27.1
Physical characteristics
27.1.1
Gender
For most types of osteoporosis-related fractures, women are at greater risk than men . In a worldwide study of fractures in 2000, there were 9.0 million new osteoporosis-related fractures in women and men combined. Of these fractures 39% occurred in men; this includes 30% of hip fractures, 20% of forearm fractures, 42% of clinical vertebral fractures, and 25% of humerus fractures. Therefore, in general, the percent of fractures at each skeletal site occurring in women is two- to fourfold greater. At age 50 the remaining lifetime risk of osteoporosis-related fractures is 20%–25% in Caucasian men, whereas approximately 50% of women over age 50 will break a bone because of osteoporosis . Many fracture types increase with age in both sexes including the following sites: femur, vertebrae, radius/ulna, humerus, clavicle, scapula, ribs, and pelvis . Morbidity and mortality following a hip fracture have been reported to be greater in men as compared to women . In women and men, mortality was shown to be increased in the year following all major fractures. The excess mortality in men at 1 year post hip fracture was 18% and only 8% in women who fracture at the same age .
Sex-related differences in osteoporosis-related fracture rates have often been attributed to higher bone mineral density (BMD) in men than women. According to NHANES data, 10% of the population had osteoporosis and 44% had low bone mass at either the spine or femoral neck, and these numbers differed by gender. Osteoporosis was found in 15% of women and 4% of men and low bone mass in 51% of females and 35% of men . In MrOS the proportion of men identified as having osteoporosis at baseline was 2% using the World Health Organization reference female-specific T-score and 7% using the US male specific T-score . According to the International Society for Clinical Densitometry 2015 Position statement, the reference database to use to determine T-scores for men of all ethnic groups should be a uniform Caucasian (nonrace adjusted) female reference database .
Osteoporosis is less common in men; however, the secondary causes for osteoporosis are more common in men (50%) than in women (20%–30%) with osteoporosis . The risk factors for male secondary osteoporosis include rheumatoid arthritis, alcoholism, excessive smoking, and gonadal deficiencies.
Although differences in bone density may partially explain gender differences in fracture rates; bone size and geometry may also differ and contribute to differences in both bone density and fracture rates. Differences in bone size and geometry in men and women appear at puberty . Men have a greater increase in periosteal apposition, bone diameter, and cortical thickness during growth. Women, unlike men, have inhibited periosteal apposition and will only increase cortical thickness through endosteal bone formation; these combined effects result in a smaller endosteal perimeter and smaller cortical thickness at puberty. A modest change in the amount of bone formed during growth will have a greater impact later in life on the periosteal apposition rate, a process thought to represent an adaptive response to maintain whole-bone strength during aging. The size of bone has been shown to result in as much as an eightfold variation in periosteal apposition rate later in life. This periosteal expansion to maintain bone strength during aging was also shown to be attributed to variation in adult bone width. Therefore variability in adult bone morphology may be an important factor in understanding skeletal fragility, and perhaps gender differences . Although periosteal apposition may occur after menopause in females, it may not be able to make up for the simultaneous resorption of the endocortical surface .
In a cross-sectional analysis to examine sex-related differences in young men and women, high-resolution peripheral quantitative computed tomography (HRpQCT) was used to measure bone size, geometry, and microarchitecture at the distal radius and tibia along with micro-finite element modeling to estimate bone strength. In this study, predictors of sex-related differences in bone strength of the radius (estimated by ultimate failure load) were due to greater cortical perimeter, trabecular area, and trabecular density in young men as compared to young women. There was no difference in cortical thickness of the radius . In a large ( n =499) HRpQCT cross-sectional study, young men had a larger bone size with larger but more porous cortices and a stronger trabecular network at the radius and tibia as compared to young women, resulting in stronger bones in males . In a cross-sectional study of 644 individuals, young men had larger bone size and larger number of thicker trabeculae and thicker cortices at both the radius and tibia as compared to young women. At older ages, there was less periosteal expansion and more porous cortices in women as compared to men. Differences in failure load suggest that women are less able to withstand strain in the distal radius, perhaps resulting in the sex differences in forearm fracture risk .
Older women have greater decreases in trabecular number as compared to older men where there is trabecular loss is primarily due to thinning. In addition, there are greater disruptions in cortical and trabecular architecture in the radius of females as compared to males . Cortical porosity was also noted to be much greater at both the radius and tibia in older women as compared to older men. These changes in both trabecular and cortical bone lead to greater bone strength in men as compared to women . In individuals over age 50, women had a 96% and men a 56% increase in cortical porosity compared to younger subjects matched for aBMD . Cortical porosity could play a role in sex-related differences in fracture rates. In a recent review, it was stated that the reported age-related increase in cortical porosity might be used to characterize patients at the increased risk of fragility fractures . In a case control study the presence of cortical porosity has been shown to be a risk factor (fourfold increase in risk) for forearm fractures in women with low bone mass . However, there are limited longitudinal data.
HRpQCT of the distal radius and tibia measurements were made at baseline and after 3 years in 260 individuals aged 21–82 years to evaluate differences over time . In young men and premenopausal women, a small increase in cortical thickness seemed to counteract any increase in cortical porosity. With age, women lost trabecula, had an increase in cortical porosity and a decrease in cortical thickness at the radius, as well as an increase in cortical porosity of the tibia resulting in a loss in estimated bone strength. Males had a lesser increase in cortical porosity as compared to females. These data confirm cross-sectional findings and lend further support to the importance of cortical porosity in sex-related differences in fracture rates. Further longitudinal studies will help to explain and confirm these differences between men and women.
The above HRpQCT findings are in agreement with histomorphometric studies where bone loss in men is related to thinner trabeculae as compared to the loss of the entire trabeculae in women. Bone volume/total volume decreases significantly with age in both males ( n =39) and females ( n =41) in iliac crest and vertebral body specimens (obtained during autopsy) using micro-computed tomography and 3D microstructure analysis. In addition, trabecular connectivity, thickness, and number decreased with age while both trabecular separation and structure model index increased with age at both sites. In addition, conversion of plate-like trabeculae into rod-like trabeculae occurred at a faster rate in women versus men leading to a greater bone loss in females . Trabecular 3D bone microstructural differences in lumbar vertebra (L2) showed average trabecular bone volume decreases similarly in men and women as they age, vertical bone was lost faster for women than for men with no sex-related differences in bone loss of horizontal voxels . In a micro-CT evaluation of femoral necks from 28 male and female cadavers, cortical thickness was greater in men than women at any age. The loss of cortical thickness was 10% in men and 15% in women between the middle-aged and elderly groups. From 57 to 98 years of age, porosity increased by 33% in women and 31% in men per decade. Overall, cortical porosity almost doubled and canal diameter increased by about 70% with age (range 57–98 years) in both genders. There was a decrease in canal number with age, indicating cortical porosity is related to intracortical canals that become larger. There are clear gender differences where women had lower cortical thickness and bone volume but higher cortical porosity and canal diameter as compared to men within each age group . It is possible that these differences may contribute to sex differences in fracture rates.
Body size is also thought to contribute to gender-related differences in BMD and fracture rates. In an earlier analysis of NHANES data, it was found that body size had a major influence on the magnitude of sex-related differences in femur BMD and geometry and correction for differences in height and weight removed femur BMD differences but not geometric differences (subperiosteal width, section modulus, and cortical thickness) between young adult men and women. However, in older adults from NHANES, the sex-related differences in both BMD and bone geometry persisted even after statistical correction for body size. In a small study, differences in skeletal size and BMD persisted at most skeletal sites even after matching males and females for body size. These differences appear to relate to differences in the width of bone, but not length of the long bones or height of the vertebrae as expected in a height-matched population . Similarly, the total hip BMD was 6% higher and the femoral neck was 5% higher in males than females. In addition, males had a longer neck axis length and wider neck diameter .
In a long bone, such as the tibia, the cortical shell is thicker in males as evidenced by a larger periosteal circumference and smaller endosteal circumference, even after matching men and women for height and weight Tibial bone density and bone area were also reported to be larger in males age 20–39 compared to females in an Italian. Study of 1205 people age 20–102 . These differences in bone size and cortical thickness are of clinical importance, since the thickness of the cortex and total bone width are the primary determinants of long bone strength. Several studies of hip fracture patients found that nonfractured controls had greater cortical thickness than the fracture patients . A thicker cortical shell might also help explain the lower rates of stress fracture seen in male versus female military cadets .
Body composition differences between males and females have also been explored in relation to bone density and fractures. In the skeleton, sex-related differences are already present at 6 years of age with boys having stronger bones (based on density and geometry) than girls, and these differences appear to be affected by body composition, specifically muscle mass . It is possible that the skeletal differences may result from differences between men and women in the adaptation of bone to mechanical loading . It is also possible that the sex-related differences in bone size are related, in part, to different types of physical activity, dietary forces, or other factors acting on both muscle mass and bone geometry in young adults. The gender differences in BMC, BMD, and cortical thickness may relate to the greater influence of muscle mass and mechanical stress placed on different skeletal sites in men as compared to women . It has been shown that mechanical loading determines cortical, periosteal, and endosteal diameters and the resulting cortical thickness in various animal models . Physical activity has been shown to increase bone circumference at the hip, tibia, and forearm and the type of force as well as other variables may determine the influence of exercise on cortical structure, including whether there is a periosteal increase or endosteal circumference decease . One study found that physical activity increased bone circumference, and when combined with higher calcium intake, there was an increase in cortical thickness, possibly because of less endosteal expansion . Therefore gender-related differences in bone size may be related, in part, to different types of physical activity, dietary forces, or muscle mass acting on bone geometry.
27.1.2
Summary
Males have greater bone density; larger bone size and lower fracture rates than females, and these differences are only partially explained by gender differences in body size between men and women. With age, there is loss in both trabecular and cortical bone and an increase in cortical porosity by HRpQCT and biopsy that is greater in women. Skeletal microarchitecture highlights that there are gender differences during growth and aging that may relate to gender differences in fracture risk.
27.2
Anthropometric variables
27.2.1
Birth weight
The foundation of bone strength likely begins in utero , and several studies have shown a positive relationship between birth weight and bone mass in children and adults , which may lend support to the hypothesis of intrauterine programming. A metaanalysis of nine studies that assessed the relationship between birth weight and BMC, demonstrated that a 1 kg increase in birth weight was associated with a 1.49 g increase in lumbar spine BMC [95% confidence interval (CI) 0.77–2.21] and a 1.41 g increase in hip BMC (95% CI 0.91–1.91) . However, data regarding the associations between birth weight and bone strength parameters in adolescence/young adulthood have been inconsistent .
Small for gestational age and very low birth weight individuals displayed lower peak bone mass and higher frequency of osteopenia/osteoporosis, with the greatest bone deficit in adults born with very low birth weight . In a cohort of 1061 young adult Swedish women, low birth weight was associated with lower BMC in adults at all measured sites, although BMD was largely unaffected. This was also most evident in those with the lowest birth weight . The estimated magnitude of effect was equivalent to a 0.3–0.5 SD difference in BMC for every 1 kg difference in birth weight [151 g (TB); 0.22 g (FN); 1.5 g (TH), 2.5 kg TB lean mass] .
Similarly, a positive association between high birth weight and BMC in adolescence was noticed . However, height growth and weight gain in childhood were more strongly associated with bone accrual at 15–20 years of age. The authors concluded that birth weight has an effect on adolescent bone mass but less than later growth and BMI in childhood and at adolescence .
27.2.2
Height
Women who sustain hip fractures have been reported to be taller than those who do not , whereas there was no effect of height on male hip fracture risk . Maternal height is also a risk factor such that male and female offspring of taller mothers have an increased hip fracture risk , although the reason for this association is not known. Higher growth in height in girls was shown to be important for obtaining a larger skeleton and consequently higher bone mass. However, when predicting bone mineral mass among elderly women, information on early growth does not improve prediction beyond that predicted by current height and weight . In the Northern Finland Birth Cohort 1966 ( n =1087) anthropometric measurements from the ages of 31 and 46 were used to show that height and lean body mass correlated most strongly with vertebral cross-sectional area among both sexes (0.469≤ r ≤ 0.514) . Presumably this would translate into a reduction in risk of vertebral fractures, although this has not been shown.
27.2.3
Weight
It is known that a low body weight (<127 lb) will increase the risk of fracture in older adults by 2–2.4 fold and maintenance of body weight in elderly men and women can prevent bone loss . Bone density is lower in lean than in obese women , although at some skeletal sites such as the spine, osteoarthritis may explain some of the reported differences . Lean women also lose bone at a faster rate than obese women , perhaps a result of the reported increased bone turnover in lean subjects . Several studies have shown that people with a higher body weight have higher bone mass . In cross-sectional studies a variety of measures of body size are positively correlated with BMD in postmenopausal women . In one study, BMD and geometric parameters measured from DXA and QCT showed significant associations with body weight in 84 elderly women . The differences in BMD based on weight appear to begin early, a systematic review and metaanalysis of 27 observational studies evaluated the differences in BMD between normal-weight children and overweight and obese children. In a total of 5,958 children, overweight (MD 213 g; 95% CI 166, 261) and obese children [MD 329 g; 95% CI (229, 430)] have a significantly higher whole body BMC than normal-weight children .
Three large epidemiologic studies (Women’s Health Initiative and Cardiovascular Health Study and EPIDemiologie de l’OStéoporose cohort) were used to determine whether weight or body mass index (BMI) was the best predictor of bone density. Weight alone was found to be a much better predictor of BMD for all skeletal sites than BMI in these three studies .
Not all skeletal parameters demonstrate appropriate adaptation to higher body weight. Cortical porosity and plate BV/TV at the radius and tibia, and cortical vBMD and trabecular thickness at the tibia are less likely to change with higher body weight. These effects may contribute to the higher risk for fracture reported in women with obesity versus controls . Other potential contributors to higher fracture rates in obese individuals are nonbone factors such as higher falling risk, greater force with landing in a fall due to higher body weight, as well as more awkward falling putting the limbs at particular risk .
27.2.4
Body mass index
Although no ideal weight-to-height ratio has been set for reducing osteoporosis and fracture risk, a higher BMI appears to confer protection. A BMI >26–28 kg/m 2 offers protection against fracture, whereas a slender figure of <22–24 kg/m 2 increases fracture risk . A BMI of about 30 kg/m 2 is associated with a 4%–8% greater spine BMD, 8%–9% greater hip BMD, and 25% greater radial BMD compared to a BMI of 20 kg/m 2 ; furthermore, a BMI of 30 kg/m 2 is associated with one-half the loss in spine BMD in early postmenopausal years than a BMI of 20 kg/m 2 . Both the Framingham cohort and the Rancho Bernardo population showed that body weight and BMI explained a substantial proportion of the variance in BMD at various skeletal sites, and this relationship was stronger at weight-bearing sites .
Low BMI is a consistent risk factor for future fracture although the strength of this association is unclear. A metaanalysis of almost 60,000 women and men from 12 cohorts was used to quantify this relationship, and it was found that the contribution of BMI to fracture risk was more marked at low values of BMI such that BMI of 20 versus 25 kg/m 2 was associated with a nearly twofold increase in risk [relative risk (RR)=1.95, 95% CI 1.71, 2.22] as compared to a 17% reduction in risk when BMI of 30 was compared to BMI of 25 . In this metaanalysis the BMI and fracture risk relationship was independent of age and gender; however, the relationship is, at least in part, dependent on BMD . The steepest gradient of increasing risk for fracture occurs at BMI levels below 20 kg/m 2 .
In another metaanalysis, using prospective cohort data from more than 25 countries, baseline data on BMI was available in 398,610 women with an average age of 63 (range, 20–105) years and follow-up of 2.2 million person-years during which 30,280 osteoporotic fractures (6457 hip fractures) occurred. Low BMI (15 vs 25 kg/m 2 ) was a risk factor for hip and all osteoporotic fractures but was protective for lower leg fracture. However, high BMI (35 vs 25 kg/m 2 ) was a risk factor for upper arm (humerus and elbow) fracture. Of note, the association between BMI and fracture risk was modified by the interaction between BMI and BMD . In fact after adjustment for BMD the effect of BMI on fractures, other than hip, is minimized.
Childhood changes in BMI appear to be related to the incidence of hip fracture in 6370 women born in Helsinki between 1934 and 1944. Women in the lowest quarter of adiposity gain had an 8.2-fold increase in hip fracture risk compared with those in the highest quarter ( P <.001) . In a cohort of Chinese with BMI followed from childhood until age 29–37 years of age, the impact of body weight on adult bones originates from childhood but is mediated by concurrent BMI and body composition .
27.2.5
Body composition
Data support the importance of both lean and fat mass as being prime determinants of bone mass. Lean mass may be important for bone mass as a reflection of physical activity levels , whereas fat mass may reflect a source of estrogen . Low muscle mass is a risk factor for low BMD in young adult women; however, high fat appears to be protective only when there is also high muscle mass . It appears that the influence of fat mass on bone mass is greater in older individuals, particularly women . This is further illustrated in a study of about 500 Japanese women where lean body mass was the principal BMD determinant in women aged less than 60 years, while body fat mass and percentage body fat were the principal BMD determinants in women aged 60–69 years . In a review, body composition was noted to “influence bone directly via mechanical stimuli and adipokine secretion and more obliquely through the communication with and modulation of various central and peripheral pathways” . There is growing evidence that fat influences skeletal health and the two main adipokines leptin and adiponectin exert both direct and indirect effects on bone mass .
In a metaanalysis of 16 studies that included 2587 participants, absolute adiposity correlated positively, and relative adiposity negatively, with BMD. Sex and age modified these relationships. The conclusion was that controlled reduction of adipose mass with concomitant preservation of lean mass would be recommended during weight loss .
In older adults, body composition appears to differentially affect the calculated risk of hip fracture in men and women. A two-level biomechanical model was used to assess the association between hip fracture risk and BMD and body composition in 50 male and 80 female hip fracture patients . In this study, impact force was not strong enough in either men or women to consider it as a sole risk factor for hip fracture. While increased BMI and trochanteric soft tissue appeared to offer protection against hip fracture in women, this was not true for men. This may indicate that body composition as a risk factor for fracture should be considered independently for men and women.
27.2.6
Obesity
There has been a dramatic increase in obesity in recent years, and this has led to an increase in various comorbid conditions including Type 2 diabetes, hypertension, and hyperlipidemia. The high rates of obesity are thought to result from numerous lifestyle factors .
When postmenopausal women are stratified by those 115% or above their ideal body weight (IBW) and those below 115%, heavier women had significantly greater BMD at the spine, hip, and radius than normal weight women . In another study in which obesity was defined as a body weight more than 10% above normal weight , obese postmenopausal women had significantly higher vertebral BMD than nonobese postmenopausal women, whereas no significant differences in BMD were observed among obese and normal weight perimenopausal women. This could indicate that obesity helps attenuate postmenopausal bone loss rather than leading to higher peak BMD. The mechanism for the higher bone density in obese women is believed to be increased mechanical loading on the skeleton and/or the greater fat mass leading to greater conversion of adrenal androgens to estrogens in adipose tissue . However, mechanical loading due to muscle contraction is thought to be more anabolic to bone than the loads induced by excess adipose tissue . This raises the possibility that other factors associated with obesity, such as inflammatory cytokines and progenitor marrow stromal cells becoming adipogenic instead of osteogenic (thereby inhibiting osteoblast differentiation) could be offsetting some of the effects of mechanical loading. In fact, higher adiposity in obesity is associated with a lower rate of bone formation Finally there are lower serum levels of 25(OH)D in obesity that appears to result from storage in adipose tissue . It is unclear if this lower level of 25(OH)D in obese women leads to any metabolic consequences.
In a recent metaanalysis, abdominal obesity was significantly associated with a higher risk of hip fracture (combined effect size: 1.30; 95% CI: 1.10, 1.53; P =.007) . This may be a result of abdominal obesity-related inflammation . Visceral fat is related to proinflammatory cytokines such as interleukin-6 (IL-6) and tumor necrosis factor alpha (TNF-α) that can increase bone resorption leading to potentially harmful effects on BMD .
The influence of body weight or degree of obesity on postmenopausal bone loss has been studied. The longitudinal rates of bone loss over 31 months in a population of overweight postmenopausal women (BMI≥25.0, n =40) were compared to those of normal weight women (BMI<25.0, n =115) . The annual rate of vertebral bone loss was reduced significantly among the overweight compared with normal weight women (0.54%–1.1% and 1.46%–1.6%, respectively, P <.05). Harris et al. reported similar results and demonstrated that increased body weight up to 106% of IBW (BMI of 23.6 kg/m 2 ) was protective against postmenopausal vertebral bone loss, although not against bone loss at other skeletal sites. Higher body weight did not appear to provide any further protection from vertebral bone loss. In more lean women, there is an increased annual rate of bone loss and higher rates of bone turnover as compared to heavier women. Serum parathyroid hormone (PTH) levels are higher in obese women , and the mechanism is unclear, though lower serum vitamin D levels could play a role. However, in very heavy and obese women, there is a report of no relationship between body weight and bone turnover .
Recent advances in technology have allowed an evaluation of bone quality that has shown that obese individuals may have lower cortical bone density at peripheral sites than age-matched controls . Severe obesity (BMI>35) was associated with increased trabecular vBMD but a lower cortical vBMD at the tibia in women ages 25–71 years as compared to those of normal weight . In female adolescents, high-compared with the normal-fat group had lower bone measures at the tibia and radius sites (cortical bone area and cortical BMC at the tibia, total bone cross-sectional area at the radius, and strength–strain index at both the tibia and radius; P <.05 for all) . However, in young males, increased fat mass was associated with a smaller radius periosteal circumference, as assessed by peripheral quantitative computed tomography (pQCT) . There are clear relationships between obesity and bone density and bone microarchitecture, although the mechanisms by which this occurs are yet to be elucidated.
27.2.7
Obesity and fractures
Although fracture rates are lower in obese as compared to low weight individuals , the severity of the fracture from falls could be greater in obese individuals . There are also reports of a higher risk of falling in obese as compared to lighter weight individuals , although the cushioning effect of the fat around fracture sites may be protective. The association between obesity and fracture in postmenopausal women was reported to be site dependent, such that obesity is protective against hip and pelvis fractures, yet there is an almost 30% increase in risk for proximal humerus fractures when compared with normal/underweight women and higher rates of ankle fractures as well . Whether this is a result of reduced cortical bone density, higher loads on the limb or another mechanism such as a change in bone quality perhaps related to diabetes is unknown . In addition, the location of the body fat may alter fracture risk such that abdominal obesity was associated with a higher risk of hip fracture in 295,674 individuals (combined effect size: 1.21, 95% CI: 1.15, 1.27, P <.001) .
Three hundred and forty-two men and women aged 62.5±0.5 years from the Newcastle Thousand Families Study birth cohort underwent DXA evaluations of femoral neck and lumbar spine BMD and of the lateral spine for vertebral fracture assessment. Compared to normal weight women, obese women were more likely to have at least one VF (OR=2.65, P =.025, CI 1.13–6.20) and at least one grade 1 vertebral deformity (OR = 4.39, P =.005, CI 1.57–12.28). Obese men were more likely to have a grade 2 and/or grade 3 VF compared to men of normal weight (OR=3.36, P =.032, CI 1.11–10.16). Obesity appears to be a risk factor for prevalent VF, and although absolute BMD is higher in obese individuals, this higher BMD may not be commensurate to their increased body weight .
27.2.8
Weight change and bone mass
Weight gain results in adipocyte hypertrophy, which leads to an increase in obesity-related inflammatory markers such as leptin, TNF-α, and IL-6, while weight loss results in a decrease in these markers . It is also known that adipocytes are not the only source of inflammatory molecules, with macrophages and muscle also secreting these molecules . It is possible that dietary-induced caloric restriction through its effect in reducing inflammation may preserve bone quality despite the reduction in BMD .
In overweight or obese individuals, weight reduction of approximately 10% is typically recommended for reduction of comorbid conditions . Individuals who lose weight involuntarily will have bone loss and weight loss has been associated with an increase in fracture risk in elderly men and women . When individuals lose weight voluntarily, there is an estimated bone loss of 1%–2% at all skeletal sites associated with a 10% weight reduction . The amount of bone loss may be greater in those with a low initial body weight , and young women compared to postmenopausal women may be less likely to experience bone loss associated with weight reduction . In an intervention study in middle-aged men, moderate weight reduction (7%) resulted in 1% bone loss . In the MrOS study of elderly men, weight loss also predicted bone loss . In a study of Norwegian women, weight loss was an independent negative predictor of forearm BMD . Studies have suggested that individuals who are voluntarily losing weight could minimize skeletal bone loss with exercise or additional calcium intake . In a recent 1-year-long, randomized, controlled study in 58 women, cortical thickness was found to decline with an average of 3 lb of weight loss but was prevented with higher vitamin D supplementation .
Changes in total and regional BMD were assessed in male and female obese patients aged 19–70 undergoing rapid weight loss on a low-calorie diet for 2 weeks and then a continued low-calorie diet . After 15 weeks, whole body BMC was reduced significantly (−5.9%), with the largest declines in the trunk (−6.9%) and the smallest in the arms (−4.0%), suggesting greater BMC losses in weight bearing than in nonweight bearing sites. Of note, losses in whole body BMC were correlated positively and strongly with fat mass losses ( r =0.86, P <.01) after 15 weeks, but not with changes in lean mass ( r =0.20, P =.15). In a study of overweight middle-aged men a weight reduction of 7 kg was related to a 1.5% total body bone loss that related to fat loss . Compston et al. reported bone loss in obese postmenopausal women participating in a low calorie dietary intervention, except at the radius . In this study, after 10 months of follow-up, subjects returned to their baseline weight as well as baseline BMD values. Given the fact that body weight and BMD changed in the same direction during weight loss and gain suggests that observed changes in BMD may indeed be real. In contrast, Avenell et al. did not observe parallel gains in weight and BMD in the second 6 months of a 1-year dietary study. These studies raise important questions about whether bone loss that occurs with weight loss will be regained with weight regain.
It is possible that weight change or weight fluctuation in adulthood may be an important determinant of BMD. Older data from the Framingham cohort (693 women) reported that the change in body weight from the biennial examination (1948–51) to the exam in 1988–89 was the strongest explanatory factor for BMD in 1988–89 at the spine, the femoral neck, and radius (10.7%, 8.2%, and 6.5%, respectively, of the total variance, P <.01 for all) . Of interest the relationship between weight change and BMD was strongest in those women not using estrogen.
The Women’s Healthy Lifestyle Project (WHLP) initiated a lifestyle intervention aimed at lowering dietary fat intake and increasing physical activity to produce modest weight loss or prevent weight gain and examined the effect on BMD in premenopausal women. Of the 236 women enrolled the intervention group experienced a mean weight loss of 3.2±4.7 kg over the 18-month study period ( n =115) compared to a weight gain of 0.42±3.6 kg in the controls ( n =111). The annualized rate of hip BMD loss was twofold higher in the intervention group (0.81±1.3%/year) than in the control group (0.42±1.1%/year); a similar although nonsignificant pattern was observed at the spine (0.70±1.4 vs 0.37±1.5%/year). Evidence from this study suggests that the overall risks and benefits of weight reduction among premenopausal women need to include effects on BMD. One would hypothesize that postmenopausal women would be at an even greater risk of osteoporosis given the potential additive effect of weight loss—induced bone loss and normal postmenopausal bone loss. Studies are needed to determine the longer term repercussions of weight loss–induced bone loss.
There is limited evidence to examine whether similar changes in BMD occur when exercise is included as a component of the intervention. In the WHLP study population, large increases in physical activity during the study attenuated spinal bone loss but had no impact on hip bone loss . However, Svendsen et al. compared an energy-restrictive dietary intervention with and without aerobic exercise on weight loss-induced changes in BMD and saw no significant difference in bone loss between overweight women in these two intervention groups. The age of the population may impact the results and a review of the evidence confirmed that weight loss of about 10% is achievable through caloric restriction and exercise in sedentary, frail, obese adults aged 65 years and older. However, there was loss of BMD and lean body mass, which can be attenuated, but not stopped, by the addition of exercise during the active weight loss period . Further studies considering the type, duration, and intensity of physical activity that confers protection against BMD during weight loss are needed.
There are numerous reasons why weight changes result in changes in BMD. The first is that loss of fat tissue that accompanies weight loss may lead to a marked reduction in endogenous estrogen production. It is also possible that weight loss leads to a decline in the mechanical stress on the skeleton and this may influence bone remodeling. There may also be a decrease in lean mass, resulting in weakened muscle strength and increased frailty. Weight loss has been associated with nonsignificant reductions in markers of bone formation and elevations in marker of bone resorption among women consuming an energy-restrictive diet compared to controls. Similar results were reported by Salamone et al. in which premenopausal women who lost the most weight (loss>8%) experienced the largest increase in the percentage change in N-telopeptide, a marker of bone resorption.
Data regarding weight loss and fracture risk are growing. Weight loss ≥10% reduced risk of hip fracture among Caucasian men (HR 2.79; 95% CI 1.62, 4.79) and women (HR 2.9; 95% CI 2.0, 4.1) in the United States. In a cohort of 63,257 Chinese in Singapore aged 45–74 at baseline, compared to stable weight, weight loss ≥10% over an average of 6 years was associated with nearly 40% increase in risk of hip fracture . A systematic review and metaanalysis of 15 studies of bariatric surgery and lifestyle weight management programs (WMPs) with fracture outcomes indicated no association between bariatric surgery and any fracture (365 participants; RR 0.82; 95% CI 0.29–2.35). Although, four out of six observational studies of bariatric surgery demonstrated significantly increased fracture risk, 6 RCTs of WMPs with 6214 participants, the longest follow-up 11.3 years, showed no clear effect on any type of fracture (RR 1.04; 95% CI 0.91–1.18). However, authors of the largest RCT reported an increased risk of frailty fracture by their definition (RR 1.40; 95% CI 1.04–1.90) .
27.2.9
Summary
Clearly body weight and BMI are positively related to BMD and in general inversely related to fracture rates. Lean mass may be a more important determinant of bone mass in premenopausal women, but fat mass appears to relate more strongly to BMD in postmenopausal women.
Obese individuals need to lose weight to reduce the risk of comorbid conditions. However, clinicians need to be aware that weight loss can be detrimental to the skeleton. Further studies are needed to determine the role of calcium, vitamin D, and exercise in minimizing bone loss that is associated with weight loss. The mechanisms whereby obesity impacts bone density and quality are uncertain.
27.3
Lifestyle factors
27.3.1
Smoking and the skeleton
There are numerous proposed mechanisms for the relationship between smoking and lower BMD. In animal studies the formation of new bone is impaired by exposure to nicotine . One report indicated that cortisol is briefly raised after smoking , although cortisol differences have not been observed in epidemiologic studies . Lower dietary calcium intake and lower calcium absorption has been reported in smokers versus nonsmokers . Although estrogen levels were thought to be a potential mechanism for lower BMD in smokers; this has been countered by the lack of an effect of smoking on bone density in premenopausal women and studies that indicate that postmenopausal women who smoke have higher rates of bone loss, yet similar plasma levels of estrogen as compared to nonsmokers . Women smokers on oral hormone therapy have lower estrogen levels because of increased hepatic metabolism and smokers have a slightly earlier menopause , but neither is expected to result in the significant difference in BMD seen between smokers and nonsmokers. Lower body weight is often reported in smokers versus nonsmokers and could be a result of lower energy intake or increased energy expenditure . Smoking has also been related to lower serum 25(OH)D levels in premenopausal women and men , perhaps related to liver enzyme activity. Lower serum osteocalcin has also been reported in cohorts of premenopausal and postmenopausal women . Smoking also adversely affects other hormones and enzymes involved in bone regulation such as parathyroid hormone and alkaline phosphatase .
There also appear to be skeletal microarchitectural changes in adult male smokers. It was shown that older men who are moderate current smokers had poor trabecular (but not cortical) microarchitecture, a difference not apparent in areal BMD. These differences in bone quality due to smoking appear to begin at a young age .
The potential mechanisms whereby smoking affects the skeleton include an impact on calcium regulation, alterations in sex-hormone production and metabolism, changes in adrenal cortical hormone metabolism and in the RANK–RANKL–OPG system), as well as direct cellular effects of cigarette use on bone cells .
27.3.2
Smoking and bone mineral density
A large metaanalysis of 10 epidemiologic studies found that smoking ( n =614 smokers) had no major effect on premenopausal bone density , a finding also noted in other studies . Another analysis from the Danish Osteoporosis Prevention Study ( n =2015 women and n =832 smokers) found the BMD difference between current smokers and never-smokers was significant and equal to 1.6% at the spine, 2.9% at the femoral neck, and 1.9% for the total body. The authors also reported a statistical interaction between smoking and fat mass such that women having the greatest fat mass had BMD that was unaffected by smoking . In addition, they reported that serum levels of vitamin D and osteocalcin were both inversely related to number of cigarettes smoked per day. The cumulated effect of smoking on BMD is small and may account for the failure to find an association between smoking and BMD in premenopausal women in previous studies .
The relationship between smoking and BMD in postmenopausal women was evaluated in several metaanalyses and even a dose dependent relationship between number of cigarettes smoked and decrease in BMD has been reported . In one metaanalysis based on 21 cross-sectional studies , lower postmenopausal BMD was noted in current smokers than in nonsmokers with a BMD decline of about 2% for every 10 year increase in age, resulting in a 6% difference at age 80. In another metaanalysis, there were independent negative effects of smoking on bone mass at the spine, hip, and forearm. The hip was most affected with BMD measured in smokers as 0.3 SD below that of nonsmokers . Furthermore, both current smoking and ever smoking were related to greater rates of bone loss in 13 prospective studies, even after controlling for age and body weight .
In a study of 1044 women 75 years of age or older from the Malmo Osteoporosis Prospective Risk Assessment (OPRA), BMD of the hip and total body were significantly lower (up to 10%) in current smokers as compared to nonsmokers after controlling for weight and levels of physical activity . A return toward the greater bone mass for never smokers was demonstrated in prior smokers and the magnitude was dependent on the length of time as a nonsmoker. In a metaanalysis the negative impact of current versus never smoking was greater than in former versus never smokers .
Several studies that have examined the relationship between smoking and BMD in men have found a stronger association than that reported in women . In a metaanalysis of 29 studies, postmenopausal bone loss was greater in current smokers than nonsmokers, with 6% difference in BMD being observed by the age of 80 years old. In that study, bone mass in smoking men was about 0.3 SD lower than that of nonsmokers . In the metaanalysis by Ward and Klesges, smoking was shown to have a more deleterious effect on bone mass in men than for women. Smoking effect on BMD was actually 50%–300% greater in men as compared to women at all combined skeletal sites, including spine and forearm . These differences in BMD are apparent in young adult men (18–20 years) followed for 5 years. The men who had started to smoke at a young age had about half as much increase in total body BMD (46.5%) and lumbar spine (51.9%), and about twice as much decrease in BMD at the total hip and femoral neck, as nonsmokers . In a more recent study, greater tobacco intakes were associated with lower femoral neck BMD in 1683 women and 1010 men over the age of 50 years who were followed up for up to 20 years in Australia . In this study the impact of smoking on spine BMD was greater in men, whereas the impact on femoral neck BMD was more similar and slightly greater in women. Clearly, smoking has a negative effect on BMD, perhaps more so in men than women.
27.3.3
Smoking and fracture risk
The risk of falling in elderly people is higher in smokers than in nonsmokers . Smoking is a major cause of hip fracture and increases the lifetime risk from about 12%–19% in women up to age 85 and from 22% to 37% to the age of 90 .
The effect of smoking on hip fracture is most evident among thin, elderly women. In female smokers (age >50 years), the excess risk of hip fracture was 50%, and among the thin female smokers (BMI <20 kg/m 2 ), the risk increased threefold both when compared to nonsmokers . In this study, thin male smokers also had an 80% increased risk as compared to male nonsmokers.
In another study the analysis was extended to men and to former smokers. Fifty-one studies including 512,000 people were analyzed. Current smoking was associated with higher risk of any fracture, and with hip and spine fractures, but not wrist fractures. Previous smoking was only associated with increased risk of hip fracture. Studies reporting on the amount smoked reported higher risk estimates based on number of cigarettes smoked. Former smokers had an increased risk for proximal humerus fracture (HR 2.07; 1.19–3.57) and current smokers had an increased risk for osteoporotic (HR 1.47; 1.05–2.05) and vertebral fractures (HR 2.50; 1.58–3.95) compared to nonsmokers. Former smokers had a decreased risk for vertebral fractures, but not for other types of fractures, compared to current smokers .
In women the cumulative risk of hip fracture is 7% and 15% higher in current smokers than nonsmokers aged 85 and 90 years, respectively .
In another study the risk of hip fracture was 1.6-fold higher in men with history of smoking than those without, and cessation of smoking reduced the risk of hip fracture by 27% .
In one of the largest evaluations the authors aimed to quantify the risk of smoking on fracture in an international population. They further explored the relationship of this risk with age, sex and BMD. The sample included 59,232 men and women (74% female) from 10 prospective cohorts followed for a total of 250,000 person-years. Current smoking was associated with a significantly higher risk of any fracture compared to nonsmokers (RR=1.25; 95% CI=1.15–1.36), with only a moderate reduction in the RR after controlling for BMD. The highest risk was observed for hip fracture (RR=1.84; 95% CI=1.52–2.22), but this was also somewhat lower after adjustment for BMD. Low BMD accounted for only 23% of the smoking-related risk of hip fracture. A prior smoking history was associated with a significantly increased risk of fracture compared with individuals with no smoking history, but the risk ratios were lower than for current smoking .
In a more recent metaanalysis, which pooled results from 10 prospective cohort studies, current female smokers had a RR for hip fracture (pooled RR, 1.30; 95% CI, 1.16–1.45), which was only significant among smokers using more than 15 cigarettes/day. Smoking cessation for ≥10 years leads to a significant decline in hip fracture risk . Similar results were found in men where a metaanalysis of 12 prospective studies showed that the RR for hip fracture in current male smoking was 1.47 (1.28–1.66). Eight studies reported the RRs for former smokers compared with never smokers and the pooled RR was 1.15 (0.97–1.34) .
Evidence regarding the relationship between cigarette smoking and wrist fractures is limited. Among thin women who had never taken postmenopausal estrogen, the risk of forearm fracture was found to be more than fivefold greater (RR, 5.4; 95% CI, 2.5–11.3) among smokers than among never-smokers Several other studies have failed to show a relationship between smoking and wrist fractures , including a metaanalysis .
Studies in female twins established that smokers have lower spinal BMD by the time they reach menopause than do nonsmokers . It also appears that by the age of 60 years, the risk for vertebral fracture is almost double among both men and women who smoke . In the study of Osteoporotic Fractures, current smoking was found to increase the risk of a first vertebral fracture . Similarly in the Leisure World Cohort and in CANDOO, smoking was related to vertebral fractures . In a metaanalysis, smokers had an elevated RR as compared to nonsmokers for spine fractures (RR=1.76; 95% CI=1.10, 2.76) .
27.3.4
Smoking and fracture healing
Smokers are not only more susceptible to fracture but healing of fractures is delayed in smokers, the maturation of the regenerating bone is reported to be abnormal, and nonunion or malunion is more frequent . Smokers also assess their outcomes after surgery much less favorably than do nonsmokers. Hilibrand et al. found that 89% of nonsmokers who had undergone multilevel anterior cervical decompressions with autogenous grafting rated their outcome as good or excellent versus 76% of the smokers. The post fracture complications of smoking have been recently reviewed .
27.3.5
Summary
A clinically important number of fractures may be related to smoking. Reducing current smoking would help prevent many fractures, including hip and spine fractures, and may also improve the healing of fractures. The data on this topic were recently reviewed and the paper provides further detail.
27.3.6
Alcohol
The negative effects of excessive alcohol consumption on bone have long been recognized and the increased risk of fracture may result from lower BMD, microarchitecture deterioration, and other nonskeletal factors, including higher falling risk. One issue is the definition of level of alcohol consumption. Chronic heavy drinking has been identified as a significant risk factor for various diseases, including osteoporosis and associated fractures. Chronic and heavy alcohol consumption is known to contribute to low bone mass, decreased bone formation, increased incidence of fractures, and delays in fracture healing . The onset of bone loss is followed by an elevation in fracture risk about 10–20 years later . The impact of chronic and heavy alcohol consumption is often confounded by many comorbidities that can impact skeletal metabolism. Alcohol is associated with a suppression of new bone formation and modest changes in bone resorption that lead to bone loss .
Not only is fracture risk elevated but, in addition, individuals with a history of alcohol abuse have significantly higher frequency of complications of fracture healing as compared to nonalcoholic patients . Alcohol might inhibit new bone formation in the fracture site, a function needed for fracture repair .
Bone histomorphometric studies of alcoholic patients have reported that numerous indices of new bone formation are suppressed in alcoholics as compared to control subjects , whereas indices of bone resorption do not appear to differ 247,268 or may be increased . However, any increase in bone resorption may impair bone quality by increasing cortical porosity and decreasing trabecular connectivity. Alternatively, abnormally low bone remodeling can lead to excess micro-fractures and impact the strength of bone. It was also reported that the active bone surface may be reduced in alcoholics and that there would be a discrepancy between total and active remodeling surfaces in alcoholics . Alcohol induced bone loss appears to relate to alterations in bone remodeling, bone formation, and bone resorption . It is also possible that alcohol may also act on bone remodeling by osteocyte apoptosis, oxidative stress, Wnt signaling pathway modulation, and lipid accumulation in bone tissue, particularly in the marrow .
The impact of alcohol on bone formation appears to be reversible, and the osteoblast will quickly recover (within 1–2 weeks) following the period of abstinence from alcohol . Bone formation increased after only 5 days of abstinence in alcoholic women .
27.3.7
Bone density and alcohol
While the toxic effects of alcohol abuse are well established, moderate alcohol consumption may actually have a modest favorable effect on bone density, particularly in postmenopausal women , although not all studies report this . Alcohol may slow down the accelerated bone-remodeling rate that is a characteristic of postmenopausal osteoporosis. There is also evidence to suggest that moderate alcohol intake is beneficial to bone density in premenopausal women although there have also been reports of a negative relationship between BMD and alcohol or no relationship . In men, moderate alcohol intake has been shown to positively relate to BMD or have no association .
An analysis of National Health and Nutrition Examination Survey data examined the cross-sectional association between alcohol intake or binge drinking and BMD in men, postmenopausal and premenopausal women, controlling for height, weight, race, physical activity, smoking, calcium intake, and self-reported health status. In that analysis, hip BMD was higher in men and postmenopausal women who drank compared to abstainers, whereas there was no significant difference in hip BMD between premenopausal women who drank versus those who abstained . In a cross-sectional analysis of 3218 women aged 50–62 years from the Aberdeen Prospective Osteoporosis Screening Study, moderate alcohol intake was positively associated with BMD, independently of lifestyle. In that study, lifestyle was defined by smoking, physical activity, and fruit and vegetable intake . Greater alcohol intakes (number of standard drinks per day) were associated with higher femoral neck BMD in 1683 women and 1010 men over the age of 50 years who were followed up for up to 20 years in Australia . More well-designed longitudinal studies, such as this, but with reproducible measures of alcohol intake, are needed to further explore the age- and gender-related differences in the effects of moderate consumption of alcohol, while controlling for important covariates.
In a study using HRpQCT, moderate-to-heavy alcohol intake had little association with bone geometry, density, and microarchitecture . However, in a histomorphometric study, compared to controls, bone tissue from alcoholic men exhibited a significant decrease of apparent mechanical properties and significant changes in several microarchitectural parameters . The impact of alcohol on the skeleton may also be modified by skeletal site, age, gender and amount, as well as duration of ETOH consumption.
27.3.8
Alcohol, falls, and fractures
Due to the effects of alcohol on balance and gait, alcoholics tend to fall more frequently than the general population. Men with problem drinking had approximately a 60% greater risk of two or more falls than those without a history of problem drinking in the MrOS study . However, the association between moderate alcohol intake and falls is less clear with reports of a positive association , no association , or even a protective association . In a cohort with 2170 older adults followed up for 3.3 years, both moderate drinking and the Mediterranean drinking pattern were associated with a lower risk of falls and injurious falls . The relationship between alcohol and injury and the effects of country-level detrimental drinking pattern (DDP) and alcohol control policy was examined in over 18,000 injured patients in 22 countries. The risk of all injury was higher in higher DDP countries compared to lower DDP countries for ≤3 drinks in relation to falls (OR=2.51, CI=1.52–4.16) .
A metaanalysis indicated that greater intake of alcohol (>2 drinks/day) is associated with greater risk of nonspine fractures , including osteoporotic and hip fractures. In men, lifetime alcohol consumption has been associated with greater risk of vertebral fractures . High intake of alcohol (>28 drinks a week) was related to higher hip fracture risk . However, several other studies have failed to show an association between daily alcohol consumption and risk of hip fracture or vertebral fracture or all fractures (in older men) . One study reported a protective association in men who consume alcohol . As expected, older alcoholics are at substantially greater risk of hip fractures than younger alcoholics . Recent alcohol use, within the 24 hours prior to the hip fracture, was reported by 21.5% of men and 7% of women . Alcohol concentration in blood was positive in 19% of men and 16% of women with a hip fracture .
Among elderly men and postmenopausal women , moderate alcohol intake has been associated with lower fracture risk but in the Nurses Health Study, even moderate intake, defined as up to one drink a day, is associated with higher risk for bone fractures . However, another study showed no association between moderate alcohol intake and fracture risk . In a review of younger (age 20–60) hip fracture patients, every second to forth patient smoke, have chronic diseases, and abuse alcohol .
27.3.9
Alcohol and fracture healing
Alcohol can suppress synthesis of an ossifiable matrix, which interferes with fracture healing. Alcohol consumption changes the composition of this matrix by suppressing the formation of osteoblasts and/or decreasing their ability to respond to signals that normally trigger bone formation after a fracture . In two large epidemiologic studies, alcoholism was related to nonunion . It is also possible that nutritional deficiencies associated with alcoholism may influence fracture healing. A recent paper noted that arginine–citrulline–nitric oxide metabolism has a major influence on the process of fracture repair, more specifically appropriate concentrations of amino acids and temporal expression of nitric oxide synthase enzymes are important for an adequate bone-healing process .
27.3.10
Alcohol and nutrition
Malnutrition is frequent in alcoholics . Therefore one must consider nutritional status as an important factor to consider in relation to skeletal health. In a study of 181 male alcoholics, variables that related to bone loss included a serum marker of bone formation, grip strength and BMI, a potential marker of nutritional status . Many nutritional deficiencies (magnesium, potassium, phosphorous, and calcium) accompany alcoholism and this could contribute to lower bone mass and higher fracture rates . One nutrient, calcium as well as calcium balance is particularly disrupted by alcohol. Alcohol can also inhibit the production of enzymes found in the liver and kidney that convert the inactive form of vitamin D to its active form. This interference in vitamin D metabolism results in an impairment of calcium absorption. Alcohol also increases magnesium excretion, an effect that can further negatively impact bone health . Low body weight often results which increases risk for low BMD .
27.3.11
Alcohol and hormones
Alcoholic men tend to produce less testosterone and low testosterone levels have been linked to decreased activity of osteoblasts, the cells that stimulate bone formation . However, estrone and estradiol concentrations are elevated in alcoholic men . In premenopausal women, chronic alcohol exposure can result in irregular menstrual cycles, an occurrence that increases osteoporosis risk. Conversely, in postmenopausal women, alcohol increases the conversion of testosterone into estradiol, a hormone commonly used to prevent bone loss after menopause. Several studies have shown that circulating levels of estrogen are positively related to alcohol intake . For this reason, moderate alcohol consumption may actually have a positive effect on bone density in women after menopause.
Alcoholics have been shown to have high levels of cortisol, a corticosteroid. Excessive levels of cortisol have been linked to decreased bone formation and increased bone resorption. Corticosteroids impair calcium absorption, which leads to an increase in parathyroid hormone secretion, which can result in further bone loss. The impact of alcohol on the liver may lead to liver dysfunction that can have a negative impact on vitamin D metabolism and lower levels of 25(OH)D have been found in alcoholics .
27.3.11.1
Alcohol and molecular mechanisms
Ethanol has been shown to promote the differentiation of mesenchymal stromal cells into adipocytes instead of osteoblasts. This may explain the increase in marrow adiposity and decrease in bone mass seen in animal models given a heavy, chronic alcohol in-tube. There are many other potential mechanisms. It is also possible that proinflamatory cytokines and Wnt and MTOR signaling may relate to the mechanism whereby alcohol impacts the skeleton .
27.3.12
Summary
Chronic and heavy alcohol consumption contributes to low bone mass, decreased bone formation, an increased incidence of fractures, and delays in fracture healing. Moderate alcohol consumption may be related to higher BMD although it is difficult to isolate the effect of moderate alcohol intake from other potential confounders. The mechanisms whereby alcohol impacts the skeleton need further exploration.
References
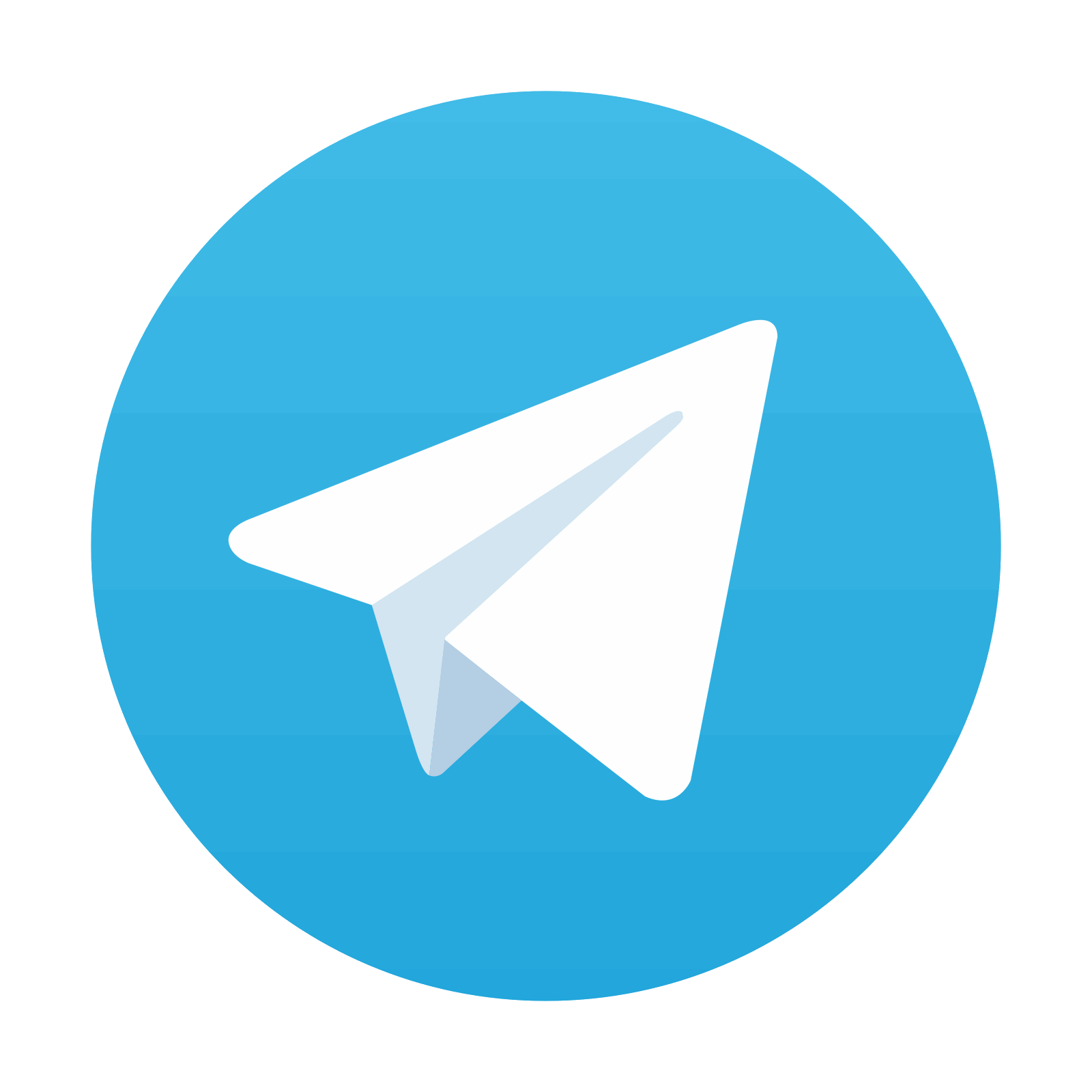
Stay updated, free articles. Join our Telegram channel

Full access? Get Clinical Tree
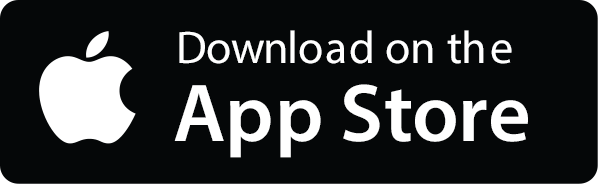
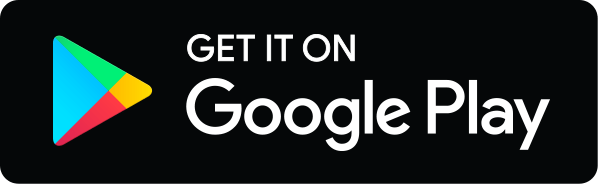
