, or in fat malabsorption syndromes such as coeliac disease or cystic fibrosis. Further hydroxylation of 25OH D3 to form 1,25(OH)2D3 occurs in the kidney through the regulation of 1 alpha hydroxylase by PTH, IGF-1, and FGF23, with feedback inhibition of PTH secretion by 1,25(OH)2D3 (Figure 19.3). The 1,25-hydroxylated form of vitamin D (1,25(OH)3D3, calcitriol) is the active metabolite of vitamin D, acts via the vitamin D receptor present in multiple tissues of the body, and should more appropriately be regarded as a hormone rather than a vitamin, as its production is under the tight control of PTH and there is a negative feedback loop whereby calcitriol inhibits PTH secretion in turn (Figure 19.3). Other hormones such as FGF23 and IGF-1 also regulate 1 alpha hydroxylase activity. In recent years, the wide tissue distribution of extrarenal 1 alpha hydroxylase and the role of 1,25 (OH)2 vitamin D in immunomodulation, autoimmunity, and tissue growth are being increasingly recognised, although PTH and FGF23 do not regulate extrarenal vitamin D metabolism. Both renal and extra-renal 1,25(OH)2D3 are inactivated by the enzyme 24 hydroxylase (CYP24R1) to form the inactive metabolite, 1,24,25(OH)2D3. Furthermore, this enzyme also protects the body from vitamin D toxicity, by inactivating 25 OH vitamin D3 to 24,25(OH)2D3.
Table 19.3 Age-related recommended daily intake (RDI) for vitamin D. AI, adequate intake; EAR, estimated average requirement; RDA, Recommended Dietary Allowance; UL, tolerable upper intake level. Holick et al. (2011), copyright 2011, The Endocrine Society.
Gut absorption of both calcium and phosphate is increased by the action of 1,25(OH)2D3 on the gut, both directly and via upregulation of the calcium-binding protein calbindin-D. Although both calcium and phosphate are absorbed throughout the length of the small intestine, the majority of phosphate is absorbed from the jejunum and ileum, and the majority of calcium from the duodenum. To maintain normal mineral-ion homeostasis, particularly in growing children, the RDIs of both vitamin D (Table 19.3) and calcium (Table 19.1) must be met. However, the RDI for vitamin D remains controversial given the wide variation in direct sun exposure around the world, and variable skin-pigmentation, latitude, and lifestyle factors. In Australia, for example, there are no national RDIs for vitamin D, as it is assumed that Australians have adequate sunlight exposure for their vitamin D requirements. The RDIs for vitamin D cited in this chapter are based on the recently published US Guidelines (Table 19.3). Dietary sources of vitamin D are listed in Table 19.4.
The skeletal effects of 1,25(OH)2D3 are complex and beyond the scope of this chapter. However, in broad terms, 1,25(OH)2D3 affects both bone formation and resorption in the ongoing modelling/remodelling process of bone. At a cellular level, osteoblasts express 1,25(OH)2D3 receptors and may modulate the synthesis of a number of osteoblast-derived products, such as alkaline phosphatase (ALP) and osteocalcin, in addition to the stimulation of proliferation of osteoblasts themselves. During pregnancy, the placenta is also an extrarenal source of 1 alpha hydroxylase and 1,25(OH)2D3. In disease states such as sarcoidosis, tuberculosis, and neonatal subcutaneous fat necrosis, hypercalcaemia may develop as a result of excessive and largely unregulated extrarenal 1 alpha hydroxylase-induced 1,25(OH)2D3 production within these tissues.
Table 19.4 Dietary sources of vitamin D. Holick MF. Vitamin D Deficiency. N Engl J Med 2007; 357: 266–281. Copyright © Massachusetts Medical Society.
Source | Vitamin D content |
Natural sources | ![]() |
Cod liver oil | ~400–1,000 IU/teaspoon vitamin D3 |
Salmon, fresh wild caught | ~600–1,000 IU/3.5 or vitamin D3 |
Salmon, fresh farmed | ~100–250 IU/3.5 oz vitamin D3, vitamin D2 |
Salmon, canned | ~300–600 IU/3.5 or vitamin D3 |
Sardines, canned | ~300 IU/3.5 oz vitamin D3 |
Mackerel, canned | ~250 IU/3.5 oz vitamin D3 |
Tuna, canned | 236 IU/3.5 oz vitamin D3 |
Shiitake mushrooms, fresh | ~100 IU/3.5 oz vitamin D2 |
Shiitake mushrooms, sun-dried | ~1,600 IU/3.5 oz vitamin D2 |
Egg yolk | ~20 IU/yolk vitamin D3 or D2 |
Sunlight/UVB radiation | ~20,000 IU equivalent to exposure to 1 minimal erythemal dose (MED) in a bathing suit. Thus, exposure of arms and legs to 0.5 MED is equivalent to ingesting ~3,000 IU vitamin D3. |
Fortified foods | |
Fortified milk | 100 IU/8 oz, usually vitamin D3 |
Fortified orange juice | 100 IU/8 oz vitamin D3 |
Infant formulas | 100 IU/8 oz vitamin D3 |
Fortified yogurts | 100 IU/8 oz, usually vitamin D3 |
Fortified butter | 56 IU/3.5 oz, usually vitamin D3 |
Fortified margarine | 429 IU/3.5 oz, usually vitamin D3 |
Fortified cheeses | 100 IU/3 oz, usually vitamin D3 |
Fortified breakfast cereals | ~100 IU/serving, usually vitamin D3 |
Pharmaceutical sources in the Untied States | |
Vitamin D2 (ergocalciferol) | 50,000 IU/capsule |
Drisdol (vitamin D2) liquid | 8,000 IU/cc |
Supplemental sources | |
Multivitamin | 400, 500, 1000 IU vitamin D3 or vitamin D2 |
Vitamin D3 | 400, 800, 1000, 2,000, 5,000, 10,000, and 50,000 IU |
IU = 25 ng. [Reproduced with permission from M. F. Holick: N Engl J Med 357:266–281, 2007 (3). © Massachusetts Medical Society.]
19.3 Age-appropriate biochemical reference ranges
Specific reference ranges will not be presented, but it is important to be aware of age-appropriate reference ranges which affect the following biochemical parameters.
Alkaline phosphatase
ALP is mostly bone-derived in infants and children, and consequently is normally up to 3–4 times the upper limit of the normal adult reference range in these age groups, with peak levels corresponding to periods of rapid linear growth, as occur in infancy and the pubertal growth spurt. The isoenzyme bone-specific ALP (BSAP) may now be readily measured using commercially available assays.
Phosphate
Phosphate tends to directly correlate with growth rate and is highest in premature infants and during the first few months of life, and continues to be higher than adult reference ranges throughout growth.
Insulin-like growth factor 1
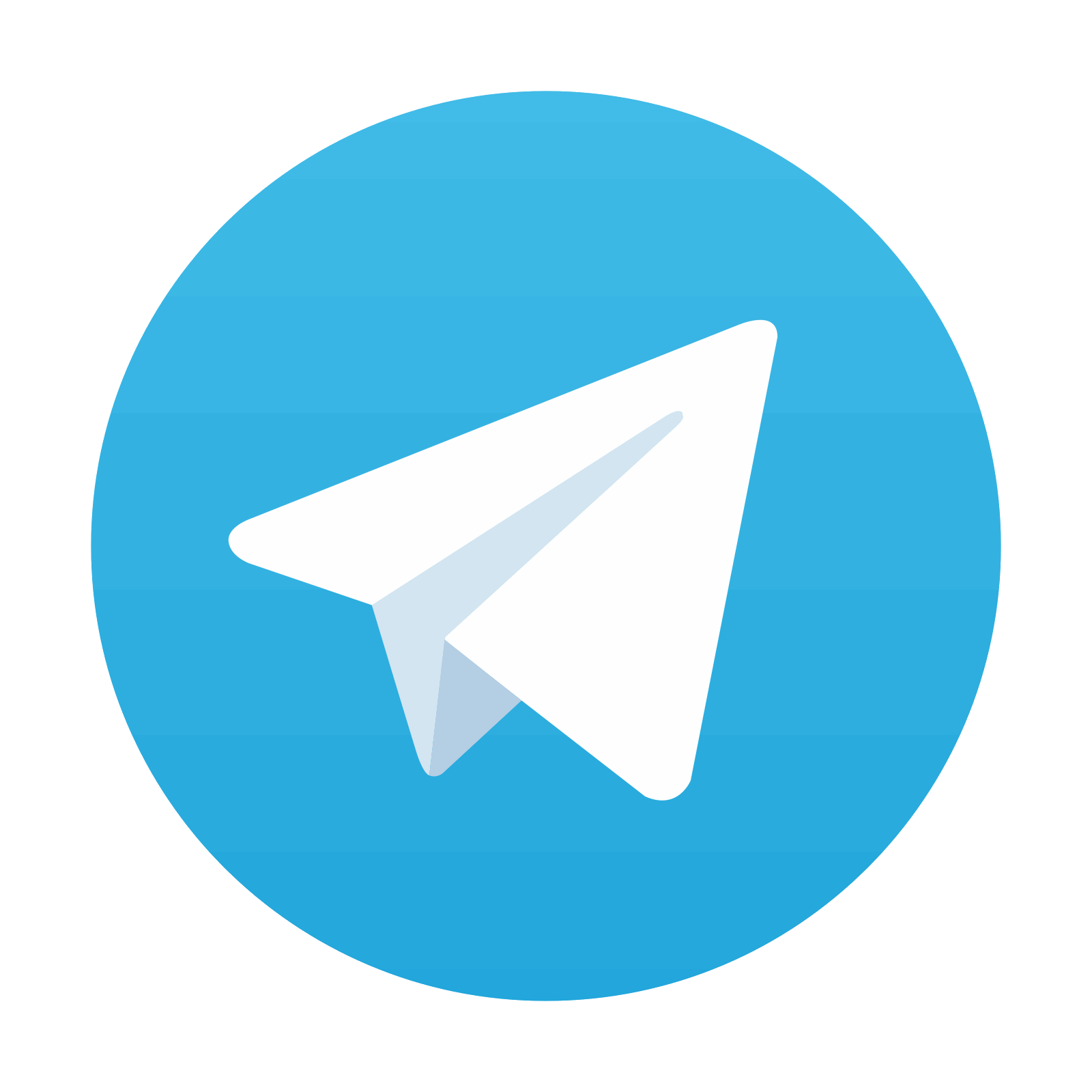
Stay updated, free articles. Join our Telegram channel

Full access? Get Clinical Tree
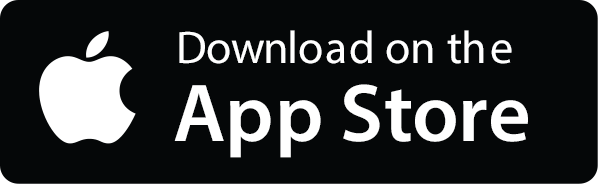
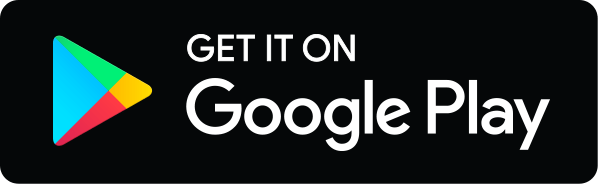