Abstract
The pattern of regular ovulatory cycles required for normal reproduction in women is achieved through precise functional and temporal integration of stimulatory and inhibitory signals from the hypothalamus, the pituitary, and the ovary. The process begins with pulsatile secretion of gonadotropin-releasing hormone (GnRH) from the hypothalamus into the pituitary portal venous system, where it regulates the synthesis of follicle-stimulating hormone (FSH) and luteinizing hormone (LH) in the anterior pituitary and their secretion into the circulation. FSH and LH stimulate follicle development, ovulation, and corpus luteum formation and the coordinated secretion of estradiol, progesterone, and the inhibins from the ovary; these hormones control the dynamic modulation of gonadotropin secretion through hypothalamic and direct pituitary mechanisms.
Key words
Gonadotropin-releasing hormone, luteinizing hormone, follicle-stimulating hormone, estrogen, progesterone, inhibin, feedback, kisspeptin, racial differences, human
Reproductive Axis
- ◆
Genetic findings from patients with congenital deficiencies in gonadotropin secretion have advanced our understanding of the ontogeny and upstream regulation of GnRH.
- ◆
Kisspeptin, neurokinin B (NKB), and dynorphin are important regulators of GnRH synthesis and secretion and transduce gonadal feedback signals to GnRH neurons.
- ◆
Differential control of LH and FSH requires the integration of GnRH pulse amplitude and frequency with direct pituitary feedback from estradiol and the inhibins.
Normal reproductive function in women involves repetitive cycles of follicle development, ovulation, and preparation of the endometrium for implantation should conception occur in that cycle. This pattern of regular ovulatory cycles is achieved through precise functional and temporal integration of stimulatory and inhibitory signals from the hypothalamus, the pituitary, and the ovary ( Fig. 7.1 ). The reproductive system functions in a classic endocrine mode. The master hormone, gonadotropin-releasing hormone (GnRH), is secreted in a pulsatile fashion from the hypothalamus into the pituitary portal venous system. GnRH regulates the synthesis and subsequent release of follicle-stimulating hormone (FSH) and luteinizing hormone (LH) from gonadotropes within the anterior pituitary into the circulation. FSH and LH stimulate ovarian follicular development, ovulation, corpus luteum formation, and the coordinated secretion of estradiol, progesterone, inhibin A, and inhibin B. A key component of this system is the modulatory effect of ovarian steroids and inhibins on gonadotropin secretion. Ovarian steroids affect the amplitude and/or frequency of GnRH secretion through their effects on KNDy neurons, discussed further on, that act upstream of GnRH neurons in the hypothalamus. In addition, ovarian steroids and inhibins act directly at the pituitary level. Negative feedback restraint of FSH secretion is critical to the development of the single mature oocyte that characterizes human reproductive cycles. In addition to negative feedback controls, the menstrual cycle is unique among endocrine systems in its dependence on estrogen-positive feedback to produce the preovulatory LH surge that is essential for ovulation.

Neuroendocrine Components of the Reproductive Axis
Gonadotropin-Releasing Hormones
Luteinizing-releasing hormone (LHRH) was isolated, characterized, and synthesized in 1971 (see Chapter 1 ). The central role of this decapeptide in propagation of the species makes it fitting that Drs. Schally and Guillemin received the Nobel Prize in Physiology and Medicine in 1977 for its isolation. It was expected that separate releasing hormones for LH and FSH would be discovered. However, subsequent studies provided evidence that both LH and FSH are secreted in response to LHRH, resulting in the common use of the term GnRH for the decapeptide originally referred to as LHRH.
GnRH neurons differentiate in the olfactory placode, cross the cribriform plate into the forebrain, and migrate to the medial basal hypothalamus, where they establish connections with the pituitary portal system in the median eminence as part of the hypothalamic tuberinfundibular system. The initial leg of this migratory journey occurs along the scaffold of olfactory, vomeronasal, and terminal nerves. In the human there are approximately 7000 GnRH-expressing neurons in areas of the brain linked to gonadotropin regulation. Unlike neurons secreting other hypothalamic releasing factors, GnRH neurons do not exist in a defined nucleus but are scattered throughout the medial basal hypothalamus, with additional scattered neurons in the preoptic area.
Genetic studies in patients with isolated gonadotropin deficiency (IGD), with concomitant disruption of the olfactory system resulting in anosmia (Kallmann syndrome [KS]) or without anosmia (normosmic idiopathic hypogonadotropic hypogonadism [nIHH]) have led to unprecedented growth in our understanding of the complex neuroendocrine control of reproduction. Mutations in over 35 genes have now been discovered in this rare patient population. Validation of their function in animal and cell systems indicates that these can be broadly classified into four groups. Genes involved in early migration and axonal guidance of GnRH neurons on the path to their eventual home in the hypothalamus include Kallmann 1 (KAL1), chromodomain helicase DNA binding protein 7 (CHD7), sex-determining region of Y-box 10 (SOX10) , semaphorin-3A (SEMA3A), fasciculation and elongation protein zeta family zinc finger 1 (FEZF1) , fibronectin leucine-rich transmembrane protein 3 (FLRT3) , and IL-17 receptor D (IL17RD) . Genes involved in the control of GnRH secretion include kisspeptin and its receptor (KISS1/KISS1R) , tachykinin 3 and its receptor (TAC3/TACR3) , gonadotropin-releasing hormone 1 (GNRH1) , and dosage-sensitive sex reversal 1 (DAX1) , also known as nuclear receptor subfamily 0, group B, member 1 (NROB1) . Genes that appear to play a role in both GnRH ontogeny and function include fibroblast growth factor 8 and its receptor fibroblast growth factor receptor 1 (FGF8/FGFR1), prokineticin 2 and its receptor (PROK2/PROKR2), heparan sulfate 6-O-sulfotranferase 1 (HS6ST1), repeat domain 11 (WDR11), AXL receptor tyrosine kinase (AXL) , NMDA receptor synaptonuclear signaling and neuronal migration factor (NSMF) , dual-specificity phosphatase 6 (DUSP6) , sprouty homologue 4 (SPRY4) , and fibroblast growth factor 17 (FGF17). Finally, the genes involved in gonadotrope stimulation that have been discovered to date in association with IGD include DAX1 and gonadotropin-releasing hormone receptor (GNRHR) . It is likely that this list will continue to grow.
Mammals, including humans, simultaneously express more than one molecular form of GnRH ( Table 7.1 ). Whereas GnRH is located on chromosome 8p and works through its own receptor, which is encoded on chromosome 4, GnRH II is located on chromosome 20p13 and works through its unique receptor, which is encoded on chromosome 1. GnRH II is widely expressed both within and outside the brain. It likely plays a role in reproductive behavior in lower animal species. It is a potent stimulator of LH and FSH in vitro and in vivo in animal models, but its role in the human is unknown. There is some evidence in lower animal species that GnRH III, initially isolated from lamprey, may have preferential FSH-releasing properties. However, a GnRH III consensus sequence has not been found in the human genome, making it unlikely that GnRH III plays a role in human reproduction.
GnRH I (Mammal) | pGLu- His- Trp- Ser- Tyr- Gly- Leu- Arg – Pro- Gly- NH2 |
GnRH II (Chicken I) | pGLu- His- Trp- Ser- His- Gly- Trp- Tyr – Pro- Gly- NH2 |
GnRH III (Lamprey III) | pGLu- His- Trp- Ser- His- Asp- Trp- Lys – Pro- Gly- NH2 |
Pulsatile Secretion of Gonadotropin-Releasing Hormone
A prominent feature of the reproductive system is the absolute requirement for pulsatile secretion of GnRH into the pituitary portal system for normal gonadotropin secretion. The now classic studies of Knobil and colleagues in hypothalamic-lesioned monkeys receiving GnRH first showed that intermittent stimulation of the pituitary results in secretion of LH and FSH, while constant GnRH stimulation is associated with the suppression of gonadotropin levels. Isolated GnRH neurons exhibit an intrinsic pulsatility, but external influences appear to modify and coordinate the secretion of GnRH, influencing both the amplitude and frequency of pulsatile GnRH secretion.
Neuromodulators of Gonadotropin-Releasing Hormone Secretion
Although a number of neurotransmitters are involved in the control of GnRH secretion in animal species, only a few have been shown to have an effect in the human. Although there is evidence for a stimulatory role of the α-adrenergic system in several animal models, it is much less likely that it plays a role in control of the human menstrual cycle. The role of the dopaminergic system remains controversial, but some studies that have documented an increase in LH pulse frequency in response to a dopamine antagonist in women with hypothalamic amenorrhea suggest that dopamine may inhibit GnRH secretion in women.
Kisspeptin
Knockout models suggest that there is considerable redundancy in the systems that ultimately control GnRH secretion; however, it is now firmly established that the kisspeptin pathway is a key upstream modifier of GnRH secretion. As with the genes that are now known to control the developmental migration of GnRH neurons, a role for kisspeptin in reproduction was initially discovered in studies in patients with IGD that identified mutations in the gene encoding the kisspeptin receptor ( KISS1R , formerly known as G protein–coupled receptor 54 [GPR54] ) and also shown in a mouse knock-out. Kisspeptin is an extremely powerful stimulator of LH, an action that is blocked by a GnRH antagonist indicating that the effect on LH is through GnRH. The kisspeptin system is thought to play a dominant role in the onset of puberty and has also been implicated in estrogen negative feedback in the median eminence. Studies of kisspeptin administration in humans—performed using different isoforms and subcutaneous, intravenous bolus, or intravenous infusion modes of administration—have demonstrated a marked difference in LH response depending on cycle phase and hormonal status. The response to kisspeptin is consistently robust in the late follicular, preovulatory, and luteal phases of the menstrual cycle and in postmenopausal women, while there is some inconsistency in the early follicular phase with a lower, and in some cases absent, LH response to kisspeptin.
In rodents and sheep, there is ample evidence that positive feedback is manifest at the hypothalamus as well as the pituitary, with generation of a marked increase in GnRH at the time of the midcycle gonadotropin surge. Kisspeptin neurons in the anteroventral periventricular nucleus (AVPV) have now been implicated in this estrogen-positive feedback on GnRH secretion in rodents. The relationship of the AVPV to the suprachiasmatic nucleus (SCN) in the rodent provides a potential mechanism for the known circadian timing of the proestrus surge in the rodent.
Despite compelling evidence in lower animal species, it is likely that kisspeptin control of GnRH does not play a role in the midcycle surge (MCS) in women. Studies in women demonstrate a paucity of kisspeptin neurons in an analogous hypothalamic region, and evidence discussed further on demonstrates that a GnRH surge is not required for the generation of a midcycle LH surge in GnRH-deficient women, and further suggests that a GnRH surge is not present in normal women.
Neurokinin B
NKB, which is encoded by the tachychinin 3 gene (TAC3) and its cognate receptor, NK3R, encoded by TACR3 , has also been implicated in the normal control of GnRH secretion through genetic studies in patients with IGD. NKB stimulates LH secretion, acting upstream of the GnRH neuron. TAC3 and KISS are colocalized in the human as well as in other species, particularly in the arcuate/median eminence. There is significant evidence that the effect of NKB on GnRH is exerted primarily through kisspeptin. NKB agonists stimulate gonadotropin secretion, while NKB3 antagonists inhibit LH secretion but do not appear to do so in the setting of high estrogen, as in the preovulatory phase. Interestingly, the NKB3/NK3R system also plays a role in hot flashes in estrogen-deficient states.
Endogenous Opioids/Dynorphin
There is substantial evidence for the involvement of endorphins in transducing the negative feedback effects of progesterone on pulsatile GnRH secretion from studies using the opioid receptor blocker naloxone in women. However, naloxone binds not only to the mu receptor but also to the kappa and gamma receptors; thus these early studies could not provide mechanistic specificity. Dynorphin, which binds to the kappa-opioid receptor, has now been identified as the key mediator of progesterone negative feedback. In a variety of animal species and the human it has been shown that kisspeptin, NKB, and dynorphin are coexpressed in cells in the arcuate nucleus/median eminence now referred to as KNDy neurons. These neurons express estrogen, progesterone, and androgen receptors and are thought to mediate gonadal steroid–negative feedback on GnRH secretion, with increasing evidence that they are also involved in the initiation and termination of GnRH secretion resulting in its pulsatile secretion. Gamma-amino butyric acid (GABA) may also be involved in mediating estrogen negative feedback on GnRH secretion, particularly in the arcuate/median eminence.
RFamide-Related Peptides
RFamide-related peptides (RFRPs) are the mammalian orthologues of gonadotropin inhibitory hormone (GnIH), which was first discovered in the hypothalamus of the quail. In the human, RFRP-1 and RFRP-3 neurons send axonal projections to GnRH neurons. RFRPs are secreted into the pituitary portal system, and their receptor, G protein–coupled receptor 147 (GPR147), is present on gonadotropes as well as in the hypothalamus. Taken together with functional data from animal and cellular systems, these findings suggest that RFRPs function at both the hypothalamus and pituitary to regulate the secretion of LH and FSH. Interestingly, there is also evidence that RFRPs increase food intake in sheep without reducing energy expenditure. There are currently limited data to address the role of these peptides in the human. A 3-hour infusion of custom-synthesized GnIH resulted in a modest suppression of LH secretion in postmenopausal women but failed to inhibit LH secretion in response to pulses of kisspeptin-10 in men. Additional studies will be required to ascertain the physiology and potential therapeutic role of RFRPs in men and women.
Sleep and Circadian Effects on Gonadotropin-Releasing Hormone Secretion in Women
Endocrine systems are profoundly influenced by both sleep and endogenous circadian rhythms, which are intrinsic rhythms that persist in the absence of sleep or other environmental cues. Diurnal (day and night) rhythms of LH and gonadal steroids have been well described in men and women. However, studies in which sleep and other environmental cues were controlled have failed to demonstrate an endogenous circadian rhythm of LH or FSH in early-follicular-phase ( Fig. 7.2 ) and postmenopausal women despite the presence of robust circadian rhythms of temperature, cortisol, and thyroid-stimulating hormone (TSH).

In contrast, there is compelling evidence that sleep directly affects the pulsatile secretion of LH and presumably that of GnRH. Studies that have separated the effects of sleep from time of day demonstrate that during puberty in boys and girls, pulsatile LH secretion is increased during sleep. More recent studies indicate that LH pulses are most commonly preceded by slow-wave sleep (SWS), and further studies have shown that even with repeated sleep interruption, 20 minutes of accumulated sleep is associated with LH pulse onset in this population. These studies suggest that factors associated with SWS stimulate GnRH secretion or that there is an upstream regulator of both GnRH secretion and deep sleep in puberty.
Paradoxically, with maturation of the reproductive system and the onset of ovulatory menstrual cycles, there is a notable slowing of pulsatile LH secretion at night in the early follicular phase of the cycle. Sleep reversal studies in women have demonstrated that the early-follicular-phase nighttime slowing is due to sleep rather than time of day. Importantly, within sleep, brief periods of wakefulness are associated with the onset of LH pulses ( Fig. 7.3 ), while SWS is inhibitory to LH pulses. Preliminary data relating luteal-phase characteristics to early-follicular-phase nighttime pulse frequency suggests that prior progesterone exposure sensitizes the GnRH pulse generator to the inhibitory effects of sleep in the early follicular phase. In this regard, it is of interest that GnRH pulse frequency, as determined by the pulsatile secretion of free α subunit (FAS), is also slower during sleep than wake in postmenopausal women whose gonadal steroid levels are similar to those in early puberty. Although the effect of sleep is much less than in normally cycling women, this finding suggests that the inhibitory effect of sleep on LH pulse frequency is related not only to prior progesterone exposure, but that other factors relating to the development of a mature reproductive axis are involved.

Gonadotropin-Producing Cells of the Pituitary
LH and FSH are synthesized in gonadotropes, which comprise between 7% and 15% of the cells in the pituitary. Immunohistochemical studies in the rat indicate that approximately 70% of gonadotropes stain for both LH and FSH, while the remainder stains for LH or FSH in approximately equal numbers. As the animals approach the day of the gonadotropin surge in proestrus, monohormonal cells begin to express both βLH and βFSH, while a population of cells that express growth hormone (GH) also express the gonadotropin subunits.
Biosynthesis of intact gonadotropins involves (1) translation of βLH, βFSH, and the common gonadotropin α-subunit; (2) posttranslational modification and folding; (3) combination of the β and α subunits; and (4) modification of the oligosaccharide residues on LH and FSH as they traverse the Golgi apparatus. FSH is secreted primarily in a constitutive manner, with little storage. In contrast, LH is packaged into granules and stored. LH and the gonadotropin-FAS are then secreted in response to GnRH stimulation of the gonadotrope. Multiple isoforms of LH and FSH, differing in their carbohydrate structure and charge, coexist in both pituitary and serum. More basic forms of both LH and FSH yield a greater in vitro potency but a shorter half-life in the circulation while, the opposite is true for less basic forms. The greater number of sialic acid residues on FSH prolong its half-life, whereas the greater number of sulfonated N-acetyl-galactosamine (GalNAc) asparagine-linked oligosaccharides on LH are associated with more rapid clearance due to binding to a specific hepatic receptor. Sulfonation and sialylation of LH and FSH vary across the menstrual cycle and in the absence of gonadal steroids; postmenopausal women have a greater preponderance of sialylated forms of both LH and FSH. The number of sulfonated and sialylated residues on LH and FSH is tightly linked with hormone clearance in women. Thus, the disappearance of LH following GnRH receptor blockade with a potent GnRH antagonist is significantly prolonged in postmenopausal women compared with women in the follicular phase and at the MCS, while the disappearance of FAS, which does not have oligosaccharide residues attached to its protein backbone, is unaffected by the absence of gonadal function ( Table 7.2 ).
Luteinizing Hormone | Gonadotropin-Free α Subunit | |||
---|---|---|---|---|
Baseline (IU/L) Mean ± SEM | T1/2 (min) Mean ± SEM | Baseline (pg/mL) Mean ± SEM | T1/2 (min) Mean ± SEM | |
Postmenopausal | 62 ± 3 * | 139 ± 35 * ,† | 774 ± 45 * | 51 ± 26 |
EFP, LFP, ELP | 10 ± 1 | 57 ± 28 | 266 ± 44 | 41 ± 12 |
MCS | 56 ± 11 * | 78 ± 20 ‡ | 627 ± 122 † | 41 ± 19 |
There is also evidence that gonadotropin secretion is modulated by a factor or factors related to obesity. Serum levels of LH are inversely related to body mass index (BMI) in normal women and in women with polycystic ovary syndrome (PCOS). Further studies have indicated that the inhibitory effect of obesity on LH secretion in PCOS is not mediated at the hypothalamus but is associated with a decrease in both the pituitary response to GnRH and the half-life of endogenous, but not exogenous, LH. The latter finding is consistent with the increase in sulfonated isoforms of LH and FSH as a function of increasing BMI in women with PCOS. Recent studies have shown that in obese men and women without PCOS, the combination of an insulin and lipid/heparin infusion, which results in increased free fatty acids and triglycerides, suppresses LH and FSH. Taken together, these studies may suggest that the effects of obesity on pituitary secretion of LH are mediated through effects of insulin and free fatty acids.
Differential Control of Luteinizing Hormone and Follicle-Stimulating Hormone Secretion
Although secreted from a common cell type within the gonadotrope, LH and FSH have markedly different functions in the control of ovarian physiology. These differences in function are reflected in their different patterns of secretion during normal reproductive cycles. The divergent control of LH and FSH is achieved through a combination of differential control of the synthesis and secretion of LH and FSH by the pattern of GnRH stimulation, the preferential control of FSH synthesis by the activin/follistatin system, and differential feedback by ovarian steroids and the inhibins on LH and FSH at the pituitary. Understanding the control of LH and FSH secretion is critical to our understanding of the dynamics of the menstrual cycle.
Gonadotropin-Releasing Hormone
FSH is secreted along with LH in response to acute stimulation by GnRH, but the relative role of GnRH in the overall control of FSH synthesis is much less than that for LH. Blockade of the GnRH receptor using a specific GnRH receptor antagonist results in 90% inhibition of LH secretion but only 40% to 60% inhibition of FSH. Synthesis and secretion of LH and FSH are differentially controlled by the amplitude and frequency of GnRH stimulation. LH is highly responsive to increases in the dose of GnRH, whereas FSH is relatively insensitive to GnRH dose. A physiologic frequency of GnRH results in synthesis and secretion of all three gonadotropin subunits; however, increases or decreases from the physiologic frequency have differential effects on LH and FSH ( Table 7.3 ). Slow frequencies of GnRH stimulation favor synthesis and secretion of FSH in vitro and are associated with an increase in FSH in human studies in settings where gonadal feedback is low. In GnRH-deficient men and women, an increase in the frequency of GnRH stimulation increases mean levels of LH with no appreciable change in FSH ( Fig. 7.4 ), effects that have implications for the pathophysiology of PCOS, which is characterized by an increased frequency of pulsatile GnRH stimulation of the pituitary and an increase in the LH to FSH ratio. The direct effect of GnRH pulse frequency on GnRH receptor number underlies the frequency modulation of LH and FSH secretion, at least in part. In addition, increased GnRH pulse frequency increases follistatin, which would attenuate activin stimulation of FSH synthesis, as discussed further on.
Luteinizing Hormone | Follicle-Stimulating Hormone | |
---|---|---|
Increased Frequency | ||
In v itro | ||
mRNA | ↑ | → |
GnRH-deficient men/women | ||
Mean | ↑ | →↓ |
Amplitude | ↓ | — |
Decreased Frequency | ||
In v itro | ||
mRNA | ↓ | ↑ |
GnRH-deficient men/women | ||
Mean | ↓ | →↑ |
Amplitude | ↑ | — |

Whereas an increase in GnRH pulse frequency increases the synthesis and mean levels of LH, LH pulse amplitude is inversely related to GnRH pulse frequency. Studies in GnRH-deficient men and women have shown that slower GnRH pulse frequencies are associated with higher LH pulse amplitude in response to GnRH, whereas faster frequencies are associated with a decrease in the LH pulse amplitude response to GnRH. This decrease in LH amplitude seen with pulse frequencies that are faster than those encountered in physiologic settings may be the earliest sign of the pituitary desensitization that is well recognized in association with continuous infusions of GnRH or the use of a GnRH agonist.
Autocrine/Paracrine Regulation of Gonadotropins: Activin, Inhibin, and Follistatin
Activins, inhibins, and follistatins were first discovered as gonadal factors with preferential actions on FSH secretion from pituitary gonadotropes. The inhibins, named for their inhibitory effect on FSH secretion in pituitary cell cultures, are heterodimers that are composed of one of two β-subunits, βA or βB, and a closely related α-subunit to form inhibin A or inhibin B. In contrast, the activins, which stimulate the synthesis and secretion of FSH, are homodimers of two β-subunits. Activin is secreted by gonadotropes and other pituitary cell populations. Like other members of the TGF – β superfamily of growth and differentiation factors, activins exert most of their effects by autocrine or paracrine mechanisms. Activins sequentially interact with one of the two known type II activin receptors, ActRII or ActRIIB, and the type 1 receptor, activin receptor–like kinase 4 (ALK4). The expression of FSHβ is extremely sensitive to the stimulatory action of activins, which work in concert with and are permissive for the actions of GnRH through promotion of transcription of both FSHβ and the GnRH receptor.
The inhibins act as specific antagonists of the FSH stimulatory actions of activin by binding to betaglycan and sequestering the type-II activin receptors. Although inhibin is made in the pituitary, circulating inhibin derived from the ovary plays a far greater role in the negative control of FSH. In addition, bone morphogenic proteins, BMP-6 and BMP-7, are capable of modulating FSH synthesis in gonadotropes, suggesting that other systems may also be involved in the control of FSH.
Follistatin is a monomeric protein that is distinct from the activin and inhibin family and acts as a virtually irreversible binding protein by complexing with activin and masking the binding sites on activin for the type I and type II receptors. Synthesis of follistatin in pituitary folliculostellate cells and gonadotropes is controlled by activin and GnRH and may be additionally modulated by gonadal steroids. The follistatin 288 isoform (FS288) has a high affinity for cell-surface proteoglycans and is presumed to act within the pituitary. Activin synthesis does not appear to vary during female reproductive cycles. However, changes in pituitary follistatin mRNA have been noted during the rat estrus cycle, suggesting that the effects of activin on FSH synthesis and secretion may be modulated through changes in follistatin. As indicated earlier, follistatin is increased by fast frequencies of GnRH stimulation and decreased with slower frequencies, compatible with the hypothesis that the activin and follistatin system mediates the effect of GnRH pulse frequency on FSH. Thus, although GnRH appears to be sufficient for control of LH synthesis and secretion, the activin-inhibin-follistatin system and perhaps BMPs play a significant role in conjunction with GnRH in regulating the synthesis and secretion of FSH.
Ovarian Feedback on the Hypothalamus and Pituitary
Negative Feedback
Estrogen
It is well known that low doses of estrogen inhibit gonadotropin secretion. Increased gonadotropin levels in patients with aromatase deficiency provide the most specific evidence of estrogen negative feedback on the control of both LH and FSH in men and women. This is supported by the marked increase in LH and FSH levels in postmenopausal and ovariectomized women. The loss of gonadal feedback in ovariectomized rats is associated with an increase in LH and FSH secretion; increased expression of LHβ, FSHβ, and α-mRNA; an increase in the number of cells expressing LHβ; an increase in cell size; and an increase in the total expression per cell. Administration of low doses of estradiol reverses these changes, as has also been shown in the ovariectomized sheep and monkey.
There is considerable evidence supporting a primary hypothalamic site of estrogen negative feedback on pituitary gonadotropin secretion. Studies, in which GnRH secretion has been measured, using a push-pull hypothalamic perifusion technique in the rat or cannulation of the pituitary portal circulation in the sheep and monkey, indicate that GnRH increases following ovariectomy and decreases with estrogen replacement. Estradiol administration is associated with a decrease in GnRH expression in rat hypothalamic tissue slices and in a GnRH neuronal cell line. Studies in estrogen receptor (ER) knockout animals support an essential role for ERα in estrogen negative feedback, although both forms of the receptor are present in the hypothalamus. The major hypothalamic estrogen negative feedback effect is mediated through neurons upstream of GnRH itself, most importantly the KNDy neurons of the arcuate/median eminence. Estrogen-receptive GABA neurons within the preoptic area may also play a role in mediating the negative feedback effects of estrogen on GnRH secretion. Although involved in progesterone negative feedback at the hypothalamus, the opioid system is unlikely to be involved in estrogen negative feedback.
In women, the mechanisms underlying estrogen negative feedback have generally been investigated in postmenopausal or ovariectomized women. The quantity of GnRH, estimated in vivo using submaximal GnRH antagonist administration, is higher in postmenopausal compared with premenopausal women, consistent with the increase in GnRH mRNA that has been demonstrated in postmenopausal women studied at autopsy. With administration of low levels of estradiol, the quantity of GnRH returned to follicular-phase levels in postmenopausal women, suggesting that the increase in GnRH in the absence of gonadal feedback can be attributed entirely to loss of estrogen. To further support a hypothalamic site of estrogen negative feedback in women, neuroimaging studies have now provided evidence of decreased metabolic activity in the medial basal hypothalamus in association with brief exposure to relatively low doses of estradiol in postmenopausal women. Estradiol administration does not decrease GnRH pulse frequency in the majority of studies in postmenopausal women ; thus the negative feedback effect of estradiol on the hypothalamus is likely to be mediated through changes in GnRH pulse amplitude. These results in women are similar to those in which GnRH was measured directly in pituitary portal blood in ovariectomized sheep and monkeys, showing a decrease in GnRH pulse amplitude but not frequency in response to estradiol administration. Autopsy studies in women are consistent with a role for neurons expressing kisspeptin, NKB, substance P, dynorphin, and ERα in mediating the negative feedback of estrogen in the medial basal hypothalamus.
Although the studies detailed here demonstrate a significant hypothalamic effect of estrogen negative feedback, they do not exclude a pituitary site of action. In cultured pituitary cells, estradiol transiently reduces the LH response to GnRH, and administration of estradiol to hypothalamic-lesioned monkeys receiving pulsatile GnRH decreased LH secretion. Both ERα and ERβ are present on gonadotropes, and characterization of the gonadotrope specific ERα (ESR1) knockout mouse has now provided definitive evidence of a direct inhibitory effect of estradiol at the pituitary in the rodent. Recent studies in postmenopausal women that isolated the pituitary from hypothalamic input have extended these findings from lower animal species to the human by demonstrating that physiologic levels of estradiol have a direct pituitary effect on gonadotropin secretion that is greater for FSH than for LH.
Progesterone
Progesterone has a profound effect on gonadotropin secretion that manifests at the hypothalamic level through slowing of pulsatile GnRH secretion. This effect requires estrogen priming, likely through upregulation of progesterone receptors in the hypothalamus. In postmenopausal women receiving low doses of estradiol, addition of progesterone uniformly suppresses GnRH pulse frequency using either LH or FAS as markers of GnRH secretion, and administration of progesterone decreases the overall amount of GnRH secretion. It is likely that progesterone exerts its effects on GnRH secretion through both direct and indirect mechanisms. As indicated earlier, there is ample evidence that the β-endorphin system plays a critical role in mediating the effects of progesterone on GnRH pulse frequency, and studies in sheep indicate that the inhibitory effect of progesterone on GnRH secretion is mediated by dynorphin.
Inhibin A and Inhibin B
Evidence for a nonsteroidal gonadal factor with feedback effects on the pituitary dates to the early 1900s, but it was not until the mid-1980s that inhibin was isolated and subsequently found to be part of a family of peptides that includes inhibin A, inhibin B, activin, and the functionally related protein follistatin. Inhibin B is present in the circulation in men, while both inhibin A and inhibin B are detected in serum and follicular fluid in women during their reproductive years.
During folliculogenesis, peak levels of inhibin A are attained in the preovulatory period and inhibin A is correlated with the size of the dominant follicle in normal cycles, as is estradiol. Follicular-phase inhibin A is primarily the product of granulosa cells; however, there is some evidence for theca cell production in the mature follicle. Studies of βA inhibin subunit expression and measurement of dimeric inhibin A in follicular fluid are consistent in indicating maximal follicular-phase levels in the preovulatory follicle. However, it is now appreciated that inhibin A is synthesized and secreted at earlier stages of follicular development than previously suggested by serum levels. The peak in inhibin A in the luteal phase and its decline with luteolysis are consistent with production by the corpus luteum, as expected from the high levels of βA inhibin subunit expression in the corpus luteum.
In contrast to inhibin A, the pattern of inhibin B in serum suggests that it is primarily secreted from small antral follicles. A correlation of inhibin B levels with the size of the dominant follicle is remarkably absent in women undergoing spontaneous ovulatory cycles, suggesting that inhibin B is not regulated in relation to dominant follicle growth. These data are consistent with studies showing that inhibin B levels in follicular fluid do not change as a function of follicle size or maturity. They are also consistent with earlier studies demonstrating that expression of βB inhibin subunit mRNA is highest in early antral follicles, with lower levels in the dominant follicle and no evidence of βB-subunit expression in the corpus luteum. The latter studies have also shown that inhibin B synthesis is confined to the granulosa cells and is absent in theca cells.
Regulation of Inhibin A and Inhibin B by Gonadotropins.
A significant body of data has demonstrated an increase in inhibin B secretion in conjunction with early follicular development stimulated by physiologic levels of FSH. This was initially suggested by the relationship of the rise in FSH during the luteal-follicular transition to the concomitant increase in inhibin B. In GnRH-deficient women, replacement of pulsatile GnRH at the slower luteal-phase frequency of every 4 hours compared with the early follicular-phase frequency of every 90 minutes resulted not only in failure of FSH to rise normally during the luteal-follicular transition but also in the absence of both follicular growth and any increase in secretion of inhibin B ( Fig. 7.5 ). This study demonstrated the remarkable sensitivity of serum inhibin B levels to changes in FSH stimulation within the physiologic range during the earliest stages of follicular development.

In studies designed to examine the response to differential gonadotropin stimulation at different stages of follicle development, recombinant human LH (rhLH) or FSH (rhFSH) was administered to women following downregulation of endogenous GnRH secretion using a potent GnRH agonist. Consistent with failure to observe LH receptors in small antral follicles, rhLH alone had no effect on follicle growth or hormone secretion when administered daily for up to 7 days. However, daily subcutaneous administration of rhFSH resulted in normal early follicular-phase levels of FSH and an increase in inhibin B secretion that preceded that of estradiol and inhibin A ( Fig. 7.6 ). One interpretation of these studies is that FSH directly stimulates inhibin B secretion from granulosa cells. However, administration of FSH at this stage of development also results in the recruitment of a cohort of follicles into the growing pool and a dramatic stimulation of granulosa cell number.

To determine whether the increase in inhibin B observed in vivo in the early stages of folliculogenesis results from direct stimulation of granulosa cell secretion or from an increase in granulosa cell number, studies were conducted using preantral and small antral follicles obtained from ovaries at the time of oophorectomy for nonovarian indications. These studies indicated that inhibin B is constitutively secreted from preantral follicles; FSH stimulates secretion of inhibin A from granulosa cells, but this is not the case for inhibin B. Taken together, these studies demonstrate that inhibin B is constitutively secreted from granulosa cells and that the increase in inhibin B observed in vivo in response to FSH results from an increased number of granulosa cells rather than direct stimulation of secretion by FSH. At later stages of follicular development, both LH and FSH stimulate secretion of inhibin A and estradiol from the dominant follicle, but have no effect on inhibin B.
Evidence for an Endocrine Negative Feedback Role for Inhibin A or Inhibin B.
Inhibin subunits are expressed in a variety of tissues including the adrenal. However, the only significant source of circulating dimeric inhibins is the gonads, and there is compelling evidence that the principal mechanism of action of inhibin in suppressing pituitary FSH secretion is an endocrine action. Although an endocrine role of either inhibin A or inhibin B cannot be implied from their relationships to FSH during the normal menstrual cycle, several lines of evidence indicate that the inhibins do play a role in the negative feedback regulation of FSH. Inhibin was initially discovered based on its ability to inhibit FSH secretion in pituitary cell cultures. In addition, FSH levels decrease in response to administration of pharmacologic doses of inhibin A administered in the follicular and luteal phases in the rhesus monkey. The most compelling line of evidence that inhibin regulates FSH secretion under normal physiologic circumstances in women is the failure of physiologic levels of gonadal steroids to restore FSH levels to normal in postmenopausal women. The model of reproductive aging has been used by a number of investigators to refine this evidence. FSH levels increase with age, before increases in LH or decreases in estradiol, and a number of studies have demonstrated an inverse relationship between increasing FSH and decreasing inhibin B in association with reproductive aging.
Reproductive aging is associated with a decline in fertility that begins in the third decade but accelerates rapidly after age 35. A gradual decrease in the pool of ovarian follicles, which first accelerates around the same age, would appear to underlie this decline in fertility. It is also at age 35 that an increase in follicular-phase FSH is first seen. In women 35 years of age or older with regular ovulatory cycles and follicular-phase FSH levels within the normal range, there is a small but significant increase in FSH in the early follicular phase only, decreased inhibin B levels across the entire follicular phase, and estradiol levels that do not differ in the early follicular phase but increase in the midfollicular and late follicular phases. In the luteal phase, inhibin B, inhibin A, and progesterone are lower in older cycling women, whereas estradiol levels are preserved. Thus the very earliest decrease in inhibin B occurs at a time that is marked by the beginning of the more rapid follicular depletion that heralds the menopause, suggesting that lower inhibin B levels reflect a decrease in available follicle number with aging. This change in inhibin B is associated with higher levels of FSH in the absence of alterations in estradiol, providing evidence for an endocrine role of inhibin B in the control of FSH secretion in reproductive-aged women. Studies conducted at later stages in the process of reproductive aging have uniformly confirmed increased FSH in conjunction with normal to higher levels of estradiol and lower inhibin B and further support the negative feedback role of inhibin B in control of FSH. Although a decrease in inhibin A may occur slightly later in reproductive aging, it precedes the decline in estradiol levels.
Whereas studies of reproductive aging provide evidence of a negative feedback role of inhibin B on FSH secretion in normal women, these studies have not clarified the role of inhibin A or inhibin B relative to that of estradiol in the dynamic control of FSH during normal reproductive cycles. A key question is whether inhibin A and inhibin B contribute to the midfollicular decrease in FSH that is critical to the monofollicular development that characterizes normal reproductive cycles in women. The tools to determine the role of inhibin directly by either administration or blockade are not available. However, it is possible to investigate the estrogen component of FSH negative feedback and thereby infer the physiologic role of inhibin. Studies in which estradiol levels were maintained by estradiol administration during the luteal-follicular transition have been interpreted to suggest that inhibin A is not involved in the negative feedback control of FSH in the luteal phase. An alternative approach is to block estrogen negative feedback. The use of tamoxifen to block the ER permitted evaluation of the relative role of estradiol and the inhibins in negative feedback on FSH. Results indicate that the low levels of estradiol in the early follicular phase contribute to negative feedback on FSH, mediated at a hypothalamic level as shown by the absence of such an effect in GnRH-deficient women receiving physiologic GnRH replacement. However, FSH failed to approach menopausal levels, indicating that inhibin B is also an important factor in FSH regulation during the normal cycle. These studies also provided evidence that both estradiol and inhibin A are required for FSH restraint during the luteal phase.
Activin/Follistatin
The control of FSH is dependent not only on inhibin and estradiol but also on the activin/follistatin system. Activin acts as a local growth and differentiation factor in the ovary as well as the pituitary. During the normal menstrual cycle, total activin A levels are highest at the midcycle and during the luteal follicular transition. However, there is no change in activin A in follicular fluid as a function of follicular development, no change in free activin across the menstrual cycle, and no difference in activin B between the follicular and luteal phases. Furthermore, a potential endocrine role of activin can only be considered in the context of follistatin, which is synthesized in many tissues as well as the pituitary. While activin has been measured in serum, circulating activin is irreversibly bound by the circulating isoform of follistatin, FS315. To date, no mechanisms have been identified within tissues that would alter neutralization by follistatin and therefore it is almost certain that activin acts in an autocrine and paracrine, but not an endocrine fashion, to regulate FSH secretion.
Gonadotropin Surge Attenuating Factor
Gonadotropin surge attenuating factor (GnSAF), also known as gonadotropin surge inhibiting factor (GnSIF), is an ovarian factor that reduces GnRH-induced LH secretion. Despite many years of investigation, the molecular structure of GnSAF has not been completely characterized, hampering efforts to fully understand its regulation and physiologic role. Its name derives from the initially hypothesized role of this compound in preventing an early LH surge and resulting in premature luteinization of the preovulatory follicle. However, there is now evidence in animal models and in women that there is an inverse relationship between GnSAF bioactivity and follicle size, with the highest concentrations in small growing follicles, suggesting that its primary role may be at earlier stages of follicle development.
Positive Feedback
Estrogen
In addition to inhibition of gonadotropin secretion, estrogen exerts a stimulatory effect to generate the preovulatory LH surge. This positive feedback effect is seen in multiple animal species and in women. There are two critical questions that form the basis of a mechanistic understanding of gonadotropin surge generation: the first is how estrogen can exert both inhibitory and stimulatory effects on LH secretion, and the second is whether the site of estrogen positive feedback is at the pituitary, the hypothalamus, or both. The direction of estrogen feedback is dependent on both the degree of estrogen exposure and its duration with low levels of estradiol administration resulting in decreased LH secretion within 12 to 24 hours, while positive feedback requires exposure to higher concentrations over a more prolonged duration. There is ample evidence that high levels of estrogen augment the pituitary response to GnRH across species. Studies in animal models demonstrate an increase in the number of cells expressing the gonadotropin subunits, an increase in GnRH receptor number, an impact on the function of ion channels in the plasma membrane, and regulation of both gene expression and second messenger systems within gonadotropes. Studies in LβT2 pituitary cells indicate that activin may act in concert with estrogen to increase GnRH receptors on pituitary gonadotropes, while NPY secretion from the median eminence may contribute to pituitary sensitization through changes in the affinity of the GnRH receptor for its ligand.
Progesterone
In addition to its predominant effect on slowing of the frequency of GnRH secretion, progesterone has both indirect and direct effects at the pituitary that result in an increase in LH. Evidence for an indirect pituitary effect of progesterone comes from studies in individuals with GnRH deficiency in whom the frequency of pulsatile GnRH administration is inversely associated with LH pulse amplitude such that LH pulse amplitude increases with a longer duration from the previous pulse. Studies in GnRH-deficient women receiving pulsatile GnRH with or without progesterone indicate that progesterone also increases LH pulse amplitude through a direct pituitary action that is independent of changes in pulse frequency.
Inhibin A
Inhibin A is elevated in women before ovulation, and there are several lines of evidence suggesting that inhibin A may play a role in positive feedback at the pituitary level. Inhibin A increases GnRH receptors by threefold to sixfold in pituitary cell cultures and this is also true in vivo in the sheep. The effects of estradiol and inhibin A on GnRH receptors are additive. The effect of inhibin A on the percentage of LH-positive cells and the percentage of LH positive cells that bind GnRH is greater than the effect of estradiol; however, inhibin A has a less pronounced effect than estradiol on positive feedback effects downstream of the LH receptor.
Kisspeptin
An emerging body of data suggests that there may be a pituitary role for kisspeptin in addition to its well-studied hypothalamic role. Kisspeptin has been found in the pituitary portal system in rodents and sheep, although it does not change dynamically, suggesting that kisspeptin from the hypothalamus may not be involved in pituitary regulation. However, Kiss1 and Kiss1r are expressed in gonadotropes and other pituitary cell types in rodents, and expression of Kiss1 is upregulated at the level of the gonadotrope by a direct action of estrogen acting through the ERα receptor. Kisspeptin induces transcription of LHβ and FSHβ gene expression in LβT2 cells and increases GnRHR expression in this same cell type. Kissr1 is enhanced in female mice during the estradiol-induced LH surge, possibly through the effect of the increased secretion of GnRH at midcycle in rodents and its stimulatory effect on kissr1. Although kisspeptin positive cells were demonstrated in the anterior pituitary in the monkey, they were not shown to be colocalized to the gonadotrope, pointing to potential species differences. KISS1R is present in the human pituitary ; further information is not available to determine whether alterations may contribute to pituitary sensitization to GnRH in the setting of high estradiol levels at the time of the MCS.
Species Differences in Hypothalamic Input
In the rat and sheep, estrogen positive feedback on gonadotropin secretion requires an increase in GnRH secretion in addition to pituitary augmentation of the GnRH signal. In rodents, this increase in GnRH secretion requires specific circadian signals. Also in rodents, estrogen negative feedback occurs in the arcuate nucleus of the medial basal hypothalamus and kisspeptin neurons in the region coexpress NKB and dynorphin. In contrast, positive feedback occurs in the AVPV, where kisspeptin neurons do not express NKB and dynorphin.
Generation of the preovulatory surge in nonhuman primates appears to be fundamentally different from that in the rodent; while there may be some alteration in GnRH input, this is not controlled in the preoptic area (the site of the AVPV), it is not tied to circadian signals, and the gonadotropin surge does not require an increase in GnRH. As in nonhuman primates, an increase in GnRH secretion is not required for generation of a normal gonadotropin surge in women ; moreover, there is no evidence for augmentation of GnRH secretion or even an altered pattern of GnRH stimulation associated with generation of the surge in women. Taken together, these data suggest that the gonadotropin surge in normal women requires ongoing pulsatile GnRH stimulation but is otherwise mediated through the marked increase in pituitary sensitivity to GnRH (discussed later in MCS). Thus, although an increase in GnRH is required for generation of the gonadotropin surge in rodents and sheep, GnRH appears to play a permissive role in generation of the preovulatory LH surge in normal women, as it does in the monkey.
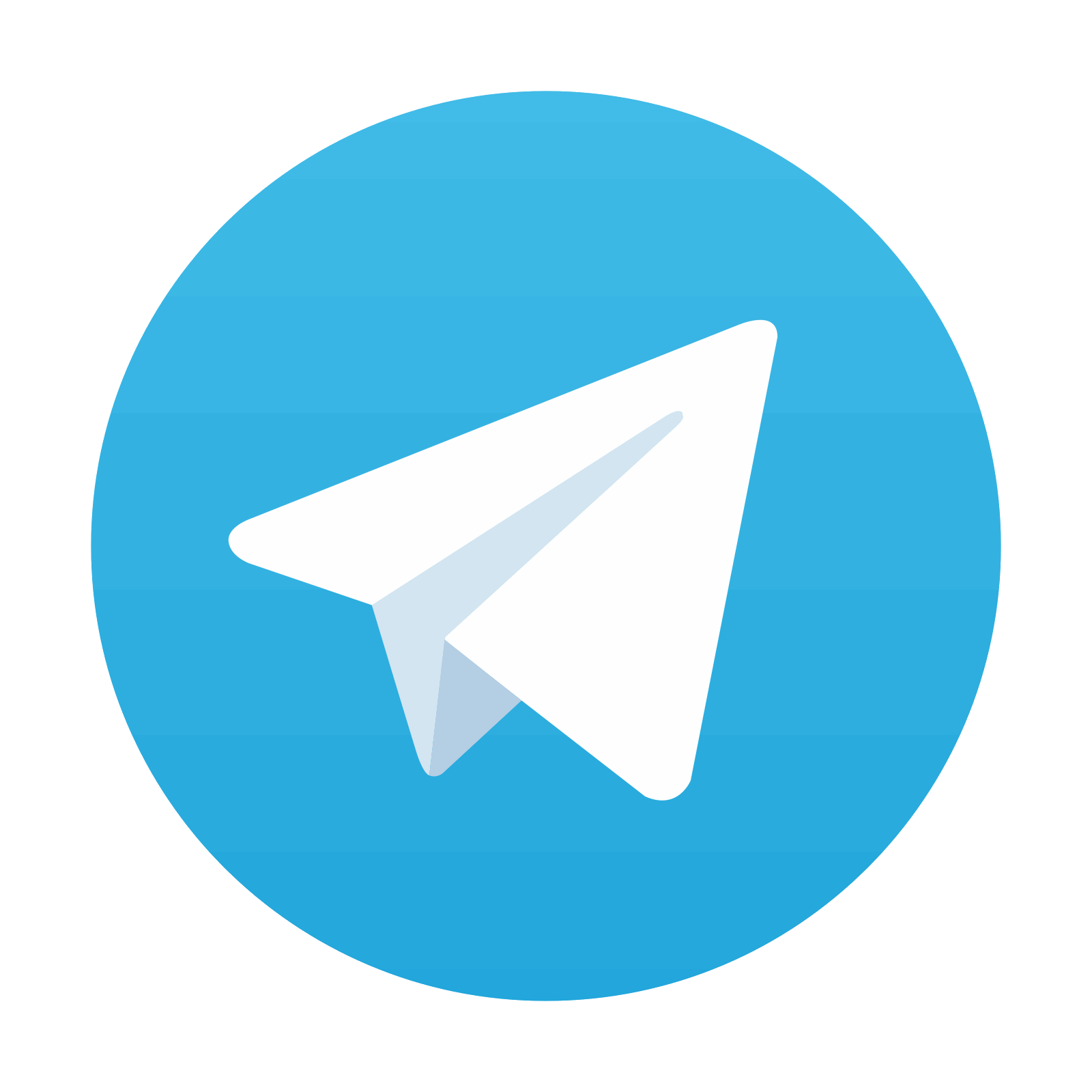
Stay updated, free articles. Join our Telegram channel

Full access? Get Clinical Tree
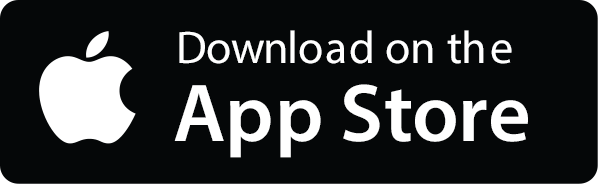
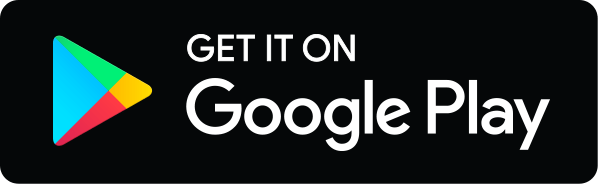