21.1
Introduction
There are substantial pragmatic difficulties in assessing the impact of nutrition in osteoporosis. Bone health and strength are generally stable and subject mostly to long-term exposures. While it is possible to conduct controlled dietary trials (i.e., intake is both controlled and measured), these trials are usually small and of short duration and may have limited impact on outcomes such as bone strength and fracture. Relevant exposures might occur early in the life-course and impact peak bone mass, while clinical outcomes occur late in the life-course. Diets are complex, which further complicate experimental study design. Single food ingredients may have limited to no effect, while assessing larger changes in diet leaves one unable to determine putative causal factors. Thus while such trials provide the best evidence, they will be difficult to generalize to recommendations among a broad population.
It is possible to conduct randomized trials in the community, where the effort is put into intervention aimed at changing the overall dietary pattern (i.e., reduce calories and reduce fat). These trials face different obstacles. The primary such obstacle is dietary adherence. Long-term dietary change is difficult, even with substantial support. Intensive support is costly, limiting the scope of such trials. The combination of need for long-term trials, lack of adherence, and small effect sizes makes such trials expensive and rare.
An alternative strategy to changing the diet itself is to approach the problems using research from dietary supplements as exposure. This strategy is appealing since it allows for blinding, may improve compliance, and allows study investigators to focus on specified exposure. The major problem with such trials is they do not generalize to diet and foods. Supplements and foods differ both in type and source of nutrients, as well as concurrent ingredients, which may result in biological effect modification, thus limiting the applicability of supplement trials. Effect size may also be limited by threshold effects. Null effects would be anticipated for nutrients that are supplemented beyond the required level of intake. Supplements may also lead to side effects that may not occur with food.
Another alternative is to assess the relationship between diet and health using observational cohort studies. Again there are substantial methodological limitations to such an approach. The main limitation is the inherent complexity of the diet. A full diet assessment of an individual includes hundreds of variables, many of which are highly correlated. Dietary variables are also highly correlated with other demographic and lifestyle variables. Thus most of the effort in using cohort studies is finding measures of exposure that summarize the salient aspects of diet and controlling for internal and external confounding.
Finally, it is possible to study diet in animal models. This allows for studying bone health over a “lifetime” under carefully controlled conditions. The main limitation of this approach is whether the animal model reflects the life-course phenotype of human disease. Animal models exist for certain common phenotypes such as postmenopausal osteoporosis; however, these models provide only a simple measure of nutrition exposure, rather than a life-course exposure affected by genetic and epigenetic factors, as well as complex time-varying environmental risk factors.
In summary, there is no ideal way to assess the impact of diet on bone health (or other outcomes). The assessment of causation should therefore be queried using different kinds of studies such as randomized controlled trials (RCTs), cohort studies, and experimental animal studies as a means of triangulation so that strengths of a given design are weighed to support or refute a scientific hypothesis.
Heaney also noted several considerations specific to bone in a previous version of this chapter. The first is that absorption versus excretion for nutrients that are bulk bone constituents, including calcium and phosphorus, can only be assessed under steady-state conditions. Therefore nutritional interventions will have a period of adaptation followed by a new equilibrium. Second, the entire skeleton undergoes very limited change with an overall bone turnover of at most 10%/year; thus the potential impact of any intervention will be limited to areas of bone turnover. Third, bone itself is a functional reserve of nutrients, which is orders of magnitude larger than the pool necessary for essential biological functions of these nutrients; so even in the case of overt nutritional deficiency, the skeletal reserve has ample capacity to compensate with minimal effects. Finally, nutritional influences tend to be more modest than those of drugs with regards to overall bone mineral density (BMD), and effect sizes will typically be quite small.
We will briefly review the association between specific micronutrients and bone health and then review macronutrients and dietary patterns. Central to the review of specific nutrients are dietary reference intakes as established by the Institute of Medicine (IOM) and Food and Nutrition Board. Dietary reference intakes have several components. The first component is the Recommended Dietary Allowance (RDA). The RDA is the daily dietary intake level of a nutrient considered sufficient by the Food and Nutrition Board to meet the requirements of nearly all (97%–98%) healthy individuals in each life stage and gender group. RDAs are conservative; this level means that a healthy individual with no additional risk factors is quite likely to have an intake meeting the requirement for that particular nutrient. The second component is the Estimated Average Requirement (EAR). An EAR is the daily dietary intake level of a nutrient considered sufficient to meet the requirements of half (50%) of the healthy individuals in each life stage and gender group. EARs are used for population health in order to determine the prevalence of those with inadequate nutrient intake. Some nutrients do not have an RDA or EAR due to insufficient evidence. An adequate intake (AI) is used when the RDA/EAR cannot be developed and is the average intake based on observed or experimental intake. The tolerable upper intake level (UL) is the maximum daily intake that is unlikely to cause adverse health effects.
21.2
Calcium, vitamin D, and dairy intake
Perhaps, the most well-known example of nutrient synergy relevant to bone health is that of calcium and vitamin D. Calcitriol (1,25-dihydroxyvitamin D) functions to maintain serum calcium within the body’s homeostatic range. Mechanisms involved include the stimulation of intestinal calcium absorption and coordination with parathyroid hormone (PTH) to mobilize calcium from the bone and to stimulate reabsorption of calcium by the renal distal tubule of the kidney. An important consideration is that a single food may be the primary source of variability for more than one nutrient. In North America, milk is fortified with vitamin D, and this makes milk intake one of the primary determinants of vitamin D intake. Milk is also a key source of calcium in the North American diet. The resulting conundrum is that dietary calcium and vitamin D should not be viewed in isolation due to the common source, but this common source also means that dietary intakes of these nutrients are highly correlated.
21.2.1
Calcium
The IOM estimated dietary reference intakes for calcium and vitamin D in 2011 . The comprehensive review was based on both skeletal and extraskeletal outcomes. The recommended intake levels were based on setting levels for optimal skeletal outcomes, including prevention of osteoporosis, whereas the evidence was not deemed sufficient to inform requirements for optimal nonskeletal outcomes. The IOM RDA for calcium varied by life stage and sex and is presented in Table 21.1 . The establishment of dietary reference intakes was based on a substantial body of research, which we will only briefly summarize here.
Age (years) | Male (mg) | Female (mg) | Pregnancy (mg) | Lactation (mg) |
---|---|---|---|---|
1–3 | 700 | 700 | ||
4–8 | 1000 | 1000 | ||
9–13 | 1300 | 1300 | ||
14–18 | 1300 | 1300 | 1300 | 1300 |
19–30 | 1000 | 1000 | 1000 | 1000 |
31–50 | 1000 | 1000 | 1000 | 1000 |
51–70 | 1000 | 1200 | ||
>70 | 1200 | 1200 |
Dairy products are the predominant source of dietary calcium in the United States. Other important dietary sources are legumes, green leafy vegetables, tofu, almonds and seeds, and fortified foods (e.g., orange juice and milk alternatives). Bailey et al. assessed calcium intake from diet alone and from diet and supplements from the 2003–06 National Health and Nutrition Examination Survey (NHANES), a nationally representative, cross-sectional survey of noninstitutionalized, civilian the US residents . Mean dietary calcium intake among nonusers of calcium supplements was 727 mg/day in women and 1005 mg/day in men, with slightly higher dietary intakes in supplement users. There was a high prevalence of inadequate intake for the general population, and based on dietary intake, nearly 90% of women ages 50 and older who were not using supplements were below the EAR of 1200 mg. Estimates of inadequate intake may have changed somewhat since this earlier survey due to general increase in the availability of fortified foods and the use of supplements . Blumberg et al. reported that 45.8% of adults used dietary supplements based on the more recent 2009–12 NHANES . Supplement users again had higher dietary intakes of most nutrients. Supplement nonusers rarely had intakes above the UL for calcium (2000 mg); however, 9% of men and women ages 51–70 had total calcium intake above the UL. Thus calcium supplements are often taken in doses higher than necessary to meet the RDA. Evidence suggests that for calcium intake, there is a substantial risk of both inadequate intake (among those without supplement use) and excessive intake.
Calcium is essential for cellular function, and circulating levels of calcium are under tight homeostatic control. Adaptive mechanisms include increased calcium absorption from the gut, decreased calcium excretion via the kidneys, and mobilization of calcium from bone. In general, those with lower dietary intake will have higher fractional calcium absorption. If dietary calcium intake is inadequate, bone resorption will increase in order to extract calcium from storage in the skeleton and thus maintain plasma calcium homeostasis. The skeleton is therefore a sizable reserve for calcium required for cellular functions, and therefore nutritional deficiency has limited impact on serum calcium but may lead to bone loss through stimulation of PTH-mediated bone remodeling.
Net calcium balance operates in a range that depends largely on genetics and stage of life. During growth, there is a determined upper bound on skeletal growth and calcium deposition, and this upper bound corresponds to net calcium balance (with associated intake). Calcium intake above this level does not further increase skeletal growth. However, calcium intake during growth is one determinant of peak bone mass. Each period of adulthood is also associated with maintenance and remodeling with associated maximal calcium balance. During some periods of adulthood, such as menopause and late adulthood, this maximal calcium balance is negative, and there is bone loss even with ample calcium intake. Calcium intake above the level necessary for maximal calcium balance does not further impact maintenance and remodeling. These considerations were the foundation for the determination of dietary reference intakes.
Tai et al. performed a review of RCTs indexed prior to September 2014 comparing the effect of calcium (as a part dietary intervention or as a supplement) on BMD among various populations (community-dwelling, nursing-home residents, general population, and high fracture risk) . Those in the calcium group had higher BMD than those in the control group at multiple skeletal sites, but the effects were small. For dietary trials, those in the calcium group had 0.7%–1.8% higher BMD than controls at the femoral neck, total hip, lumbar spine, and total body after 2 years. For calcium supplement trials, those in the calcium group had 0.7%–1.8% higher BMD than controls at all skeletal measurement sites after 2 years. Seven trials included both a dietary calcium group and a calcium supplement group, and there was no overall difference between dietary calcium and supplemental calcium. There were no consistent differences between trials using calcium monotherapy and those using calcium and vitamin D, nor were there differences by dose, baseline dietary calcium intake, or baseline 25(OH)D status.
Bolland et al. performed a parallel review of studies indexed prior to September 2014 on the association between dietary calcium, milk intake, dairy intake, or calcium supplements and fractures among various populations . There were only two dietary calcium trials, and they were too small to look at fracture outcomes. For calcium supplements, there were 20 trials with 58,573 randomized participants, and the relative risk (RR) comparing the calcium versus the control intervention was 0.89 (95% CI: 0.81, 0.96) for all clinical fractures. There were 12 studies with 48,967 participants that assessed radiographic vertebral fractures, and the RR comparing the calcium versus the control intervention was 0.86 (95% CI: 0.74, 1.00). Similar comparisons for hip fracture and forearm fractures were null. In summary, findings from this analysis provide very limited support for the use of calcium supplements for the prevention of fracture in the general population.
21.2.2
Vitamin D
Vitamin D has two predominant forms, vitamin D2 (ergocalciferol) and vitamin D3 (cholecalciferol). Dietary sources of vitamin D2 are mushrooms and fortified foods. Dietary sources of vitamin D3 include fatty fish, eggs, and liver. Supplements are available for both forms of vitamin D, with fish oil supplements providing vitamin D3.
For vitamin D the RDA levels are 600 IU for both sexes throughout childhood, early adulthood, and for those under 70 years and increased to 800 IU for those 71 and older . Of note, the RDA levels for vitamin D assume minimal sun exposure. These levels would be most relevant for subgroups, including those who live in northern latitudes and those with low habitual sun exposure (e.g., full-body covering or indoors). The UL for vitamin D is set at 4000 IU.
The endogenous cutaneous production of vitamin D complicates the assessment of dietary vitamin D. Exposure of skin to ultraviolet radiation leads to the production of 7-dehydrocholesterol that is subsequently converted to previtamin D3 and then to vitamin D3 (cholecalciferol). Endogenous production depends on sufficient radiation and exposure to sunlight and therefore varies by season, with stronger variation for those in northernmost latitudes. Circulating vitamin D (D2 or D3) is converted in the liver to 25-hydroxy vitamin D [25(OH)D]. Serum 25(OH)D may be converted in the kidney to 1,25-dihydroxyvitamin D or calcitriol, the active form of vitamin D, under the control of the ambient PTH level that regulates the renal 1-alpha hydroxylase. Alternatively, it may be converted to 24,25-dihydroxyvitamin D and hence removed from the pool for conversion to calcitriol. Conversion to calcitriol requires an adequate pool of 25(OH)D.
Serum 25(OH)D has been used as a measure of vitamin D status, in particular by the IOM committee that sets reference levels for 25(OH)D analogs to those for intakes (i.e., RDA level and EAR level). The assessment of the dietary requirement of vitamin D is complicated due to additional factors such as fat storage, protein binding, and the multiple forms of vitamin D. Some early studies have suggested that 25(OH)D may be sequestered in fat tissue with limited release . A recent study considered the impact of vitamin D storage on subsequent levels of 25(OH)D and found that release may result in nominally higher levels of 25(OH)D for up to a year after vitamin D intake intervention ceased . Thus dietary requirements may be confounded by seasonal sunlight exposure in combination with fat storage. Total serum 25(OH)D is a marker of current vitamin D status, but a large part of this is bound to either vitamin D–binding protein (VDBP) or albumin, while a small fraction (<1%) remains unbound. Vitamin D is strongly bound to VDBP, but it is loosely bound to albumin, and therefore a substantial portion of total 25(OH)D may still be considered bioavailable. Selective binding affinity and differing levels of circulating VDBP or albumin might result in normal levels of active vitamin D in spite of lower levels of 25(OH)D, and thus total 25(OH)D level should be interpreted with caution.
Bailey et al. analyzed vitamin D intake from food, supplements, and serum 25(OH)D in a stratified random sample of participants in the NHANES 2005–06 survey . The mean estimated intake from food alone was between 204 and 288 IU/day by age group in males and between 144 and 276 IU/day in females. Inclusion of supplement intake increased overall mean intake, with resulting ranges of 264–428 IU/day in males and 200–400 IU/day in females. In particular, the mean dietary intake alone was well below the RDA levels set by the IOM (600 IU in most strata) and in many cases below the EAR (400 IU in most strata). In contrast, the mean serum 25(OH)D levels were all above 55 nmol/L. While dietary intake of vitamin D is often deemed inadequate, the strong assumption of no endogenous production may not apply generically since adequate serum 25(OH)D levels (i.e., above the RDA level set by the IOM) were achieved despite low prevalence of AI.
Bolland et al. identified 81 RCTs that assessed supplemental vitamin D and musculoskeletal outcome among various populations (community-dwelling, nursing-home residents, general population, high fracture risk, and disease with impact on bone turnover) . There were 42 trials that reported fracture outcomes, 37 of which reported fall outcomes and 41 reported BMD. In pooled analysis, based on final time point, vitamin D had a very limited effect on BMD. Between-group differences were 0.34% (95% CI: 0.13, 0.55) at the total hip, 0.76% (95% CI: 0.42, 1.09) at the femoral neck, and 0.25% (95% CI: 0.00, 0.49) at the lumbar spine. In pooled analyses, vitamin D had no effect on total fracture, hip fracture, or falls. Results did not vary by dose (low-dose regimens vs trials using doses greater than 800 IU/day). In summary, findings from this analysis did not support the use of vitamin D supplements, either alone or in combination with calcium for the prevention of fracture in the general population, and effects on BMD were limited.
21.2.3
Supplemental calcium and vitamin D
Zhao identified 33 RCTs that assessed supplemental calcium and vitamin D (separately and concurrently) and musculoskeletal outcomes with the main outcome as hip fracture. There was no statistically significant association between combined calcium and vitamin supplementation and fracture, vertebral fracture, nonvertebral fracture, or hip fracture. One notable limitation is that the study authors considered only trials among community-dwelling older adults, many of whom had sufficient dietary intake of calcium and vitamin D . Two trials were primary sources of fracture outcomes in this metaanalysis. One key trial was the Randomized Evaluation of Calcium and Vitamin (RECORD) trial . The RECORD trial was a secondary prevention trial comparing 800 IU vitamin D, 1000 mg calcium both separately and in combination versus placebo among men and women age 70 and older with prior low-trauma fracture. Participants receiving calcium supplementation (with or without vitamin D) versus placebo had a hazard ratio (HR) of 0.94 (95% CI: 0.81, 1.09) for low-trauma fracture, 0.89 (95% CI: 0.77, 1.02) for falls and 0.99 (95% CI: 0.86, 1.15) for all fracture. Poor compliance with calcium supplements was noted with gastrointestinal symptoms, a frequent precipitating complaint. While compliance was low, a per-protocol analysis did not alter the findings.
Another key trial was the Women’s Health Initiative (WHI), one of the largest RCTs of supplemental calcium and vitamin D . The WHI trial compared treatment of 1000 mg/day calcium carbonate and 400 IU vitamin D to placebo in 36,282 postmenopausal women 50–79 years old over 7–11 years. The treatment group showed a modest improvement in hip BMD but no reduced fracture risk compared to the placebo group, according to the intent-to-treat analysis. Participants receiving calcium plus vitamin D supplementation had an HR of 0.88 (95% CI: 0.72, 1.08) for hip fracture, 0.90 (95% CI: 0.74, 1.10) for spine fracture, and 0.96 (95% CI: 0.91, 1.02) for all fracture.
Sensitivity analysis to assess effect of nonadherence by censoring data from women who ceased to adhere to the study medication changed the HR for hip fracture to 0.71 (95% CI: 0.52, 0.97) . The WHI trial also permitted the use of personal calcium supplements in addition to study treatment, and this crossover may have attenuated overall effect. The HRs by supplement use at baseline were HR=0.70 (95% CI: 0.51, 0.98) for nonusers of calcium supplements, 0.87 (95% CI: 0.61, 1.24) for those taking less than 500 mg/day, and 1.22 (95% CI: 0.83, 1.79) for those taking 500 mg, or more per day . The overall effect was null, but some of this would be attributable to dilution of effect by use of personal supplements and lack of adherence. The interpretation of this large trial depends on the target population. The baseline mean calcium intake was 1150 mg/day, well above the EAR. Thus the trial was not limited to those with inadequate calcium intake. The ideal target population may also depend on other factors. With regards to the WHI trial, potential effect modification was noted for age, falls, and hormone replacement therapy. The HRs by baseline age were HR=2.17 (95% CI: 1.13, 4.18) for those aged 50–59 years, 0.74 (95% CI: 0.52, 1.06) for those aged 60–69 years, and 0.82 (95% CI: 0.62, 1.08) for those aged 70–79 years .
The trial results are particular to the study intervention and population but may be used in assessing marginal impact of increasing calcium and vitamin D intake in the general population. We have presented the intent-to-treat result together with noted limitations due to adherence. Other metaanalyses have explicitly used analysis based on adherence and nonuse of personal supplements, such as that of Prentice et al. , with resulting interpretation more favorable to use of supplement for fracture prevention . Another notable limitation bears repeating, and, that is, calcium and vitamin D are nutrients, with necessarily limited effect sizes and possible thresholds above which there is no effect at all . Very few trials systematically assessed dose–response effects or limited to those with inadequate intake. Smith et al. observed a possible nonlinear association between vitamin D dose and fall risk, an effect that might contribute to increased fracture risk at high dose .
Calcium supplements can cause a number of gastrointestinal side effects, particularly constipation . Further considerations regarding these trials are that supplement use will increase the risk of exceeding the UL of calcium , and excessive calcium intake may increase the risk of nephrolithiasis, cerebrovascular accident, and myocardial infarction . We note, however, that other reviews have assessed potential adverse outcomes and found no association between calcium supplement use and cardiovascular outcomes when following recommended intakes .
The role of dietary calcium and vitamin D as established by dietary reference intakes is not superseded by RCTs on supplements. The current NOF Clinician’s Guide to Prevention and Treatment of Osteoporosis contains recommendations for adequate calcium and vitamin D through the use of supplements, if patients are unable to consume enough calcium and vitamin D from food . Supplements are not a replacement for food sources of nutrients, as food sources have complementary nutrients. However, the supplemental calcium may be warranted in the presence of inadequate intake not amenable to dietary modification or use of fortified foods/beverages. Due to limited dietary sources of vitamin D, the use of fortified foods or supplemental vitamin D is warranted for those with conditions (age, race, geography, season, and clothing) limiting natural endogenous production.
21.2.4
Dairy consumption for bone health
The 2015–20 Dietary Guidelines for Americans recommend 3 c of low-fat/skim milk (or equivalent dairy products or fortified soy milk) per day for bone health in persons 9 years old and above . Dairy products not only are considered ideal vehicles for calcium delivery but also provide several additional bone-enhancing nutrients such as potassium, protein, zinc, magnesium, phosphorus, vitamin A, and vitamin D (with fortification). Dairy products are economical , widely available and provide calcium and phosphorus in a ratio favorable to bone . Malnutrition among the elderly is linked to hip fracture pathogenesis .
Despite the logic applied to construct the dietary guidelines, the issue of how much and which sources is not without controversy. As noted previously, the assessment of dairy intake and dietary calcium and vitamin D intake overlaps, since dairy products are the predominant source of both nutrients. Some metaanalyses have focused specifically on milk or dairy products, while others have looked at summarizing the association by calcium or vitamin D intake. The association between dairy intake and fracture outcomes is likely to be modest at best, and many important fracture outcomes (e.g., hip fracture) are rare, and thus individual studies may well fail to find an effect.
Previous reviews have noted that the positive evidence from studies of dairy on bone health exceeds negative or neutral findings . Bolland et al. present a contrary view , albeit without performing a formal metaanalysis. In 2011 Bischoff-Ferrari et al. performed a literature review to assess the relationship between milk intake and the risk of hip fracture in men and women . A total of seven separate studies were identified, none of which reported statistically significant findings. The pooled RR per daily glass of milk was 0.91 (95% CI: 0.81–1.01), albeit with substantial heterogeneity. The summary estimate (and heterogeneity) was driven in large part by the results from the study by Michaelsson et al. in the Swedish Mammography Cohort . Notably, in this large study of Swedish women, there was a positive, but not statistically significant, association between milk intake and fracture risk. Other limitations of interpretation may result from the particular parameters of a given study. For example, the individual participant metaanalysis by Kanis et al. on milk intake was motivated by screening for risk factors . The aim of this study was to determine whether low milk intake was an important risk factor for fracture and not to determine whether this link might be causal. For the latter analysis, one would make sure that possible confounders were assessed and included in the model.
In 2018 Bian et al. performed a literature review to assess the relationship between dairy intake and the risk of hip fracture in men and women . A total of 18 studies, 10 cohort studies and 8 case–control studies, meeting the criteria were identified. For milk the pooled RR from cohort studies comparing the highest versus lowest category of milk intake was 0.91 (95% CI: 0.74–1.12). The pooled RR from cohort studies comparing the highest versus lowest category of yogurt intake was 0.75 (95% CI: 0.68–0.86), while the pooled RR from cohort studies comparing the highest versus lowest category of cheese intake was 0.68 (95% CI: 0.61–0.77). The estimates for case–control studies were consistent in magnitude and direction to those of cohort studies, albeit with higher uncertainty. The summary estimate (and heterogeneity) for the association between milk and fracture was again driven in large part by the results from the Swedish Mammography study . In a 2014 updated analysis, Michaelsson et al. reported an association between higher milk intake and increased fracture incidence in both men and women, as well as increased mortality in women, thus suggesting a harmful effect of milk . This study was based on longer follow-up of the Swedish Mammography Cohort included in the Bischoff-Ferrari metaanalysis. These results have not been replicated in other cohorts and might be attributable to residual confounding related to specific patterns of dairy consumption in Swedish women . These results were also in slight contrast to earlier findings from the same cohort HR 1.03 (95% CI: 0.98, 1.08) per glass . Other observational studies not included in either metaanalysis further support a benefit of dairy products on bone health. A large study looking at calcium intake (as opposed to milk intake) in Great Britain found higher calcium intake was associated with lower fracture risk when comparing women with calcium intakes of less than 525 mg/day to women with calcium intakes at least 1200 mg/day .
Intervention studies address some of the biologic pathways. Three servings of yogurt per day increased overall diet quality and decreased urinary N-telopeptide of type 1 collagen (NTx, a bone resorption marker) in older women as early as 7–10 days after the start of treatment . A prospective crossover trial of 30 healthy postmenopausal women compared a diet with 600 mg calcium/day for 6 weeks to a milk treatment (which increased calcium intake to 1200 mg/day) for 6 weeks . Results suggested that the milk supplement suppressed PTH that subsequently inhibited bone turnover, as evidenced by reduced carboxy-terminal crosslinked telopeptide of type 1 collagen (CTX, a resorption marker), aminoterminal propeptide of type 1 collagen (P1NP, a formation marker), and osteocalcin (a formation marker). A 2-year RCT of 173 postmenopausal Chinese women compared effects on BMD of high calcium skim milk powder (1200 mg calcium/day) to a normal diet . Though both groups lost bone, the milk treatment significantly attenuated BMD loss for the total body, lumbar spine, femoral neck, and hip. A 30-month study of 66 postmenopausal women in Greece examined the effects of a dairy treatment to a control diet . Significant increases in BMD of the arms, total spine, and total body were found for the dairy group compared to the control group. In addition, the dairy group had a significant increase from baseline for lumbar spine BMD.
Few published studies have directly compared equivalent amounts of calcium/vitamin D from dairy foods versus supplements. One study involving 30 healthy, postmenopausal women compared 24 oz of low-fat milk per day for 24 months versus control (no milk treatment), and data were compared to a similar study by the same group that used calcium carbonate . Both the milk and the calcium carbonate improved calcium balance, but the calcium carbonate suppressed overall bone remodeling to a greater extent than the milk. Another 2-year study of postmenopausal women ( n =168) evaluated the effects on BMD of three calcium treatments (skim milk powder, calcium lactate gluconate tablet, and calcium lactate gluconate tablet plus 4 hours of weight-bearing exercise per week) or placebo . All calcium treatments contained 1000 mg of elemental calcium, and all calcium treatments significantly attenuated bone loss at certain sites compared to placebo. Differences between the calcium tablet and milk powder were not significant, and researchers concluded that the milk powder and calcium carbonate were essentially equivalent in preventing bone loss. However, vitamin D was not included in this study, and results have not been confirmed in any recent investigations. An RCT of adolescent girls ( n =195) compared calcium carbonate tablets, calcium carbonate/vitamin D3 tablets, a high dairy diet (low-fat cheese and yogurt) or placebo on bone health outcomes . Per the intent-to-treat analysis, the dairy food treatment increased cortical thickness of the tibia to a greater extent than the other treatments, and the dairy food treatment group gained significantly more total body BMD than the placebo group.
The complex nature of osteoporosis etiology, differences and limitations in study design, and often conflicting results of research studies makes it a challenge to understand the proper roles for calcium supplements and dairy foods in the prevention of osteoporosis. The interpretation of the cohort studies may well rely on the overall body of evidence and biologic plausibility. The evidence is not strongly supportive of a novel hypothesis, but it is also not sufficient to overturn the predominant paradigm that dietary calcium and vitamin D (and in particular dairy products) are related to bone health. In term of population measures, there are other sources of the requisite nutrients, as demonstrated by dietary patterns around the world.
21.3
Other nutrients and bone health
21.3.1
Vitamin A
Vitamin A is essential for normal growth, vision, and immune function. Vitamin A exists both as preformed vitamin A (retinol and retinyl esters) and provitamin forms (alpha-carotene, beta-carotene, and beta-cryptoxanthin). Dietary sources of preformed vitamin A (largely retinyl palmitate) include fish, eggs, dairy, and liver, while dietary sources of provitamins include fruits and vegetables such as carrots, broccoli, cantaloupe, and squash. The RDA for vitamin A varies by life stage and sex and is shown in Table 21.2 .
Age (years) | Male (μg RAE) | Female (μg RAE) | Pregnancy (μg RAE) | Lactation (μg RAE) |
---|---|---|---|---|
1–3 | 300 | 300 | ||
4–8 | 400 | 400 | ||
9–13 | 600 | 600 | ||
14–18 | 900 | 700 | 750 | 1200 |
19–30 | 900 | 700 | 770 | 1300 |
31–50 | 900 | 700 | 770 | 1300 |
51+ | 900 | 700 |
Reference intakes are shown in retinol activity equivalents (RAE), where 1 RAE=1 μg, retinol=12 μg, beta-carotene=24 μg, and alpha-carotene or beta-cryptoxanthin. The UL for vitamin A also varies by life stage and sex and ranges from 600 μg RAE among children of both sexes to 3000 μg RAE for adults. Significant health risks may occur from excess vitamin A, often as supplements, rather than dietary provitamin A . Animal studies have suggested the high levels of retinoic acid, the biologically active form of vitamin A, may impair osteoblast function . Thus it is possible that high vitamin A might be associated with adverse bone health.
Findings from studies on vitamin A and fracture have been inconsistent. Feskanich et al. observed an increased hip fracture risk among postmenopausal women in the highest quintile of vitamin A intake . In contrast, Caire-Juvera et al. reported no association between quintile of total vitamin A intake or retinol intake and the risk of fracture (hip fracture or total fracture) . A study based on NHANES data found increased risk of hip fracture among those in the lowest or highest quintile of serum retinol compared to those with serum retinol in the third quintile . Michaelsson et al. found that hip fracture risk was increased among men with the higher of serum retinol among older Swedish men . In contrast, Holvik et al. reported that there was no association between high serum retinol and risk of hip fracture among older Norwegians with consideration of current vitamin D status and possible interaction . Several considerations preclude definitive interpretation. There are multiple dietary sources of vitamin A, and these sources have substantially different nutrient profiles. In addition, the conversion of provitamin A to retinol is variable and diet dependent. Avoidance of excess vitamin A from supplements may be prudent while ensuring at least adequate dietary vitamin A intake.
21.3.2
Vitamin C
Vitamin C, known as ascorbic acid in its reduced form, is found in a variety of fruits and vegetables, such as citrus fruits, tomatoes, potatoes, cruciferous vegetables, strawberries, and spinach. The RDA for adults is 90 mg/day for men and 75 mg/day for women, though it is recommended that smokers consume an additional 35 mg/day; the UL for adults is 2 g/day . Vitamin C deficiency (scurvy) is rare in developed countries, but individuals at greatest risk include those who consume minimal fruits and vegetables, substance abusers, and those on severely restrictive diets.
Vitamin C has several known mechanisms of action in bone. Work in animal models (such as knockout mice) has suggested that vitamin C deficiency can impair osteoblast function and subsequent bone formation, resulting in low BMD, low trabecular number, and reduced cortical thickness . In vitro and in vivo studies have shown that vitamin C promotes the expression of genes that regulate the differentiation of bone cells, including chondrocytes, osteoblasts, and osteoclasts. Furthermore, vitamin C promotes bone formation by inducing the expression of growth factors and hormones such as transforming growth factor-β, osteopontin, and estrogen receptor-α. Also, the antioxidant properties of vitamin C can prevent osteoclast differentiation, thus impeding bone resorption. In addition, vitamin C serves as a cofactor for prolyl and lysyl hydroxylases that are enzymes necessary for the formation and maturation of collagen in the bone matrix.
In 2014 Finck et al. published a literature review of studies related to vitamin C and the prevention of osteoporosis and bone fracture . Results suggested a positive role of vitamin C in bone health, though the authors noted considerable heterogeneity across all of the studies, making comparisons challenging. Eighteen cross-sectional studies were reviewed, and these studies varied the most in methodology, dietary exposure and assessment, outcome measures, study populations, and confounding factors, in particular intake of fruits and vegetables. Consequently, results across the cross-sectional studies were inconsistent, with some studies suggesting that higher dietary intake of vitamin C may be associated with higher BMD by 3%–5%, and other studies report no significant associations between vitamin C intake and BMD. The three case–controlled studies that were included in the review consistently showed that patients with osteoporosis and/or fracture had significantly lower plasma vitamin C levels compared to controls. Three longitudinal studies of dietary vitamin C intake were included in the review and showed mixed results: Greater vitamin C intake was associated with attenuated total hip BMD loss in older British women. In a cohort of older Americans, higher vitamin C intake was associated with less BMD loss at the lumbar spine and trochanter (but not the femoral neck or radial shaft) in men. Both supplemental vitamin C and total vitamin C intake (diet plus supplements) were associated with reduced risk of hip fracture over 15–17 years of follow-up in a cohort of older American men and women. Three small ( n <100) RCTs using supplemental vitamin C were included in the review, and results were equivocal in regards to BMD and bone turnover marker outcomes.
In 2018 Malmir et al. published a metaanalysis of observational studies of vitamin C intake, BMD and risk of fracture, and osteoporosis . Analyses of the pooled data suggested positive associations between greater dietary vitamin C intake and femoral neck BMD and lumbar spine. The association between dietary vitamin C intake and risk of hip fracture was nonsignificant, but there was a 33% lower risk of osteoporosis with greater dietary vitamin C intake. Like the review by Finck et al., this metaanalysis detected significant heterogeneity between studies, which was attributed primarily to study design, sex, and age of participants.
21.3.3
Vitamin K
Vitamin K has two predominant forms, vitamin K1 (phylloquinone) and vitamin K2 (menaquinone). Dietary sources of vitamin K1 include kale, spinach, broccoli, herbs, kiwi fruit, grapes, soybean oil, and canola oil. Dietary sources of vitamin K2 include eggs, cheese, and meat, but it is also synthesized by intestinal bacteria. The endogenous production of vitamin K complicates the assessment of dietary vitamin K. AI may depend on individual phenotype and intestinal microbiota. In addition, vitamin K status is affected by certain medications, such as anticoagulants, and these medications may result in vitamin K deficiency. Plasma vitamin K is responsive to diet, but as a single measure may not reflect overall intake, since foods that are high in vitamin K may not be a regular part of the diet. An EAR has not been established for vitamin K due to insufficient evidence. However, the AI levels for sex and life stage are given in Table 21.3 . Analysis of NHANES data has shown that a majority of the US population has intakes below estimated AI levels. These results are certainly of concern but should be viewed in the context of limited evidence for the establishment of thresholds as well as endogenous synthesis.
Age | Male (µg) | Female (µg) | Pregnancy (µg) | Lactation (µg) |
---|---|---|---|---|
Birth to 6 months | 2 | 2 | ||
7–12 months | 2.5 | 2.5 | ||
1–3 years | 30 | 30 | ||
4–8 years | 55 | 55 | ||
9–13 years | 60 | 60 | ||
14–18 years | 75 | 75 | 75 | 75 |
19+ years | 120 | 90 | 90 | 90 |
Vitamin K has multiple functions with respect to bone. Vitamin K is a cofactor of the enzyme gamma-carboxylase that is necessary for the activation of osteocalcin, matrix gla protein (MGP), Gla-rich protein, protein S, and growth arrest–specific gene 6 protein. Vitamin K also stimulates osteoblastogenesis and inhibits osteoclastogenesis through nuclear factor-κB. In addition, vitamin K activates MGP, a potent inhibitor of vascular calcification.
In 2008 Cheung et al. reported a clinical trial assessing the effects of vitamin K supplementation on BMD and fracture . Daily vitamin K supplementation of 5 mg vitamin K1 did not protect against age-related bone loss. The trial was not designed to assess fracture outcomes, but fracture risk was lower among those in the vitamin K supplementation than the control arm. In 2012 Fang et al. performed a metaanalysis on RCTs assessing vitamin K and BMD . There was a significant effect favoring the vitamin K arm at the lumbar spine, but not at the femoral neck. Major limitations were noted, since subgroup analysis revealed differences by ethnicity, sex, and type of vitamin K. In 2015 Huang et al. performed metaanalysis on vitamin K and osteoporosis (both BMD and fractures) . This metaanalysis replicated the BMD findings of the previous study. Seven trials had fracture data, with the largest study finding no effect. Thus evidence of the effect of vitamin K alone on fracture outcomes is inconclusive. There is some evidence of vitamin K as biologically active with respect to bone, but it is less clear how dietary K is related to bone health outcomes.
21.3.4
B-vitamins
Table 21.4 summarizes the functions of the B-vitamins, as well as dietary requirements, ULs, food sources, and groups at risk for deficiency .
Functions | RDA | UL | Food sources | Persons at-risk for deficiency | |
---|---|---|---|---|---|
B 1 (thiamin) |
|
| N/A | Whole grains, wheat germ, yeast extract, and pork |
|
B 2 (riboflavin) | Oxidation–reduction reactions in metabolic pathways and energy production |
| N/A | Fortified dairy, cereal, meat, fatty fish, dark green vegetables |
|
B 3 (niacin) |
|
| 35 mg/day for adults 19 and older | Yeast, meat, cereals, legumes, seeds, milk, green leafy vegetables, fish, coffee, tea |
|
B 6 (pyridoxine) | Bioactive form, PLP, acts as a cofactor for >100 enzymes in numerous metabolic reactions | For adults 50 and older: 1.7 mg/day (men) and 1.5 mg/day (women) | 100 mg/day for adults 19 and older | Meat, whole grains, vegetables, bananas, and nuts |
|
B 9 (folate) |
| 400 mg/day for adult men and nonpregnant adult women | 1 mg/day for adults 19 and older | Citrus fruits, dark leafy vegetables, and legumes |
|
B 12 (cobalamin) | Cofactor for enzymes in metabolism of homocysteine and in the Krebs cycle | 2.4 mg/day for adults 50 and older | N/A | Meat, seafood, fish, dairy, and eggs |
|
There is little evidence linking B 1 (thiamine) or B 3 (niacin) directly to bone, but nutritional deficiency may lead to conditions with increased fall risk and hence fracture. Vitamin B 2 (riboflavin) has been shown in vitro to increase alkaline phosphatase activity and decrease receptor activator of nuclear factor-kappa ligand (RANKL) activity and therefore may be related directly to bone mineral metabolism . Several studies have reported an interaction between a genetic polymorphism C677T and vitamin B 2 associated with BMD and fracture risk, but the implications of these findings are unclear . Historically, experimental and some observational evidence suggested that B-vitamins could have therapeutic benefit for bone due to their relationship with homocysteine metabolism . In a metaanalysis of 12 trials, folic acid–based supplementation (without/with B 6 and B 12 cosupplementation) has been shown to lower serum homocysteine . High serum homocysteine is a risk factor for hip fracture, both alone and after adjusting for B 6 and folate intake, but attenuated after adjusting for B 12 intake . Vitamin B 6 also serves as a cofactor for the enzyme lysyl oxidase that is integral to collagen cross-linking during bone formation.
Van Wijngaarden et al. conducted a metaanalysis of four prospective studies ( n =7475) and reported a modest reduction in fracture risk with increased intake of vitamin B 12 , but data were inadequate to draw conclusions about folate and fracture risk . More recent intervention studies have been in general negative. The B-PROOF trial compared a daily dose of 500 mg vitamin B 12 plus 400 mg folic acid versus placebo for 2 years in older adults . Osteoporotic risk did not differ between groups, but for subjects older than 80 years, risk of osteoporotic fracture was lower in the treatment group. Ruan et al. published a metaanalysis in 2015 of four RCTs and found no significant effect on fracture risk with vitamin B supplementation (single or combined use of vitamin B 6 , folate, and vitamin B 12 ). More recently, Garcia et al. examined data from two large RCTs ( n =6837 women and men) and concluded that treatment with 0.8 mg/day folic acid plus 0.4 mg/day vitamin B 12 was not associated with hip fracture risk during the 3-year trial (or during the 10 years of follow-up) . Furthermore, treatment with 40 mg/day vitamin B 6 had no significant effect on hip fracture risk during the trial but actually increased hip fracture risk during the follow-up. In an ancillary study to the Women’s Antioxidant and Folic Acid Cardiovascular Study (WAFACS; n =4810 women), Stone et al. reported no significant effects of vitamin B supplementation (2.5 mg/day folic acid, 50 mg/day vitamin B 6 , and 1 mg/day vitamin B 12 ) on risk of nonspine fractures; vitamin B supplementation also had no significant effects on markers of bone turnover . Heterogeneity (in terms of population and supplementation dosage) should be noted across these trials, but the predominately null findings overall suggest that vitamin B supplementation is not effective in reducing fracture risk.
21.3.5
Phosphorus
The IOM estimated dietary reference intakes for phosphorus in 1997 . The RDA for phosphorus varies by life stage and sex and is shown in Table 21.5 .
Age (years) | Male (mg) | Female (mg) | Pregnancy (mg) | Lactation (mg) |
---|---|---|---|---|
1–3 | 460 | 460 | ||
4–8 | 500 | 500 | ||
9–13 | 1250 | 1250 | ||
14–18 | 1250 | 1250 | 1250 | 1250 |
19–30 | 700 | 700 | 700 | 700 |
31–50 | 700 | 700 | 700 | 700 |
51+ | 700 | 700 |
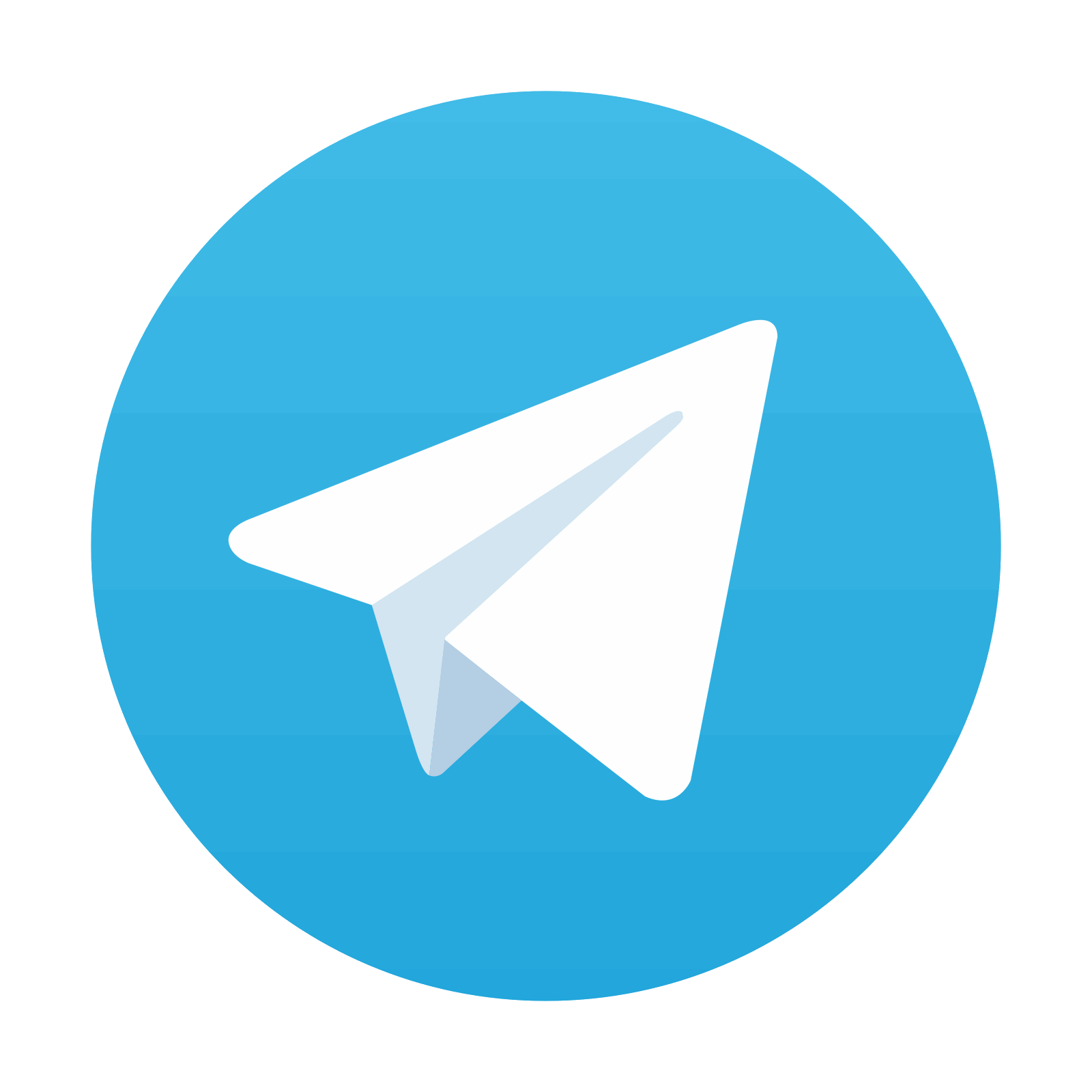
Stay updated, free articles. Join our Telegram channel

Full access? Get Clinical Tree
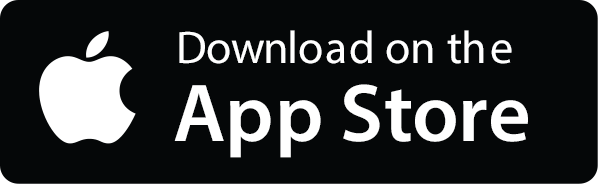
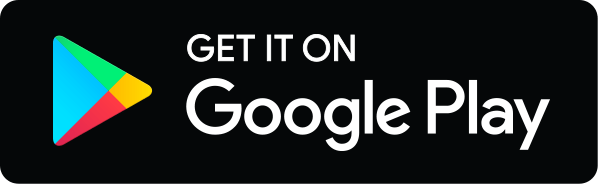
