Fig. 7.1
Images demonstrating various forms of radiation injury affecting breast reconstruction. (a) Acute diffuse radiation injury characterized by erythema, edema, and desquamation of injured skin. (b) Fibrotic soft tissue coverage of underlying tissue expander preventing implant expansion. (c) Hyperpigmented and fibrotic skin with significant capsular contracture distorting the breast prosthesis. (d) Skin hyperpigmentation and contraction in association with poor wound healing and persistent wound after implant removal due to infection
Radiation following breast prosthetic placement is associated with a higher rate of complications, particularly capsular contracture [1]. Poor cosmetic result, expander extrusion, and eventual implant loss are all potential further complications. Patients receiving immediate implant reconstruction and subsequent radiation also have a high rate of reoperation for either correction of defects created by radiation or replacement of the reconstruction by an autologous flap [2]. Autogenous tissue utilized for breast reconstruction undergoes similar changes as native tissue when irradiated. Patients with radiated autologous breast reconstructions frequently require another flap to correct contour irregularities created by radiotherapy [3].
Due to the above, breast reconstruction should be delayed until after any planned radiation, if possible. In contrast, chemotherapy does not seem to increase the complication rate of implant-based or autologous breast reconstruction and may be safely performed on an immediate basis in patients who require adjuvant chemotherapy.
In the era of patient-centered care, a shared decision-making process which takes patient satisfaction into account is critical to improving the quality of breast healthcare. Ultimately, patient satisfaction in breast reconstruction is tied to the symmetry between breasts at the completion of reconstruction and is paramount to the process of technique selection. Patients undergoing unilateral breast reconstruction report higher patient satisfaction when autologous tissue techniques are employed for the unilateral reconstruction; however, bilateral mastectomy patients have equal satisfaction regardless of the technique chosen. This is due to the fact that breast symmetry is excellent when the same technique is used on both breasts [4].
New Techniques
Patient Education
Breast reconstruction options vary greatly and can be quite complex, making this a challenging decision for patients considering reconstruction. Preoperative patient education is one of the strongest predictors of patient satisfaction with breast reconstruction outcomes, and patient dissatisfaction with breast reconstruction information contributes to decision regret [5, 6]. Despite this understanding, approximately 20% of breast cancer survivors report they were never told about reconstruction [7]. Moreover, ethnic minority women appear to be the least informed about reconstruction [8]. Historically, breast reconstruction information was only available verbally and was provided by the oncologic surgeon or the reconstructive surgeon. Today, more information sources are available to mastectomy patients and include verbal, written, and digital/online options. Unfortunately, some of the most commonly used resources regarding breast reconstruction are written at a level that is too difficult for the average patient to understand [9]. In order to utilize patient education as a means to improve overall satisfaction, it is crucial not only to verify the quality and accuracy of resources provided but also to ensure that information is interpreted properly. Other factors affecting discussions regarding breast reconstructions include patient fear and anxiety regarding their diagnosis and oncologic management, cultural or language barriers, and the surgeon-patient relationship itself [5].
Currently, efforts are ongoing to maximize patient education and information quality by creating easy access, easy-to-use pre-consultation digital information regarding breast reconstruction that is then discussed and clarified during the meeting with the reconstructive surgeon. In order to reinforce information gained during the pre-consultation period and surgical consultation, opportunities for patients to speak to those who have completed the reconstruction process are becoming more common.
Patient Selection and Risk Management
Weight Loss and Its Role in Breast Reconstruction
Obesity, defined as a body mass index (BMI ) above 30, is a worldwide epidemic. Selecting obese patients appropriate for breast reconstruction poses a challenge for the reconstructive surgeon due to the fact that this patient population is predisposed to other comorbidities (diabetes, hypertension, coronary artery disease, and lymphedema), as well as an increased risk of postoperative complications including seromas, skin and soft tissue infections, bronchopneumonia, and venous thromboembolism (VTE ) [10]. In addition, operation-specific complications are more likely to occur in the obese patient. Understanding the risks associated with obese patients and each reconstruction option is critical to ensure a safe and appropriate method is chosen.
Implant-based breast reconstruction remains the most common type of breast reconstruction worldwide. When performed in the obese patient, there is a total complication rate ranging from 18 to 30%. The obese patient is twice as likely to suffer implant loss and seven times more likely to have reconstructive failure [11, 12]. Although flap survival rates are similar to autologous reconstruction in the nonobese patient, patients with a BMI >30 are significantly more likely to experience an abdominal donor site complication, including ventral hernias [13]. Examination of the NSQIP registry confirms an increased risk for postoperative morbidity in this patient population regardless of reconstruction technique [14].
Attempts to mitigate operative risk in the obese patient have included weight loss prior to operative intervention; however no clear evidence exists that this actually reduces postoperative complications [15]. Ozturk et al. examined 182 abdominal free flap-based breast reconstructions and reported significantly higher flap and donor site complications in obese patients than those with lower BMIs. Interestingly, the authors did not find preoperative weight loss to significantly reduce these complication rates [16]. Despite these results, obese patients should still be encouraged to lose weight prior to surgery due to the well-known health and psychological benefits of this practice and higher rates of patient satisfaction with respect to surgical outcomes [17].
Venous Thromboembolism (VTE) Risk Assessment
Postoperative venous thromboembolism (VTE ) is a serious yet preventable disorder with the potential to cause short-term mortality and long-term morbidity. Early identification of VTE is critical to its successful management; however clinical signs are notoriously unreliable, which may lead to a delay in diagnosis [18, 19]. The potential for debilitating consequences secondary to VTE has fueled efforts to identify patients who are at high risk of VTE development and the institution of prophylactic measures when appropriate. The Caprini Risk Assessment Model (RAM) and guidelines provided by the American College of Chest Physicians (ACCP ) have predominantly been applied to non-plastic surgical procedures to aid in identification of those patients who may benefit from chemical VTE prophylaxis. Recently, the Venous Thromboembolism Prevention Study (VTEPS) was completed in order to specifically apply the Caprini RAM as a screening tool to identify patients who would benefit from DVT prophylaxis after plastic and reconstructive surgical procedures. The Caprini RAM has been validated by the American Society of Plastic Surgeons (ASPS ) and has been shown to decrease the rate of VTE events without an increased risk of postoperative bleeding complications. Similar to its use in non-plastic surgical procedures, a numerical score is assigned to each patient after review of different patient and operation-specific risk factors and recommends using chemical prophylaxis for “high risk” patients who have a Caprini score ≥7 [20].
Free Tissue Transfer Assessment
Preoperative Evaluation
Perforating vessels arising from the larger named blood vessels supplying the various breast reconstruction soft tissue donor sites have variations within an individual patient and among patients. Knowledge of these anatomic variations preoperatively aids in appropriate perforator selection and improves operative efficiency. Multiple diagnostic techniques including Doppler ultrasonography, computed tomography angiography (CTA), magnetic resonance angiography (MRA), and fluorescent angiography (FA) have been utilized to facilitate preoperative perforator mapping to provide reconstructive surgeons with invaluable information regarding vessel origin, caliber, branching patterns, and magnitude of flow [21, 22].
Doppler/duplex ultrasonography was first used in the 1990s to assist with perforator-based free flaps and is a cheap, widely available imaging modality that does not expose patients to radioactive or nephrotoxic contrast agents [23]. Despite these benefits, its known disadvantages include long study times, operator dependence, and the inability to reliably identify perforator caliber. Computed tomography angiography has supplanted ultrasound as the preferred method of preoperative perforator identification with sensitivities and specificities approaching 100% [24] (Fig. 7.2). Despite the established benefits of preoperative imaging with CTA , it can only provide a static view of the abdominal vasculature in its preoperative state, and it cannot provide information about flow within the perforators. In addition, radiation exposure and the potential to develop a contrast allergy may complicate the performance of this imaging test.
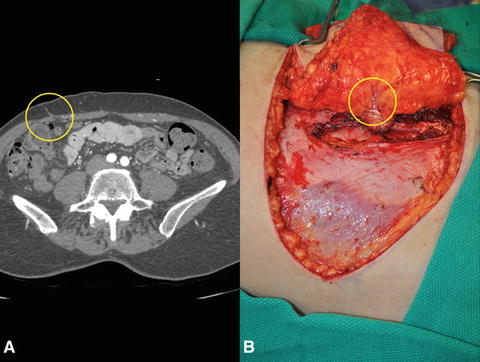
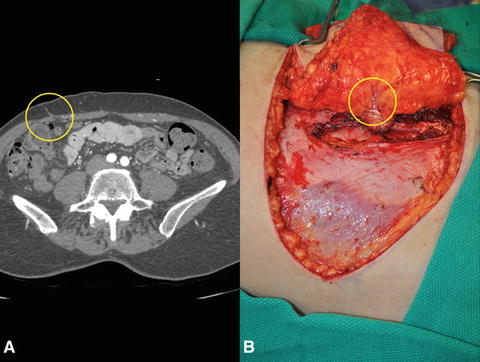
Fig. 7.2
(a) Computed tomography angiogram (CTA ) image demonstrating right hemi-abdomen perforating vessels from the deep inferior epigastric pedicle. (b) Intraoperatively identified perforator correlating to preoperative perforator mapping with CTA
Magnetic resonance angiography has received recent attention as an option for perforator mapping [25]. The safer side effect profile of gadolinium and recent use of higher field strength scanners have improved the accuracy of MRA for perforator characterization [21]. With regard to perforator mapping, MRA has demonstrated high specificity when compared to CTA [26]. Furthermore, the image quality of MRA is generally considered to be inferior to that of CTA; however its muscle-to-vessel contrast ratio is excellent and most accurately delineates perforator intramuscular course. The disadvantages of MRA include its high cost, low availability, susceptibility to motion artifact, and limited capacity to detect perforators with a diameter <0.8 mm [25]. Although gadolinium is less likely to cause an anaphylactic reaction, it may produce nephrogenic systemic fibrosis limiting its use to a specific subset of patients including those with iodine allergies and impaired renal function. Additionally, MRA should be avoided in patients who are morbidly obese, those with pacemakers, and in patients who suffer from anxiety or claustrophobia.
Indocyanine green fluorescent angiography (ICGFA ) is an accepted imaging technique newly applied to plastic and reconstructive surgery [27, 28]. This imaging technique allows direct visualization of macrovascular anastomoses, microvascular anastomoses, and tissue perfusion. The ICG fluorescent dye emits energy upon excitation by a light source (laser or LED light), which is then captured and recorded by a variety of image capture devices available creating real-time videos of blood flow and/or tissue perfusion. This unique feature allows images to be captured before, during, and after a flap is elevated, providing a live and continuous assessment of flap perfusion. Although the benefits of intraoperative fluorescent angiography are established, its role in the preoperative setting is being evaluated. A prospective study examining ICGFA perforator mapping of the abdominal wall in preparation for free tissue transfer breast reconstruction from the abdominal donor site demonstrated that skin blushes identified by ICGFA do not correlate with preoperative CTA or intraoperative perforator characteristics and therefore should not replace other forms of preoperative perforator mapping techniques [22].
Intraoperative Evaluation
Fluorescent Angiography (FA )
A growing body of literature supports intraoperative use of ICGFA. As discussed above, FA may be employed to confirm flow within vascular anastomoses. In addition, correlation between intraoperative FA and postoperative tissue perfusion-related complications has led to its frequent use in soft tissue perfusion assessment. Specific intraoperative uses have been described for both implant-based and autologous tissue breast reconstruction.
A common use of intraoperative ICGFA is determination of the viability of mastectomy skin flaps in the setting of immediate breast reconstruction. This technique has demonstrated high sensitivity and specificity in predicting postoperative mastectomy skin flap necrosis [29]. Similarly, Duggal et al. showed that intraoperative ICGFA was associated with a significant reduction in mastectomy skin flap necrosis and reoperation rate [30].
Perfusion assessment of breast reconstruction flap skin paddle and subcutaneous tissue is another common intraoperative use of ICGFA. Similar to its benefits in predicting skin loss for mastectomy flaps, ICGFA has been demonstrated to aid in intraoperative identification of poorly perfused portions of a flap, guiding soft tissue resection to minimize postoperative tissue loss [22].
Postoperative Evaluation
Implantable Doppler Monitoring
Loss of free tissue transplanted for breast reconstruction is a devastating complication. Intraoperative efforts to ensure flap survival are paramount and monitoring of these fragile procedures postoperatively is of equal importance. The goal of postoperative free flap monitoring is to maximize the potential for tissue salvage by focusing on early detection of microvascular complications before permanent flap injury occurs [31]. Although physical exam remains the gold standard for monitoring flap viability, early changes in a compromised flap can often be subtle. The use of adjunct technology can supplement clinical acumen and improve the accuracy and objectivity of flap monitoring.
The Cook-Swartz implantable Doppler system was first described by Swartz et al. in 1988 and pioneered real-time monitoring of blood flow through a vessel [32]. Continuous assessment of the microvascular anastomosis allows for early recognition of pedicle compromise. The Doppler consists of a 20 MHz ultrasonic Doppler crystal, a silicone cuff, and an external monitoring device. Intraoperatively, the probes may be placed on the vein, artery, or both. Direct pedicle monitoring is unique to this technique as other monitoring devices predominantly gauge flap viability through measurement of perfusion, oxygenation, or ischemia within the flap itself. Additionally, its invasive nature allows for the monitoring of buried flaps which are unexaminable from the body’s surface. The success of this monitoring technique is measured by its ability to improve flap salvage/survival and decrease the rate of false-positive events, defined as a loss of signal without a true blood flow disruption [33]. Kind et al. evaluated a series of 147 free flaps monitored by implanted Doppler probe and reported a 100% rate of flap salvage with a 3.4% rate of false-positive events [34]. In addition, a meta-analysis of 547 patients demonstrated the Doppler probe to be a safe and effective monitoring technique after free flap reconstruction with a strong trend toward improved salvaged rates without increasing the rate of unnecessary reoperations [33]. The Flow Coupler is an advancement in the implantable Doppler probe concept as it represents a fusion between the venous coupler and a 20 MHz micro-Doppler probe and allows the surgeon to complete the microvascular anastomosis while simultaneously monitoring vascular patency [35, 36].
Several studies have demonstrated the implantable Doppler can be used as a trusted adjunct to clinical exam for close monitoring of blood flow after free flap reconstruction; however device implantation through placement of the cuff around the vein can be technically challenging and increase operative times [35]. Although published data regarding Flow Coupler is limited, its use can reliably identify a potential vascular crisis without requiring a separate procedure to apply the device.
Tissue Oximetry
Survival of microsurgical tissue transplants depends on tissue perfusion and oxygenation. Successful reperfusion of compromised flaps depends on early detection with quick reestablishment of blood flow. Flap salvage rates have an inverse relationship to the time interval marking the onset of ischemia and its clinical recognition. Tissue oximetry measurement using near-infrared spectroscopy is a noninvasive method of tissue monitoring and provides continuous, real-time numeric data in comparison to the qualitative information provided by the implantable Doppler [37]. The scattering and absorption of wavelengths of near-infrared light is measured by near-infrared spectroscopy and is related to the oxygen content of hemoglobin within the tissues being monitored. A surface probe is placed on the flap which allows measuring of the oxygen saturation within the cutaneous layer of the flap. The hemoglobin concentration of a flap is relatively constant; therefore changes in flap perfusion can be quickly detected prior to the development of obvious clinical signs [38]. Lin et al. support the routine use of tissue oximetry monitoring in postoperative patients, showing a significant decrease in the number of flaps requiring reoperation [39].
Mastectomy Modifications
Dramatic advancements have been made in the surgical management of breast malignancies since the introduction of the radical mastectomy by Halsted [40]. Radical mastectomy includes en bloc resection of the breast gland and skin, pectoralis muscles, and axillary lymph nodes in order to achieve local disease control. This approach successfully ensured patient survival but was associated with significant postoperative morbidity. Halsted’s principles and radical mastectomy mark an important milestone in the history of breast cancer surgery and remained the standard surgical treatment for almost a century.
Over the last 50 years, the results of clinical trials as well as advancements in mammography and neoadjuvant therapies have helped revolutionize the surgical approach to breast cancer. Less aggressive versions of the radical mastectomy began to emerge starting with the modified radical mastectomy (MRM ), also known as the non-skin-sparing mastectomy (NSSM ) [41]. In contradistinction to the radical mastectomy, MRM removes all breast tissue and level I/II axillary lymph nodes while sparing the pectoralis musculature. By the early 1980s, it was established that BCS, consisting of wide local excision (lumpectomy) and subsequent radiation, has an equivalent survival rate when compared to mastectomy [42]. This observation, in addition to significantly decreased morbidity and improved cosmetic outcomes associated with BCS, secured its position as the treatment of choice for most low-grade invasive breast cancers for the past 20 years [43].
Despite the benefits of BCS , mastectomy rates in the early-stage breast malignancy population continue to rise [44]. The reason for this is likely multifactorial and includes the anxiety provoked by the need for prolonged surveillance, the perceived danger associated with a more conservative surgical approach, and prophylaxis required for patient populations at high risk for breast cancer development. In addition, surgical techniques have developed over the years, arming plastic and reconstructive surgeons with the ability to offer a variety of reconstructive options with excellent aesthetic results.
Skin-Sparing Mastectomy
The skin-sparing mastectomy (SSM ) was developed in the early 1990s by Toth and Lappert and involves removal of the breast gland and nipple-areolar complex (NAC) while preserving the overlying skin envelope and inframammary fold (IMF) [45, 46]. Maintenance of these critical landmarks allows the breast to assume a more natural shape and contour and facilitates the process of immediate breast reconstruction with any technique [47]. Incision patterns employed in SSM are limited to the immediate periareolar skin and are easily concealed with completion of nipple reconstruction or areolar tattoo.
The goal of the breast surgeon in SSM is to secure negative margins and to provide optimal cosmetic results. The balance between the two depends on mastectomy skin flap thickness, which is recommended to be approximately 10 mm. The surgical literature has confirmed the oncologic safety of SSM, finding no significant difference in local recurrence or disease-free survival when compared to traditional mastectomy. The combination of SSM and immediate breast reconstruction is also safe in patients with advanced Stage IIB and III breast cancer [47].
Nipple-Sparing Mastectomy
First reported by Hinton in the 1980s, the nipple-sparing mastectomy (NSM ) is more frequently used today and differs from the SSM by sparing the nipple-areolar complex (NAC). The NAC is a unique visual detail of a breast, and its preservation has proven to be a safe surgical option in a select group of patients, providing superior aesthetic outcomes and improved patient psychological well-being [48]. A recent systematic review highlights several factors that facilitate the process of appropriate patient selection to minimize breast cancer recurrence rates. These criteria include peripherally located tumors <5 cm in diameter, tumors located >2 cm from the NAC, lack of HER2 overexpression, and positive ER/PR status [49].
Common problems specific to NSM include nipple ischemia, partial or complete NAC loss, and nipple malposition (Fig. 7.3). Data regarding risk factors contributing to NSM complications are limited, conflicting, and variable, leading to a lack of consensus with respect to optimal surgical techniques. In light of this, Donovan et al. evaluated the effects of NSM incision location on rates of NAC ischemia, wound infection, and implant loss. There was an increased rate of NAC necrosis with use of periareolar incisions, while inframammary incisions had fewer ischemia-related compilations [50]. A retrospective review of 340 NSMs by a single surgeon over a 5-year period was completed to define specific and critical steps that should be utilized during NSM to reduce the risk of nipple necrosis and optimize cosmetic outcomes. The overall rate of nipple necrosis was reported to be 2.6%, with complete necrosis occurring in three cases (0.8%) and partial loss affecting six patients (1.8%) [51]. The authors recommend:
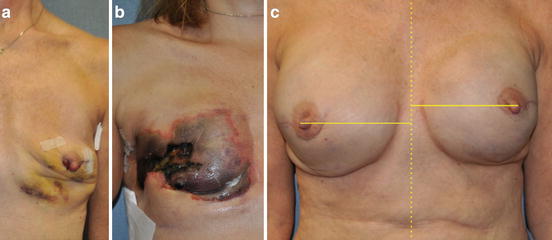
- 1.
Preservation of major perforating vessels supplying the breast skin, specifically the second intercostal perforator off the internal mammary artery, which feeds the smaller vessels surrounding the nipple periphery.
- 2.
Careful elevation of skin flaps in the plane between subcutaneous fat and breast glandular tissue, while keeping in mind that the thickness of the fat and of the skin flap changes with a tendency to become thicker as dissection proceeds away from the NAC. This subtle detail requires close attention to ensure dissection continues in the appropriate plane. Accidental deviation can lead to inadvertent thinning of the flap and ultimate vascular compromise.
- 3.
Ensure proper incision placement. Three categories of incisions were described: radial (lateral and vertical), periareolar (medial or lateral extensions), and crease. Magnetic resonance imaging showed a significant reduction in perfusion inferior to the NAC, which may be the reason inframammary incisions have lower rates of nipple necrosis [51].
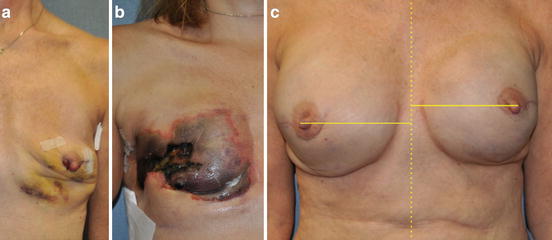
Fig. 7.3
Complications associated with nipple-sparing mastectomy (NSM). (a) Nipple ischemia demonstrated by inferior nipple mottling and desquamation. (b) Complete nipple and mastectomy skin flap necrosis. (c) Nipple areolar complex malposition
Breast Implant Technology
Two-stage implant-based breast reconstruction remains the most commonly performed type of breast reconstruction after mastectomy, as it does not significantly increase operative time or length of hospital stay, is a technically straightforward procedure performed through the mastectomy incision or scar, and may be performed in the outpatient setting (Fig. 7.4).
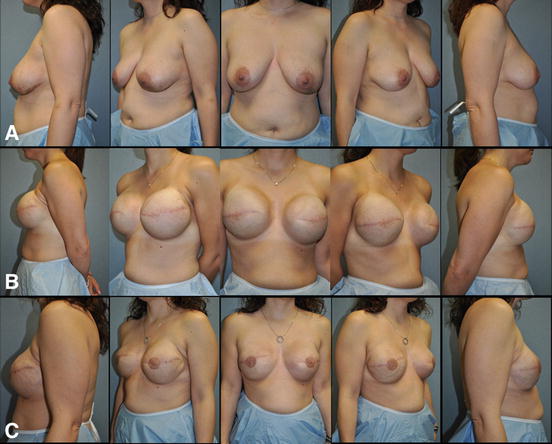
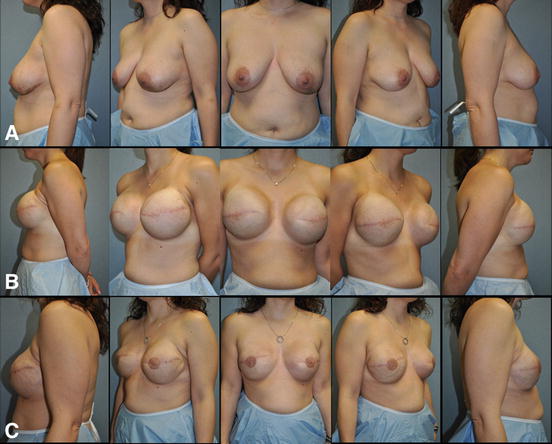
Fig. 7.4
Two-stage implant-based breast reconstruction . (a) 36-year-old female with left invasive ductal carcinoma managed with bilateral mastectomy and immediate submuscular tissue expander placement. (b) Fully inflated submuscular tissue expanders in preparation for exchange for final breast implants. (c) Patient at the completion of breast reconstruction with 500 cc smooth round moderate profile plus silicone gel breast implants, nipple reconstruction, and areolar tattoo
Tissue Expander Advances
The earliest of tissue expanders were designed by Dr. Chedomir Radovan and consisted of a silicone prosthesis with remotely placed valves/ports allowing for fluid injection in one port and its removal from the other [52]. Since then, breast tissue expanders have improved and have superior silicone shells and integrated filling ports. This type of expander is associated with lower infection rates and complications caused by using a remote valve/port such as valve flipping, tube kinking, and pain associated with repeated needle sticks for port access.
More recent technological advances in breast tissue expanders include changes in expander shape and the development of self-filling expanders. The round shape of initial expanders has been modified to a more anatomic or “tear-drop” shape, which allows for a more natural breast appearance as well as accommodation of shaped devices at the second stage. The geometry of this device allows for differential expansion and maximization of the lower pole of the breast. These expanders have produced lower complication rates with reported capsular contracture rate of 3%, infection rate of 1.2%, and no valve dysfunction [53]. Self-filling tissue expanders were introduced due to the theoretical benefit of fewer office visits for expansion, decreased number of needle sticks for implant access, and the potential for patient-controlled inflation of prostheses. They contain either an osmotic agent or gas to allow for progressive tissue expansion.
First-generation osmotic expanders were devised by Austed and Rose [54]. Rapid expansion of these expanders, which did not have an envelope, resulted in soft tissue ischemia at times. Modifications of early osmotic expanders, including integration of a silicone membrane, allow for safer, slower expansion speed. Vinyl pyrrolidone, an osmotic hydrogel, is the agent utilized within this type of expander producing migration of water through the silicone membrane of the device resulting in progressive expansion. This is in contrast to the self-inflating expander which use gas (CO2) for filling. This expander type includes a small cylinder which releases small amounts of gas into the expander allowing for progressive expansion. Gas-based self-filling expanders were introduced by Connell and have shown 100% expansion success rate with minor adverse events [55, 56].
Prosthesis Location Modifications
Recreation of the inframammary fold and maintenance of natural breast ptosis is the ultimate goal of breast reconstruction thus making expander shape and implant location of critical importance. Originally, breast tissue expanders were placed in the subcutaneous plane. This evolved to the preference of the subpectoral plane due to lower rates of capsular contracture which produced the firm, round, and unnatural appearance associated with subcutaneous placement. Subsequently, the partial submuscular plane was popularized due to the ability to place the prostheses lower on the chest wall augmenting lower pole expansion of the breast and accentuating the inframammary fold resulting in improved breast cosmesis. Currently, the inferolateral portions of the breast prosthesis are covered by the lateral inferior chest musculature (serratus anterior) or a form of dermal graft (see below).
Final Breast Implant Advances
The evolution of the contemporary breast prosthesis began with the “first-generation” silicone gel implant introduced by Cronin in 1962 and the first saline-filled breast implant by Arion in 1965. Since that time, both silicone gel and saline-filled implants have undergone several technical alterations and improvements to maximize safety and aesthetic results [57]. Saline implants are available as deflated devices which are to be filled with normal saline at the time of implantation. This allows for placement through a small incision as well as subtle size adjustments to be made at the time of surgery. These implants tend to be less popular than their silicone counterpart due to a less natural consistency similar to that of water compared to the more viscous natural breast tissue. In addition, overfilling may lead to a spherical shape and scalloping along the edge of the implant causing a firmer feel upon palpation.
Several versions of silicone gel implants have been developed since their introduction and differ with respect to the characteristics of the silicone gel filler, characteristics of the silicone shell surrounding the filler, and implant shape. Silicone is a mixture of polymeric molecules which can exhibit different physical properties depending on polymer chain length and degree of cross-linking between polymer chains [58]. There are two basic categories of silicone options available for use in breast implants, and they are termed “fluid form” and “form stable.” The “fluid-form” liquid silicones are short polymers with very little cross-linking and have the consistency of oil [59]. They are usually used as surgical lubricants and are not cohesive enough to hold a given anatomic shape. When enough cross-linking is achieved, a more viscous “form-stable” silicone gel is created which allows the implant to maintain its dimensions and hold a given shape, affording the surgeon more control over the device. Technology exists to measure the cohesivity of silicone gel implants allowing measurement of implant stiffness. Form stability correlates with lower rates of capsular contracture, implant rupture, rippling, and improved patient satisfaction compared to low-cohesive fillings [60].
Extensive chemical cross-linking of the silicone gel polymers will create a solid form of silicone called an elastomer shell. Implant shell modifications, including barrier layers, have been introduced to protect the silicone gel filler. Shell characteristics, such as shell thickness, are important to consider as they contribute to the stability of implant shape. The maintenance of gel distribution within the implant shell helps to preserve this stability. The cohesivity of the gel and gel-shell fill ratio improves shape maintenance and varies among implant shapes. In addition to shell thickness, surface characteristics of the shell have undergone modifications with the ultimate goal of creating a surface texture that can minimize implant capsule formation [61]. The evolution of textured implants stemmed from the introduction of polyurethane-coated implants. These were foam-coated implants which were eventually dismissed due to concerns about possible carcinogenic conversion after chemical degradation of the foams. In the 1980s, a shift from foam-coated shells to textured silicone shells was made. Studies have shown that the pore size is critical to allow for tissue adherence leading to the adhesive effect of implant texturing and implant stabilization.
Round-shaped implants have been available for breast reconstruction since the 1980s and are described as having a “disk-like” shape. Several years later, anatomic or “tear-drop”-shaped implants were popularized outside of the United States and were subsequently approved by the FDA for use in the United States in 2013 [62]. Anatomic implants were developed to optimize the natural look of the reconstructed breast through implementation of a lower point of maximal projection resulting in a more prominent lower breast contour. They provide greater versatility and control of breast shape, which leads to a more “natural” aesthetic result. Although the anatomic, form-stable, silicone gel implants are gaining popularity, gel fracture and implant rotation are known risks associated with their use.
The “first-generation” prosthesis was anatomically shaped (“tear-drop”), filled with a viscous silicone gel, and covered with a smooth, thick outer silicone elastomer shell. In addition, they had Dacron fixation patches on their posterior aspect to maintain the proper position of the implant on the chest wall. The shell kept the liquid filler in one place creating a natural breast-like shape. Unfortunately, these devices had a high rate of capsular contracture due to the quality of the shells and the lack of cohesivity of the gel. Second-generation silicone gel implants were introduced about a decade later in attempts to address these complications. They were round in shape, had a thinner shell without Dacron patches, and were filled with a less viscous silicone gel. While these looked and felt more natural, the combination of the lower viscosity gel filler and permeable shell made them more likely to rupture and leak resulting in “gel bleed”. The third-generation implants were developed in the 1980s to reduce the rate of implant rupture with subsequent gel migration by using a stronger multilayer silicone elastomer shell. Despite attempts to improve implant design, a moratorium on the use of third-generation silicone gel breast implants was issued by the FDA in 1992 in response to concern about the possible association between “gel bleed” and the development of connective tissue disorders. Subsequent studies and literature reviews failed to show this relationship, and restrictions on their use were lifted in 2006. The most recently designed silicone-filled breast implants are manufactured using the highest standards, concentrating on optimal shell thickness and gel cohesiveness to create a more natural feeling breast with reduced rates of complications [57]. Currently, fourth- and fifth-generation silicone implants are utilized. Fifth-generation implants have a more cohesive “form-stable” silicone gel and a textured surface and were manufactured with improved quality control allowing for several surface textures and implant shapes. Although they have been shown to be safe, a possible association between their use and anaplastic large-cell lymphoma (ALCL) was reported by the FDA in 2011. Although extremely rare, this finding must be reported to anyone considering having silicone breast implants.
Single-Stage Implant-Based Breast Reconstruction
Recently, single-stage implant-based breast reconstruction has been popularized [63]. This technique involves the insertion of the final breast implant at the time of the initial procedure (Fig. 7.5). The ability to perform single-stage implant-based breast reconstruction depends on multiple factors. The amount and quality of the remaining mastectomy skin plays a large role in the ability to perform this operation safely in a single stage. To this end, the reconstructive surgeon must understand what surgical options the oncologic surgeon is willing to offer to each patient, as skin-sparing mastectomy, nipple-sparing mastectomy, and other advanced mastectomy techniques are not available at all institutions nor are they applicable to every individual. Likewise, the plan for adjuvant therapies, specifically any indications for PMRT, must be evaluated by the reconstructive surgeon due to the fact that radiotherapy significantly increases the risk of complications associated with prosthetic-based reconstruction. The immediate direct-to-implant reconstruction has been shown to reduce operating room time, cost, and potential added morbidity associated with a two-step expander/implant reconstruction and is ideal for certain patient populations allowing quicker return to normal daily activities [64].
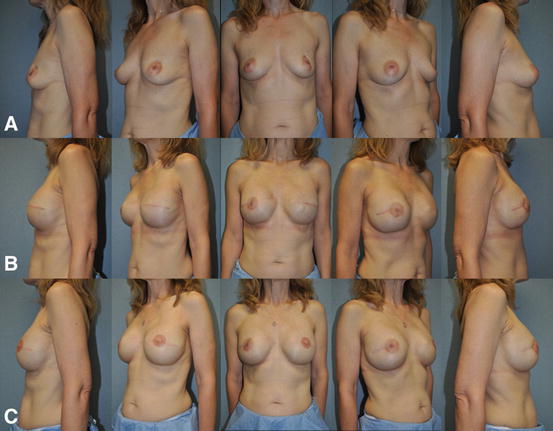
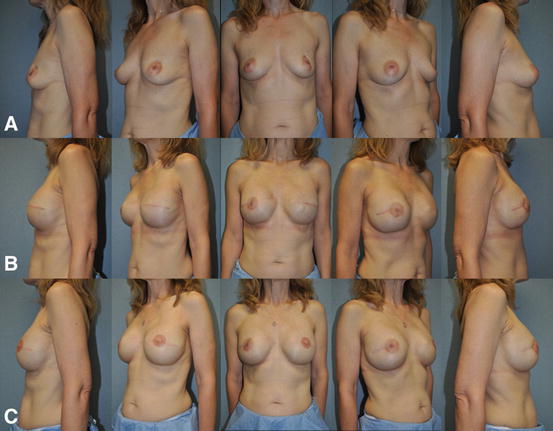
Fig. 7.5
Single-stage implant-based breast reconstruction . (a) 48-year-old female with left invasive ductal carcinoma managed with left skin sparing mastectomy, right prophylactic nipple-sparing mastectomy, and immediate bilateral submuscular 350 cc smooth round moderate profile plus silicone gel breast implants. (b) Postoperative appearance after single-stage implant-based breast reconstruction. (c) Patient at the completion of breast reconstruction with liposculpting (16 cc) of the right upper inner breast pole and left nipple reconstruction with C-V flaps and areolar tattoo
Dermal Graft Utilization
The use of autologous dermal grafts in reconstructive surgery is not a new technique but one that has been recently applied in breast surgery. Implant-based breast reconstruction techniques have evolved from total submuscular coverage of the breast implant to a dual plane technique which leaves the lower third of the prosthesis/expander in a more superficial plane and only covered by mastectomy flaps. The use of autologous dermis or allogeneic acellular dermal matrices have been described to act as a “hammock” between the inferior border of the pectoralis major and inframammary fold. The benefits of autologous dermal tissue stem from the fact that it is not a foreign material, it is cheap, and it is readily available from common donor sites such as the abdomen.
The use of nonantigenic cadaveric human dermis or acellular dermal matrices (ADMs) to cover the lower pole of the implant expander was introduced in 2005 and has gained popularity due to an acceptable safety profile and improved breast aesthetics [65]. ADMs have provided a surgical option to address the previously reported challenges with two-staged breast reconstruction, including lack of total expander muscular coverage, low initial fill volumes, numerous outpatient visits, and poor inframammary fold definition, in addition to being available “off the shelf” obviating the need for a separate donor site. ADMs are soft tissue matrix grafts that are created by decellularization, leaving the extracellular matrix intact to function as a scaffold for cellular ingrowth and revascularization (Fig. 7.6). The tensile strength and low elasticity of acellular dermis allow tension to be applied preferentially to the graft during expansion instead of direct transmission to mastectomy flaps. The purpose of using ADMs in expander/implant reconstruction is to improve upon and maintain the goals of breast reconstruction including maintenance of inframammary fold and creation of breast ptosis. Inferior implant coverage with ADMs has the advantage of adding soft tissue thickness overlying the prosthesis reducing implant visibility, palpability, and increased initial fill volumes, therefore decreasing expansion duration. The use of ADMs may reduce the incidence of capsular contracture [66, 67].
