Nasopharynx
ANATOMY
The nasopharynx is a cuboidal chamber that is slightly broader in the transverse dimension than in the anterior–posterior dimension (Fig. 41.1). Anteriorly it is continuous with the nasal cavity via the posterior choanae, while inferiorly it communicates with the oropharynx. The roof of the nasopharynx is formed by the basilar portion of the sphenoid and occipital bones and the floor by the superior surface of the soft palate and nasopharyngeal isthmus. The lateral walls of the nasopharynx contain the pharyngotympanic tube (Eustachian tube) openings, which are bounded by a prominence known as the torus tubarius. The torus is formed by the cartilage of the pharyngotympanic tube elevating the mucous membrane of the lateral nasopharynx. Posterior to the torus is the pharyngeal recess otherwise known as the fossa of Rosenmüller. The lateral walls, including the pharyngeal recess (fossa of Rosenmüller), are the most common origin of nasopharyngeal malignancies. The posterior wall of the nasopharynx contains the superior pharyngeal constrictor muscle, pharyngobasilar fascia, and buccopharyngeal fascia.
The superior pharyngeal constrictor only extends superiorly to the skull base in the midline, and laterally the pharyngobasilar fascia serves to attach the constrictor muscle to the base of the skull at the basiocciput and petrous portion of the temporal bone. This lateral area of muscular deficiency is otherwise known as the sinus of Morgagni, through which the pharyngotympanic tube and levator veli palatini pass. The pharyngobasilar fascia is continuous with the foramen lacerum and is in close proximity to the foramen ovale, foramen spinosum, jugular foramen, hypoglossal canal, and carotid space. The proximity of these foramina to the sinus of Morgagni assumes importance in the consideration of intracranial extension (Fig. 41.2). A summary of the various foramina located in the base of skull is presented in Table 41.1.
The afferent innervation of the nasopharynx anterior to the pharyngotympanic tube orifice is provided by the maxillary division of the trigeminal nerve (V2), and posterior to the tubal orifice by the glossopharyngeal nerve. Motor supply is via the pharyngeal branches of the glossopharyngeal nerve, vagus nerve, and sympathetic fibers from the superior cervical ganglion. The arterial supply of the nasopharynx is provided by the ascending pharyngeal artery, sphenopalatine artery, and the artery of the pterygoid canal. Venous drainage is provided by the pharyngeal plexus, which drains into the internal jugular veins directly or via communication with the pterygoid plexus.
FIGURE 41.1. A: Midsagittal magnetic resonance image (MRI) of the head, showing the nasopharynx and related structures. B: Axial contrast-enhanced MRI showing a small tumor in the left fossa of Rosenmüller (arrow) and normal structures in the rest of the nasopharynx.
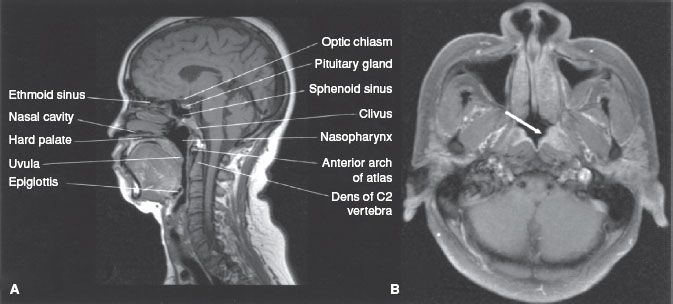
EPIDEMIOLOGY AND ETIOLOGY
Nasopharyngeal carcinoma is an uncommon cancer in most parts of the world. The age-adjusted incidence rate (per 100,000 people per year) among men ranges from 0.6 in the United States and Japan to 5.4 in Algeria, 5.8 in the Philippines, 11.0 in Singapore, 17.2 among Eskimos, Indians, and Aleuts in Alaska to 17.8 and 26.9 in Hong Kong and Guangdong Province in Southern China, respectively.1–2,3
A bimodal age distribution is observed in low-risk populations. The first peak incidence arises between 15 to 25 years of age, with the second peak at 50 to 59 years of age.4,5–6 In high-risk populations, the peak incidence occurs in the fourth and fifth decades of life.4 Both genders have a similar age distribution; however, the male-to-female incidence ratio is 2:1 to 3:1.7
This distinct racial and geographic distribution of nasopharyngeal carcinoma suggests a multifactorial cause. Current epidemiologic and experimental data identify at least three important etiologic factors: (i) genetic, (ii) environmental, and (iii) viral.
The high incidence of nasopharyngeal carcinoma among Southern Chinese and populations of Southern Chinese descent suggests a component of genetic susceptibility. A genome-wide association study of nasopharyngeal cancer found three susceptibility loci8 and confirmed a linkage study that found a gene closely linked to the HLA locus conferred a greatly increased risk of this disease.9 In addition, several HLA haplotypes, including A2, B46, and B17, are associated with an increased risk of developing nasopharyngeal carcinoma.10,11
The high consumption of salted fish in Southern China has been implicated as an important environmental factor.12–13,14–15 Dimethylnitrosamine, a carcinogen found in salted fish, has been shown to induce carcinoma in the upper respiratory tract in rats.13 Other potential environmental etiologic factors that have been associated with nasopharyngeal carcinoma include alcohol consumption and exposure to dust, fumes, formaldehyde, and cigarette smoke,16,17 although definitive conclusion has been elusive. Descendents from Chinese who have migrated from endemic areas to Western countries show progressively lower risk, but their incidence remains higher than that of the indigenous populations.18–20 Buell18 observed that American-born second-generation Chinese had a lower risk than the Asian-born first generation, while whites born in Southeast Asia had an increased risk compared to American-born whites. Dickson and Flores20 reported that the incidence rate in Chinese who were natively born in China was 20.5, compared with 1.3 for Chinese and 0.2 for whites born in Canada. Taken together, environmental factors appear to play a role in the etiology of nasopharyngeal cancer.
Epstein-Barr virus (EBV) has been associated with nasopharyngeal carcinoma, especially the nonkeratinizing type, irrespectively of ethnic or geographic origin.21 Premalignant lesions of nasopharyngeal epithelium show increased levels of EBV, suggesting that EBV infection may influence the early stages of tumorigenesis in nasopharyngeal carcinoma (NPC).22 Detection of a single form of EBV DNA in tumors suggests that clonal expansion from an initial EBV infected and ultimately transformed cell is likely. EBV’s tumorigenic potential is due to a set of latent genes: latent membrane proteins (LMP1, LMP2A, and LMP2B) and EBV-determined nuclear antigens (EBNA1 and EBNA2), which are the proteins predominantly expressed in NPC.23 LMP1 is the principal oncogene, with evidence that the C-terminal activating regions of the protein activate a variety of signaling pathways, including mitogen-activated protein kinases, phosphoionositol-3-kinase, nuclear factor κ-B, and epidermal growth factor receptor (EGFR).24,25 LMP1 is also required for cell immortalization and is present in 80% to 90% of NPC tumors.26 This mounting evidence highlights the likely etiologic role for EBV in NPC.
FIGURE 41.2. A: Basal view of skull illustrating the foramina of the base of the skull and the occupying structures. B: Axial computed tomography scan illustrating the bony anatomy.
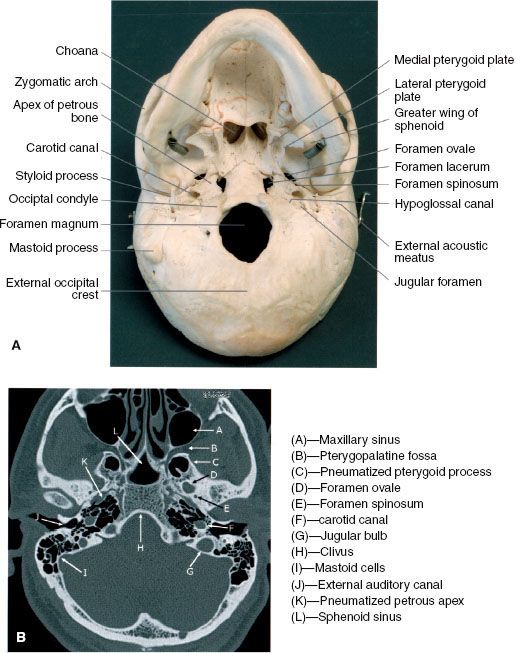
FIGURE 41.3. Coronal section through the sphenoid sinus and roof of the nasopharynx showing the relative positions of the cranial nerves III to VI. (Modified from Chao KSC. Practical essentials of intensity-modulated radiation therapy. Philadelphia: Lippincott Williams & Wilkins; 2005:138.)
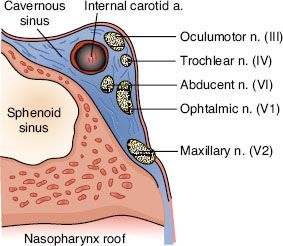
TABLE 41.1 FORAMINA OF THE BASE OF THE SKULL AND ASSOCIATED ANATOMIC STRUCTURES
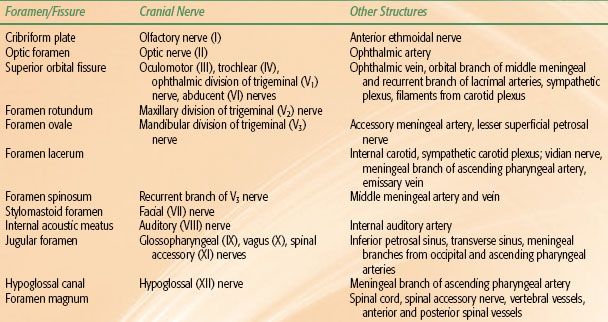
NATURAL HISTORY
Local Extension
A summary of structures locally infiltrated by nasopharyngeal carcinoma at diagnosis is provided in Table 41.2.
Anterior
Anteriorly, it is common for the extension and infiltration of tumor to occur into the nasal fossa. Invasion of the lateral wall of the nasal fossa can lead to involvement and destruction of the pterygoid plates. Beyond these structures, albeit less common, is invasion of the posterior ethmoid and maxillary sinuses. In advanced disease, infiltration of the orbital apex (typically through the inferior orbital fissure) can occur.
Superior and Posterior
Superiorly, tumors can directly invade the base of skull, sphenoid sinus, and the clivus. The foramen lacerum, positioned directly above the pharyngeal recess (fossa of Rosenmüller), is a vulnerable spot through which tumor may enter the cavernous sinus and the middle cranial fossa to invade cranial nerves II to VI (Fig. 41.3). Figure 41.4 demonstrates involvement of the trigeminal cave (Meckel’s cave) and the maxillary branch of the trigeminal nerve (V2). The foramen ovale also allows access for tumor to invade the middle cranial fossa, in addition to the petrous portion of the temporal bone, and the cavernous sinus. Posteriorly, invasion of the prevertebral (longus capitus) muscles is commonly seen.
Inferior
Extension inferiorly to the oropharynx is not unlikely, with potential involvement of the tonsillar pillars, the tonsillar fossa, and the lateral and posterior oropharyngeal walls. In advanced disease, invasion of the C1 vertebra posteriorly and inferiorly can occur. Direct invasion of the soft palate is uncommon.
FIGURE 41.4. A: Axial T1-weighted magnetic resonance image (MRI) demonstrating involvement of the maxillary branch of the trigeminal nerve by nasopharyngeal carcinoma (V2) (arrow). B: Coronal contrast-enhanced MRI showing involvement of the trigeminal cave (also known as Meckel’s cave) by nasopharyngeal carcinoma (arrow). C: Coronal contrast-enhanced MRI showing involvement of the maxillary branch of the trigeminal nerve by nasopharyngeal carcinoma (V2) (arrow).
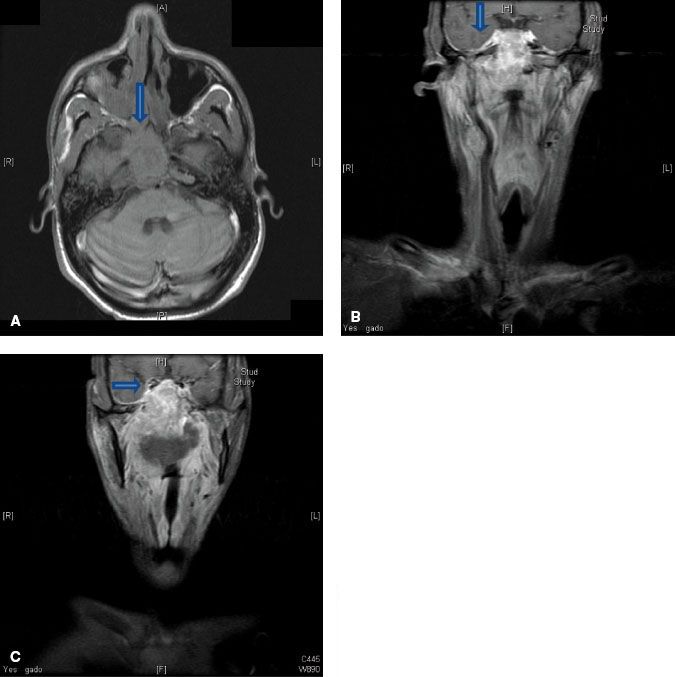
FIGURE 41.5. A: Axial T1-weighted magnetic resonance image (MRI) showing tumor infiltration of the right parapharyngeal space (left arrow). Note the resultant serous otitis media (right arrow). B: Axial contrast-enhanced MRI showing enhanced tumor involving the parapharyngeal space and medial pterygoid muscles (arrow).
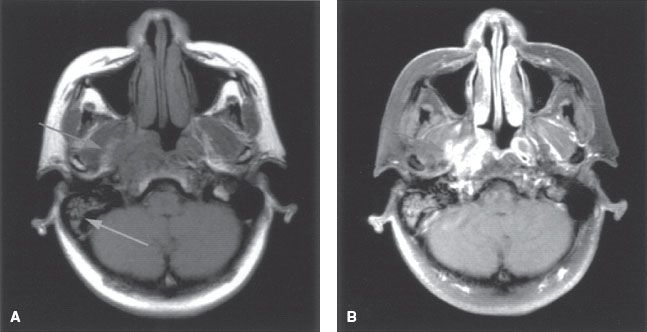
FIGURE 41.6. A: Axial contrast-enhanced magnetic resonance image (MRI) demonstrating involvement of the cavernous sinus by nasopharyngeal carcinoma. B: Axial contrast-enhanced MRI showing invasion of pterygopalatine fossa (vertical arrow) with spread to cavernous sinus (horizontal arrow).
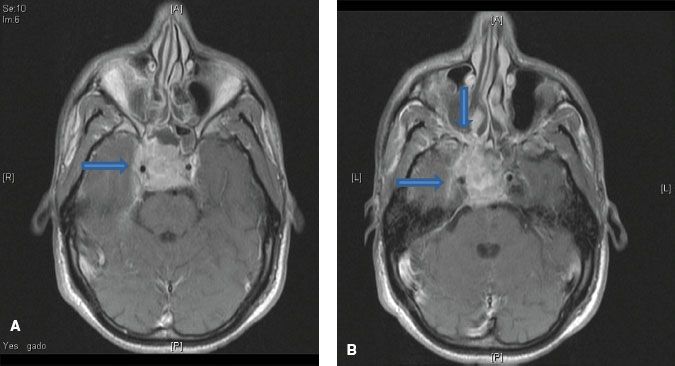
FIGURE 41.7. A: Pathways for lymphatic spread of nasopharyngeal carcinoma. B: Two major lymph collectors of the nasopharynx: (i) lateral lymph collector and (ii) posterior lymph collector. Yellow, lymphatic vessels from nasal cavity. Blue, lymphatic vessels from soft palate. Green, lymph nodes. Retropharyngeal nodes are numbered. Red, carotid artery. Dark brown, longus muscles. Light brown, remaining pharyngeal and nasal wall. (A, Redrawn from Rouviere H. Anatomy of the human lymphatic system. Ann Arbor, MI: Edward Brothers; 1938:27. B, From Pan WR, Suami H, Corlett RJ, et al. Lymphatic drainage of the nasal fossae and nasopharynx: Preliminary anatomical and radiological study with clinical implications. Head Neck 2009;31:52–57, with permission.)
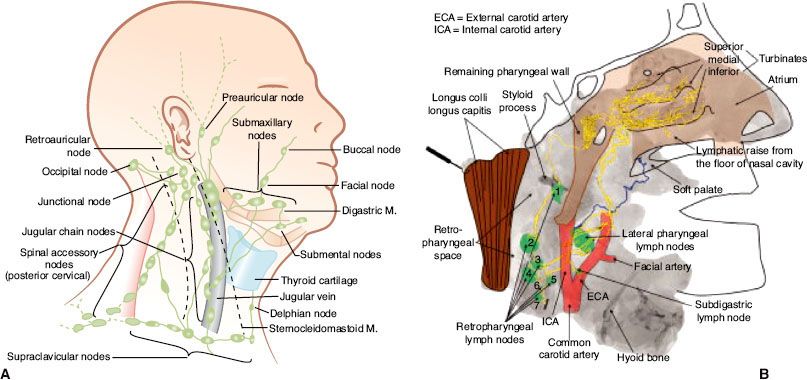
FIGURE 41.8. Distribution of positive nodes at different radiologic levels based on magnetic resonance imaging of 202 patients with nasopharyngeal carcinoma treated at Pamela Youde Nethersole Eastern Hospital (Hong Kong). Note the anatomic boundaries of the supraclavicular fossa as defined by the American Joint Committee on Cancer/International Union Against Cancer staging system.
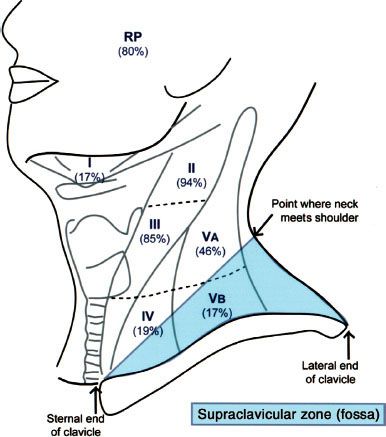
Lateral
Lateral extension occurs early, with involvement of the lateral parapharyngeal space along with invasion of the levator and tensor veli palatini muscles (Fig. 41.5). In advanced disease, invasion of the pterygoid muscles can occur. Direct extension of the tumor or lateral retropharyngeal lymph node metastasis in the parapharyngeal space may lead to invasion or compression of cranial nerves IX to XI as they transpire the jugular foramen, cranial nerve XII as it emerges from the hypoglossal canal, and the cervical sympathetic nerves. Direct invasion or compression of the internal carotid artery can occur in advanced disease (Fig. 41.6). Tumor can directly invade the middle ear through the pharyngotympanic tube (Eustachian tube).
Lymphatic Spread
The nasopharynx is comprised of a vast avalvular lymph capillary network that exists in the mucous membrane, leading to frequent involvement of regional neck nodes (Fig. 41.7). As many as 85% to 90% of cases present with lymphatic spread to the ipsilateral nodes.27–29 Bilateral spread is present in approximately 50% of cases. The distribution of NPC involved nodes at diagnosis is shown in Figure 41.8. An anatomic and radiographic study that directly examined lymphatic vasculature concluded that there are two major lymph collectors of the nasopharynx (Fig. 41.7B).30 One lymph collector runs along the lateral side of the pharyngeal wall, while the second runs more posteriorly. The lateral lymph collector empties into multiple first-tier nodes, which include the lateral pharyngeal node, the jugulodigastric/subdigastric node, and the third, fourth, and fifth nodes of the retropharyngeal group.30 The posterior lymph collector empties into the first node (node of Rouviere) of the retropharyngeal group (Fig. 41.9). This direct study is corroborated clinically by frequent observation of lateral and retropharyngeal lymph node involvement by magnetic resonance imaging (MRI) or computed tomography (CT) scans, even though they remain impalpable. Metastasis to the jugulodigastric and superior posterior cervical nodes is also common. From these first-tier nodes, further metastatic spread to the midjugular, lower jugular, and posterior cervical and supraclavicular nodes can develop. Seldom, submental and occipital nodes can be involved secondary to lymphatic obstruction caused by widespread cervical lymphadenopathy. Mediastinal lymph nodes and, occasionally, axillary nodes may be involved with the presence of supraclavicular lymphadenopathy.
Hematogenous Dissemination
Distant metastasis is present in 3% to 6% of the cases at presentation and may occur in 18% to 50% of cases during the disease course.31,32,33–34,35 The rate of distant metastasis is highest in patients with advanced neck node metastasis,27,36,37 especially with low-neck involvement.38,39 Bone is the most common distant metastatic site, followed by the lungs and liver,40 with lung metastasis being associated with better prognosis than other sites. Brain and skin metastases rarely occur.41,42
FIGURE 41.9. A: Axial contrast-enhanced computed tomography scan showing involvement of bilateral retropharyngeal lymph nodes (arrows) by nasopharyngeal carcinoma. B: Axial T2-weighted magnetic resonance image showing involvement of bilateral retropharyngeal lymph nodes (arrows) by nasopharyngeal carcinoma.
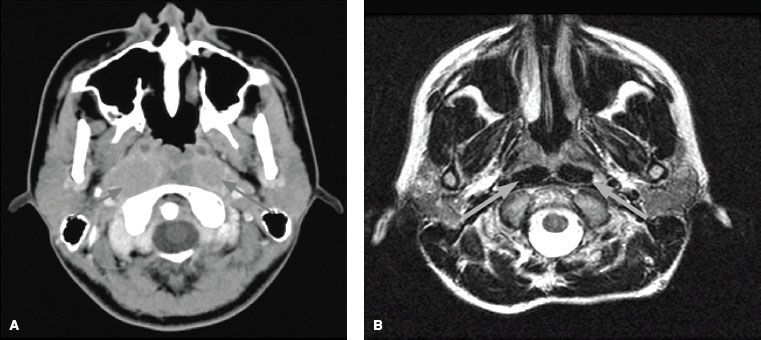
CLINICAL PRESENTATION
Nasopharyngeal carcinoma presents in patients with symptoms in one or more of the following three categories: (i) neck masses, usually appearing in the upper neck; (ii) presence of tumor mass in the nasopharynx (epistaxis, nasal obstruction and discharge); (iii) skull-base erosion and palsy of cranial nerves V and VI due to tumor extension superiorly (headache, diplopia, facial pain and numbness).
The frequency of various presenting symptoms and signs is summarized in Table 41.3. A neck mass is the most common presenting symptom, followed by nasal and aural symptoms. The physical signs commonly present at diagnosis are enlarged neck node(s) and, less often, cranial nerve palsy. The cranial nerves V and VI are frequently involved, while I, VII, and VIII are rarely involved (Table 41.4).29,43
Cervical lymphadenopathy is present in up to 87% of patients.44 Typically, a mass is observable in the upper posterior neck and palpable beneath the superior portion of the stemocleidomastoid muscle close to the mastoid process. This is caused by metastasis to the parapharyngeal nodes or superior posterior cervical nodes of the spinal accessory chain.
TABLE 41.2 STRUCTURES LOCALLY INFILTRATED BY NASOPHARYNGEAL CARCINOMA AT DIAGNOSISA
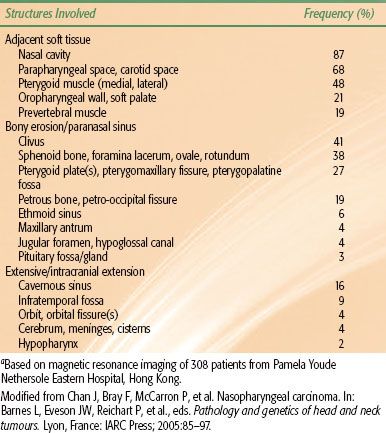
TABLE 41.3 SYMPTOMS AND PHYSICAL SIGNS OF NASOPHARYNGEAL CARCINOMA AT PRESENTATION
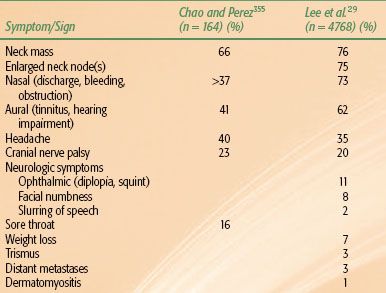
TABLE 41.4 INCIDENCE OF CRANIAL NERVE INVOLVEMENT BY NASOPHARYNGEAL CARCINOMA AT DIAGNOSIS
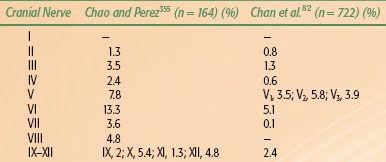
TABLE 41.5 RECOMMENDED PRETREATMENT DIAGNOSTIC EVALUATIONS FOR NASOPHARYNGEAL CARCINOMA
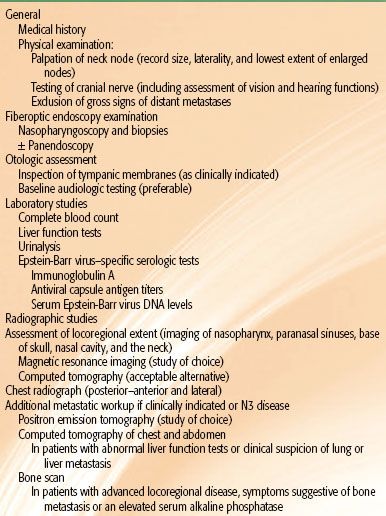
DIAGNOSTIC AND STAGING WORKUP
Diagnosis of nasopharyngeal carcinoma is made by biopsy of the primary tumor. This can typically be performed with local anesthesia in an outpatient setting. Biopsy by direct visualization with general anesthesia may be necessary for diagnosis when the tumor is not visible or when the patient cannot cooperate. Not uncommonly the tumor is submucosal and not visible. For suspicious cases of a nasopharyngeal primary tumor with lack of visible tumor, random biopsies of the most commonly involved sites are warranted: pharyngeal recess (fossa of Rosenmüller) on each of the lateral walls and superior posterior wall of the nasopharynx. Fine-needle aspiration of a suspicious neck mass may establish the presence of metastatic nasopharyngeal carcinoma in the regional lymphatics. This may be performed prior to the biopsy of the nasopharynx when the primary tumor is not clinically detectable.
Table 41.5 lists the pretreatment diagnostic evaluations and staging evaluations that are generally recommended for NPC.
Complete physical examination should include thorough palpation of the neck, cranial nerve examination, percussion and auscultation of the chest, palpation of the abdomen for possible liver involvement, and percussion of the spine and bones for possible bone metastasis. CT and MRI of the head and neck are useful in the evaluation of tumor erosion into the bony structures of the base of skull along with retropharyngeal and cervical lymphadenopathy. However, MRI is the preferred imaging technique in the staging evaluation of nasopharyngeal carcinoma.45–47 The current American Joint Committee on Cancer (AJCC) T-classification requires a search for tumor invasion into the soft tissue (e.g., parapharyngeal space) and bony structures. MRI may be necessary for proper staging because CT has limitations in accurately defining tumor extension into these regions.48 MRI is superior to CT in delineating muscle, soft tissue involvement, and examination of the skull base.48–50 When utilizing MRI, thin slices (3 mm) should be used for accurate staging (Fig. 41.10). Thicker slices (e.g., ≥5 mm) risk misdiagnosis of what may be a higher-T-stage disease.
Ng et al.51 compared MRI and CT in assessing extent of disease. The study found a significantly higher sensitivity of MRI for skull base involvement (60% vs. 40%), intracranial involvement (57% vs. 36%), retropharyngeal node (58% vs. 21%), and tumor infiltration of prevertebral muscles (i.e., longus colli muscles) (51% vs. 22%) compared to CT. By MRI, T-staging was modified in 27% of patients, with 22% being upstaged and 4% being downstaged.
MRI and CT scans can detect lymph node metastasis that may not be clinically evident on physical examination.52 According to a study by Van den Brekel et al.,53 lymph node metastases are commonly recommended to be radiologically defined by presence of central necrosis, extracapsular spread, shortest axial diameter ≥10 mm (11 mm for the juglodigastric node and 5 mm for the retropharyngeal node), or a cluster of three or more lymph nodes that are borderline in size.
Detailed evaluation of nodal enlargement by palpation and imaging should consist of the size and location of the node, unilateral/bilateral involvement, and assessment of supraclavicular fossa involvement. Figure 41.8 defines the anatomical boundaries of the supraclavicular fossa, and Figure 41.11 demonstrates an example of bilateral cervical lymph node involvement seen by radiologic studies.
A complete search for distant metastasis is recommended for patients with advanced logoregional disease (e.g., N3 disease) or patients with suspicious clinical or laboratory findings. Positron emission tomography (PET) CT scanning (Fig. 41.12) is now commonly utilized in place of conventional staging by CT, bone, scans and ultrasound and appears to be at least as sensitive. Chang et al.54 demonstrated that [18F] fluorodeoxyglucose (FDG)-PET was superior to conventional work-up (i.e., chest x-ray, isotope bone scan, and abdominal ultrasound) in detection of distant metastases, where 12% of patients were upstaged to stage IVC. PET, in the study, offered sensitivity and specificity of 100% and 90.1%, respectively. However, large comparative studies of these various staging modalities have yet to be reported.54,55–56
The intimate association of EBV with nasopharyngeal carcinoma, independent of geographic and ethnic background, has provided clinicians with a tumor marker for disease diagnosis. Immunoglobulin (Ig) A anti-viral capsid antigen (VCA) and IgG anti–early antigen (EA) antibodies are both sensitive for diagnosing nasopharyngeal carcinoma; however, IgA anti-VCA has better specificity.57 More than 90% of untreated nasopharyngeal carcinoma patients from California, East Africa, and Hong Kong have elevated IgA antibody titers.58–60 Elevated IgA anti-VCA and IgG anti-EA antibody titers are typically associated with nonkeratinizing carcinoma (both the differentiated and undifferentiated histologic subtypes). Neel et al.61 reported 82% and 86% of patients with nonkeratinizing carcinoma had elevated IgA anti-VCA and IgG anti-EA antibody titers, respectively, contrasted with only 16% and 35%, respectively, in patients with the keratinizing histologic type. IgA anti-VCA antibodies may serve as a screening test in high-risk patients, as they can be found elevated in patients months before the onset of symptoms.62,63 A baseline test of plasma EBV DNA may be useful for prognosis, and levels over time can be utilized as surveillance in a posttreatment setting.64,65
FIGURE 41.10. A: Axial T1-weighted magnetic resonance image (MRI) with 5-mm slices. B: Axial T1 MRI with 3-mm slices; skull-base invasion (arrow) upstaged this tumor from T1 to T3.
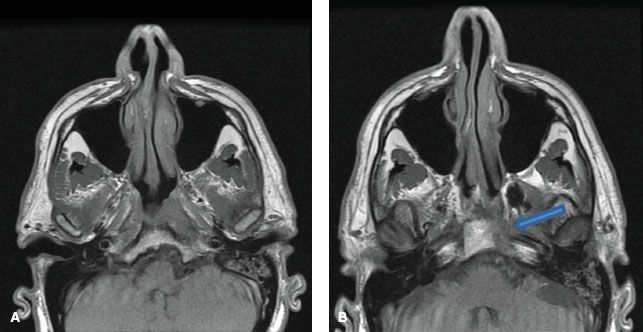
FIGURE 41.11. Two examples of coronal magnetic resonance images showing bilateral cervical lymphadenopathy. There is orderly downward lymphatic spread toward the supraclavicular fossa.
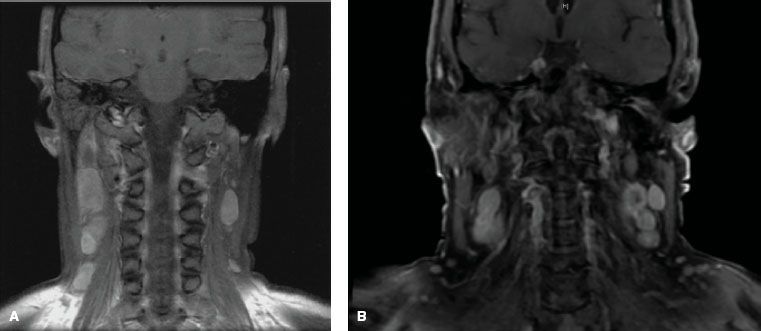
FIGURE 41.12. Positron emission tomography coupled with computed tomography (PET-CT) for a patient with nasopharyngeal carcinoma. Physical examination and biochemistry did not show any sign suggestive of distant metastases. X-ray of chest was normal. PET-CT revealed multiple distant metastases in lung, liver, and spleen, in addition to extensive local infiltration and bilateral cervical lymph nodes. (From Chan J, Bray F, McCarron P, et al. Nasopharyngeal carcinoma. In: Pathology and genetics of head and neck tumours. Lyon, France: IARC Press; 2005:85–97, with permission.)
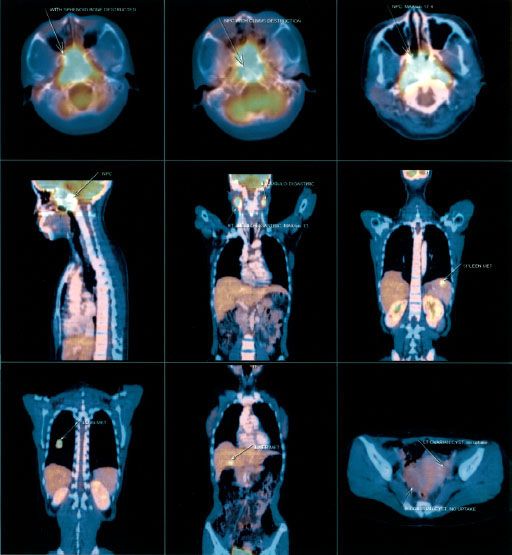
STAGING SYSTEM
Various staging systems have been devised to predict prognosis and guide treatment strategy for patients with nasopharyngeal carcinoma.66–69,70 The AJCC, International Union Against Cancer (UICC), and Ho staging systems are the most commonly used systems in the English-language literature. The AJCC and UICC systems are virtually identical in their 2002 version, and this continues in the 2010 update. Each system has particular limitations; however, they continually evolve and build upon each other’s experience. Table 41.6, left, displays the AJCC staging system currently in use. One advantage of the Ho system is its approach to N-stage classification, which uses level or location of nodal involvement. This N-staging appears superior to that of the AJCC/UICC system, which is primarily based upon the laterality, size, and number of lymph node involvements.14,38,71 However, for the first time, the 2010 AJCC system includes spread of disease to retropharyngeal lymph nodes as N1 classification. These nodal sites are considered the first echelon of nodal metastasis and were involved in 83% of nasopharyngeal carcinoma patients, compared with 74% with involvement of level II to IV neck nodes.72
Other noteworthy changes in the most recent AJCC system are in regard to the T-stage classification. In the 2002 AJCC system (Table 41.6, right), invasion of the nasopharynx soft tissue was used to separate T1 and T2 tumors, however, studies have shown that this distinction has no prognostic significance.71,73,74 Parapharyngeal extension had been found to have prognostic value75–76,77 and had been used to segregate T2a and T2b. The new 2010 AJCC system now separates tumors with parapharyngeal involvement into the T2 subgroup, while all tumors confined to the nasopharynx or with extension into oropharynx or nasal cavity without parapharyngeal involvement are classified as T1 (i.e., former 2002 AJCC T1 and T2a is now 2010 AJCC T1). Base-of-skull involvement has a significantly better prognosis than cranial nerve involvement,27,38,78–80 but both were included in the T4 subgroup in the 2002 AJCC system. The 2010 AJCC system downstages base-of-skull involvement to T3.
These modifications to the staging classifications will require continued examination to determine if they improve prognostic accuracy.
PATHOLOGIC CLASSIFICATION
The vast majority of malignant nasopharyngeal tumors are carcinoma (80% to 99%), with the remainder of these lesions (about 5%) being lymphomas.81 Other rare malignant tumors of the nasopharynx include adenocarcinoma, plasmacytoma, melanoma, and sarcomas. Regarding nasopharyngeal carcinoma, the current World Health Organization (WHO) pathologic classification,82 released in 2005, includes three major types (Fig. 41.13). Keratinizing squamous cell carcinoma is distinguished by the presence of keratin pearls or intracellular keratin. Nonkeratinizing carcinoma is characterized by the complete absence of keratin formation and is further subdivided into differentiated and undifferentiated subtypes. The third type is known as basaloid squamous cell carcinoma83 and is composed of closely packed small tumor cells that form a lobular and, at times, pallisading pattern along with focal squamous carcinoma elements. Basaloid squamous cell carcinoma is quite rare, with a frequency of <0.2%82 (Fig. 41.13). The nonkeratinizing type has a strong association with EBV positivity.84 The keratinizing type may have a correlation with HPV; however, the small sample size of these studies necessitates continued investigation.85
The histologic differences between these three types are by no means distinct. Lesions can share intermediate features, and some may be histologic hybrids. Lymphoepithelioma or lymphoepithelial carcinoma is considered a morphologic variant of undifferentiated carcinoma in which many lymphocytes are found among the tumor cells. Geography, race, and national origin affect the distribution of the WHO histologic types (Table 41.7). The frequency of nonkeratinizing carcinoma varies from 99% in Hong Kong to 75% in the United States.82
Of note, the former WHO classification remains quite commonly used and classifies the three histologic types as follows: (I) squamous cell carcinoma, (II) nonkeratinizing carcinoma, and (III) undifferentiated carcinoma.66 This leads to unnecessary confusion with the new WHO classification, as the former classification was used in the majority of older studies.
FIGURE 41.13. Photomicrographs of nasopharyngeal carcinoma. A: Keratinizing squamous cell carcinoma. B: Nonkeratinizing carcinoma, differentiated subtype. C: Nonkeratinizing carcinoma, undifferentiated subtype. D: Basaloid squamous cell carcinoma. (From Chan J, Bray F, McCarron P, et al. Nasopharyngeal carcinoma. In: Pathology and genetics of head and neck tumours. Lyon, France: IARC Press; 2005:85–97, with permission.)
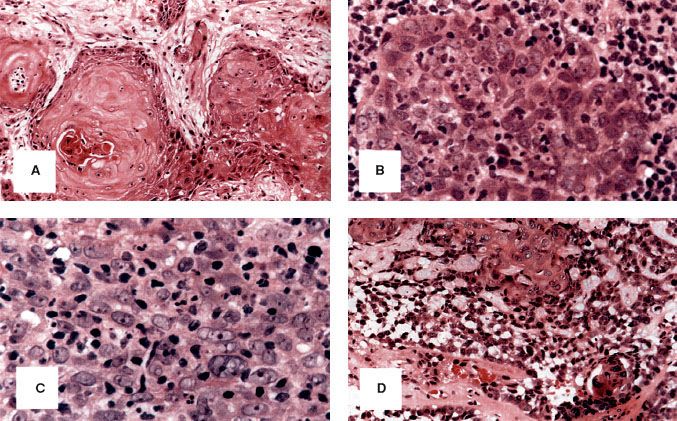
TABLE 41.6 AMERICAN JOINT COMMITTEE ON CANCER STAGING OF NASOPHARYNGEAL CANCER, 2010 AND 2002
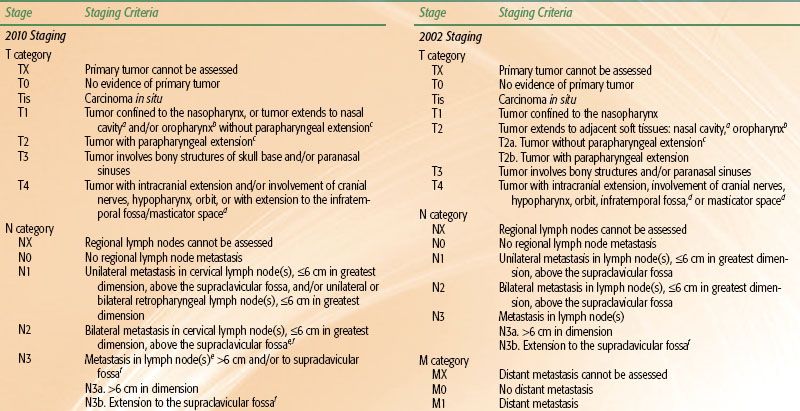
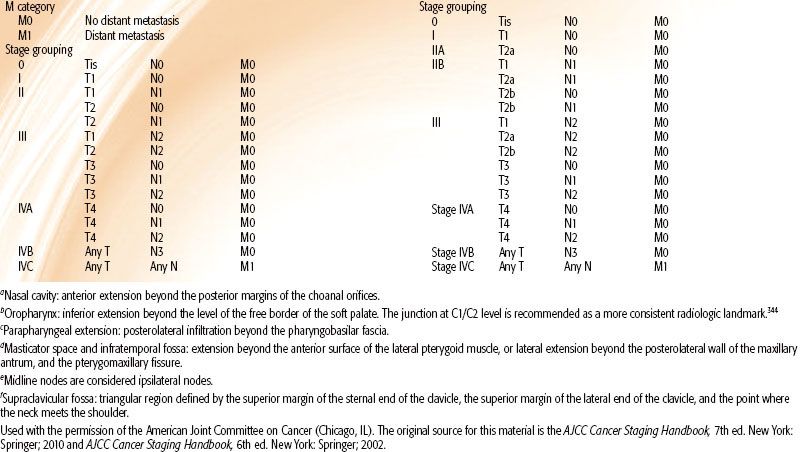
TABLE 41.7 FREQUENCY OF DIFFERENT HISTOLOGIC SUBTYPES OF NASOPHARYNGEAL CARCINOMA
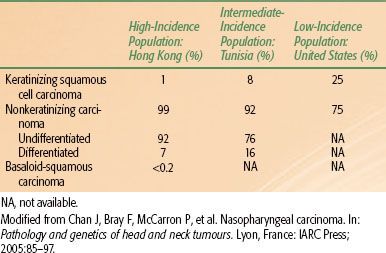
PROGNOSTIC FACTORS
The extent of local invasion, regional lymphatic spread, and distant metastasis, as reflected by the TNM staging, is the most important prognostic factor. In general, advanced T-category is associated with worse local control and overall survival; advanced N-category predicts increased risk of distant metastasis and worse survival. Presence of distant metastasis (M1) upon presentation usually indicates poor prognosis, and treatment has conventionally been palliative in nature. A summary of the patterns of failure and survival rate for the different stages can be found in the Results of Treatment section.
The association of bone erosion, cranial nerve palsy, and lower nodal level with poorer survival is largely undisputed.86–87,88–89 However, the prognostic significance of parapharyngeal extension has been a topic of controversy. In a study of 364 patients, Chua et al.90 showed that greater tumor extension as defined by extension to the prestyloid space or extension to the anterior part of the masticator space was associated with a worse local failure-free rate (L-FFR; 72% vs. 86%) and lower distant failure-free survival rate (D-FFR; 68% vs. 87%) compared to tumors with no extension or extension only to the retrostyloid space. Other investigators reported similarly significant findings.75,76,88,91,92 Cheng et al.93 found parapharyngeal space extension to be the key factor in distant metastasis, even in N1 and N2 NPC.
However, Teo et al.87 did not find parapharyngeal space involvement to be an independent significant prognosticator in a study of 903 patients. Au et al.,94 using the AJCC/UICC definition of extension beyond the pharyngobasilar fascia in a study of 1,294 patients, also found that parapharyngeal extension was not a significant factor upon multivariate analysis.
These contradictory findings may be attributed to varying definitions of parapharyngeal space and incidence in the different series,75,87,88,91 prompting some to advocate for consideration of the degree of parapharyngeal space extension in future staging systems.76 In addition, suboptimal imaging by CT and conflation with retropharyngeal node enlargement likely contribute to the debate.95,96
Nevertheless, the most recent 2010 AJCC staging system (Table 41.6) downgraded involvement of oropharynx and/or nasal cavity without parapharyngeal extension from T2a to T1 while designating the presence of parapharyngeal extension to be the sole determinant of T2 classification. This was in light of recent multiple large retrospective studies that found no significant difference in disease failure hazard ratios between former AJCC 2002 T2a and T1.73,77,93,97
One recent topic of interest has been the prognostic significance of prevertebral space involvement (PSI). Recent MRI-based studies reported PSI to be an independent prognostic factor in cases of NPC treated with two-dimensional (2D) radiation therapy.98,99 In a study of 506 patients treated with intensity-modulated radiotherapy (IMRT), Zhou et al.100 found PSI to independently predict overall survival (OS) and distant metastasis-free survival (DMFS) rates similar to those of T4 disease and advocated for its inclusion as a T4 parameter in future AJCC staging systems.
Another prognostic factor to consider is the gross volume of the primary tumor (GTV-P). Although it is highly correlated to T-stage, considerable variability in tumor volume exists within the same T-stage, and evidence increasingly suggests that tumor volume as an independent significant factor can better predict prognosis than T-category as specified by both AJCC/UICC and Ho systems101,102–103,104,105 (Fig. 41.14).
In a study of 308 patients staged with MRI, Sze et al.103 showed that those with GTV-P of <15 cm3 had significantly higher L-FFR than those with a value of ≥15 cm3 (97% vs. 82% at 3 years; p < 0.01). Multivariate analysis confirmed GTV-P to be a strongly significant factor independent of T-category by the 1997 fifth edition of the AJCC/UICC; the risk of local failure increased by 1% for every 1-cm3 increase in volume. A similar observation between GTV and clinical outcomes was observed in other head and neck tumors, including oropharyngeal cancers.106 Further study is required to determine how tumor volume may be optimally incorporated into future staging systems.
Most series found significantly better prognosis for females and younger patients.86,94,107 In 759 patients, Sham and Choy86 showed a higher 5-year survival rate in females compared with males (45% vs. 28%) and in patients younger than 40 vs. older than 40 years of age (50% vs. 40%, p = .002). However, they did not find age to significantly affect the 10-year survival rate. Multivariate analysis of 1,294 patients by Au et al.94 also showed worse cancer-specific death rates in males (hazard ratio [HR] = 1.28, p = .02) and patients older than 50 years (HR = 1.79, p <0.001).
Although not all studies found histology to be an independent prognostic factor,27,108 many found nonkeratinizing and undifferentiated carcinomas (formerly known as lymphoepitheliomas) to be more radiosensitive and offer better prognosis than keratinizing squamous cell carcinoma.31,109,110 Of note, regarding ethnicity as a prognostic factor, a study by Corry et al.111 showed no prognostic difference between ethnic Asian and non-Asian patients with nonkeratinizing carcinoma.
FIGURE 41.14. The correlation between T-category and gross volume of primary tumor (GTV-P). UICC, International Union Against Cancer. (Modified from Sze W, Lee A, Yau T, et al. Primary tumor volume of nasopharyngeal carcinoma: Prognostic significance for local control. Int J Radiat Oncol Biol Phys 2004;59:21–27.)
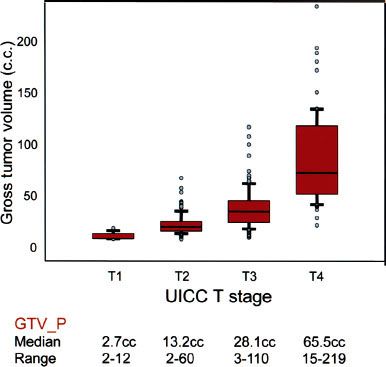
EBV and Other Biomarkers
Because of the association of EBV with NPC, various anti-EBV antibodies have long been studied for their potential as biomarkers. While some studies showed that elevated level of serum anti-EBV antibodies could indicate presence60,112,113 of disease, others showed anti-EBV antibody titers to have little value for posttreatment surveillance.61,114,115 The prognostic value of such titers prior to treatment has also been controversial. While Xu et al.116 found that high EBV DNase-specific neutralizing antibody at diagnosis predicted significantly worse event-free and overall survival, others found that a number of antibodies (VCA-IgG, VCA-IgA, EA-IgG, EA-IgA, EBNA-IgG, EBNA-IgA) could not predict prognosis.113,117,118
Circulating cell-free DNA of EBV in the plasma of NPC patients is a significant prognostic marker and has been found to be superior to serum anti-EBV antibodies.118 Lo et al.119 showed that plasma EBV DNA had high sensitivity (96%) and specificity (93%) for detecting NPC, while Ma et al.120 showed that circulating EBV DNA levels correlated significantly with tumor burden. Studies by Lo et al.121 and Lin et al.64 found that high pretreatment levels were associated with advanced stages and poor prognosis. Meanwhile, Leung et al.65 showed that pretreatment plasma EBV DNA load was an independent prognostic factor for OS in 376 patients and could be used to segregate early-stage patients into poor-risk and high-risk subgroups. Thus, pretreatment EBV DNA assays have the potential to complement TNM staging in guiding treatment.
Although Le et al.122 found no correlation between pretreatment EBV DNA levels and survival, they did find posttreatment levels to be a strongly significant predictor of outcome; patients with no detectable EBV DNA had a 2-year OS rate of 94% versus 55% for patients with detectable posttreatment levels (p <0.002).
Other studies also consistently reported that patients with elevated posttreatment EBV DNA load had higher risk of tumor recurrence.64,121,123–125 Using multivariate analysis to compare various prognostic factors for NPC, Lin et al.126 found that the combined EBV DNA load (pretreatment and 1-week posttreatment) was the most significant factor.
More recently, both Wang et al.127 and An et al.128 demonstrated that the clearance rate of plasma EBV DNA during the first month of salvage chemotherapy could predict tumor response and overall survival in patients with metastatic/recurrent NPC; undetectable levels after the first cycle indicated significantly better survival. These data suggest that early evaluation of plasma EBV DNA can offer oncologists timely insight for potential alterations in the therapeutic regimen for patients with a slow clearance rate. In addition, Wang et al.129 prospectively monitored the plasma EBV DNA of 245 NPC patients in clinical remission with assays every 3 to 6 months and found the plasma EBV DNA assay to have much greater sensitivity, specificity, and accuracy than FDG-PET in predicting relapse, suggesting its utility for posttreatment surveillance.
As with other head and neck squamous cell carcinomas, EGFR is commonly expressed in patients with NPC. Chua et al.130 found expression of EFGR in 89% of patients, in which overexpression was associated with significantly poorer disease-specific survival. Others reported similar findings of prognostic significance.24,131,132 Ma et al.131 performed multivariate analysis on several biomarkers in 78 patients, including microvessel density, Ki67 antigen, p53 oncoprotein, HER2, and EGFR, and found EGFR to be the only independent prognostic factor.
The study by Hui et al.133 showed that 58% of NPC patients had expression of hypoxia-inducible factor 1α (HIF-1α), 57% had carbonic anhydrase IX (CA IX), and 60% had vascular endothelial growth factor (VEGF). Those with positive hypoxic profile (high expression of HIF-1α and CA IX) had a worse progression-free survival (p = .04); those with both positive hypoxic and angiogenic profile (high VEGF) were strongly associated with worse progression-free survival (p = .0095). Multiple other studies have also showed that overexpression of these markers, particularly VEGF, is associated with poorer survival.134–136
Other biologic factors that might have prognostic significance include E-cadherin and β-catenin,137 c-erbB2,138 p53,139 NM23-HI,140,141 and interleukin-10.142 Further validation of these potential biomarkers is needed.
TREATMENT STRATEGY
Because of the anatomic location—proximity to critical structures—surgical exposure and tumor resection with sufficient margins have been very challenging.143 Primary surgical intervention was rare after the 1950s for these reasons, with surgical interventions employed mainly for biopsy to gain histologic confirmation and salvage therapy for persistent or recurrent cancer. Primary treatment since has typically employed radiotherapy (RT) alone and, more recently, in combination with chemotherapy.
Radiation Therapy
To achieve the best therapeutic ratio, every single step in the RT procedures (localization of gross tumor and target volumes, immobilization, optimization of dose fractionation, determination of treatment techniques, and precision in RT delivery) is important.
For planning, the patient should be set up in a supine position with head extended for adequate separation between the primary tumor/retropharyngeal nodes and the upper neck nodes. The tip of the uvula and the base of the occiput should be on a parallel plane to the beam axis. The patient is immobilized with a thermoplastic mask covering the head-to-shoulder region (Fig. 41.15). For patients to be treated by conventional 2D technique, a mouth bite is useful to minimize the dose to the oral cavity, with enlarged neck nodes to be marked with wire before imaging.
FIGURE 41.15. Immobilization of patient in a customized thermoplastic mask covering the whole head-to-shoulder region.
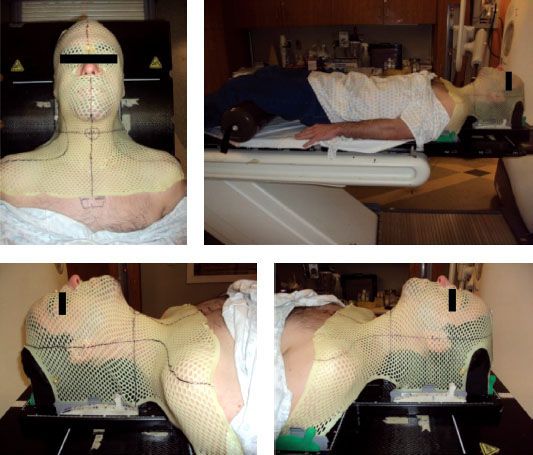
Dose, Time, and Fractionation
A significant dose–response relation was observed in the majority of retrospective studies, based on patients irradiated with 2D techniques. Marks et al.144 and Vikram et al.145 showed that local control was significantly improved in patients who received >67 Gy to the tumor target. Perez et al.27 observed that patients with T1-2 tumors had a local tumor control rate of 100% for those given >70 Gy, compared with 80% for those treated with 66 to 70 Gy. However, local control for patients with T3-4 tumors remained <55%, even with total dose >70 Gy. Similar findings were reported by Mesic et al.,146 where ≥70 Gy achieved better local control for T1-2 tumors than 60 Gy (94% vs. 76%), but higher doses or larger fields did not significantly improve outcomes in T3-4 tumors. These observations suggest that, besides consideration of the prescribed dose, the problem of sufficient coverage has to be overcome for advanced tumors.
Lee et al.147 reported a study of 1,008 patients with T1 tumors irradiated by four different fractionation schedules and demonstrated that total dose was the most important radiation factor (p = .01). Dose fraction did not affect local control; however, it was a significant risk factor for temporal lobe necrosis.148,149 Therefore, a fractional dose of >2 Gy should be avoided150 (see section Sequelae of Treatment).
The impact of the time factor is more contentious. A randomized study by Marcial et al.151 in which 62 patients were treated with split-course irradiation (30 Gy in 10 fractions over 2 weeks, then a 3-week rest period, followed by an additional 30 Gy in 10 fractions) and compared with 59 patients with 66 Gy in 33 fractions in 6.5 to 7 weeks demonstrated no significant difference in 5-year local control (86% vs. 80%), nodal control (86% vs. 78%), or disease-free survival (40% vs. 30%).
However, Vikram et al.145 observed that patients with interruption of RT for ≥21 days had significantly poorer local tumor control than patients without interruptions (34% vs. 67%). Similar findings have been subsequently reported,152,153 with the general consensus that prolongation is likely to be detrimental, even for nonkeratinizing NPC.
In general, the prescription recommended for NPC is to a total dose of about 70 Gy over 7 weeks to the gross tumor along with 50 to 60 Gy for elective treatment of potential risk sites.
TABLE 41.8 EXAMPLE OF GUIDELINE ON ANATOMIC STRUCTURES/BOUNDARIES FOR DELINEATING CLINICAL TARGET VOLUMES FOR INTENSITY-MODULATED RADIATION THERAPYA
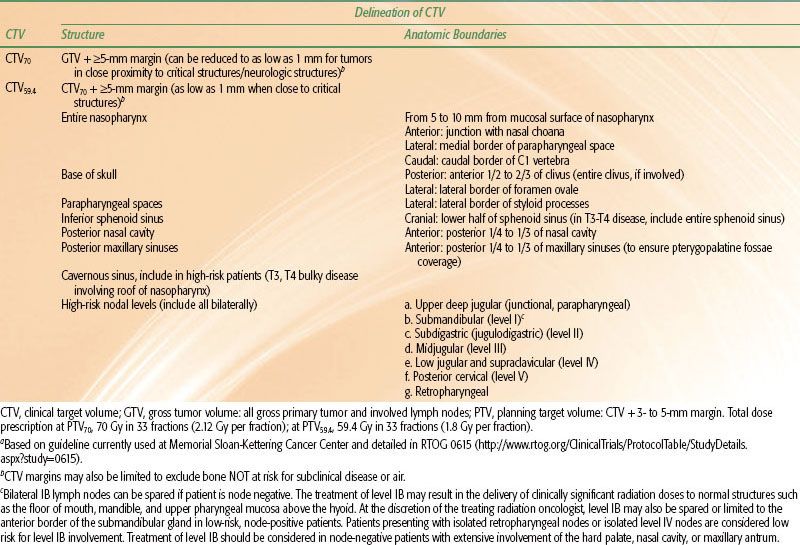
Tumor Target Volumes
The GTV should encompass the primary nasopharyngeal tumor, gross retropharyngeal lymphadenopathy, and gross nodal disease as determined by clinical, endoscopic, and radiologic examinations. Presence of lymph nodes of ≥1 cm or with evidence of central necrosis is considered gross nodal disease. For patients given induction chemotherapy, it is recommended that the targets be determined by the prechemotherapy extent.
Prophylactic neck radiation is usually recommended in N0 patients because of the high incidence of occult neck node involvement. Lee et al.35 found that patients with a clinically negative neck who underwent elective neck irradiation had a significantly lower nodal recurrence rate than those who were untreated (11% vs. 40%). In addition, even with successful salvage by subsequent treatment, patients with nodal recurrence had a significantly greater incidence of distant metastases than those without recurrence (21% vs. 6%).
The clinical target volume (CTV) includes the GTV, regions of microscopic disease, and potential infiltrative spread. Different centers may have different philosophies in defining the margins and dose level. For example, Table 41.8 shows the delineation criteria for the various CTVs currently employed at Memorial Sloan-Kettering Cancer Center. A gross disease CTV (CTV70) is defined as the GTV plus an additional margin of 5 mm to 1 cm surrounding all gross disease. The margin may be decreased to as small as 1 mm in critical regions near the brainstem or spinal cord. The high-risk subclinical CTV (CTV59.4) encompasses the GTV including all potential areas of microscopic spread of disease. This volume should include at a minimum the entire nasopharynx; retropharyngeal lymph nodal regions; clivus; skull base; pterygoid fossae; parapharyngeal space; sphenoid sinus; posterior one-fourth to one-third of the nasal cavity; and posterior one-fourth to one-third of the maxillary sinuses. This CTV59.4 should also include lymph nodal groups that are at risk of potential microscopic disease spread: bilateral upper deep jugular (junctional, parapharyngeal), submandibular, subdigastric (jugulodigastric), midjugular, posterior cervical, and retropharyngeal lymph nodes. In patients with clinically N0 neck, it is not necessary to include level I nodal regions.
The planning target volume (PTV) is defined as the CTV including a circumferential margin of typically 3 to 5 mm to all the CTVs to account for setup errors and potential patient motion. The PTV margin may be decreased to as small as 1 mm in regions near critical normal structures such as the brainstem or spinal cord.
Conventional Two-Dimensional Treatment Techniques
One of the most common RT approaches employed is comprised of two phases.154 Phase I consists of large lateral opposing faciocervical fields that encompass the primary tumor and the upper neck nodes in one volume, with a matching lower anterior cervical field for the lower cervical lymphatics. Phase II is used after 40 Gy to limit the dose to the spinal cord. This three-field technique includes lateral opposing facial fields coupled with anterior facial field for the primary tumor. Typical treatment fields and radiologic landmarks are shown in Figure 41.16. Shrinking treatment fields by cone-down after 50 to 60 Gy should be done, when possible, to increase protection of critical structures.
The three-field technique in phase II allows the dose to be minimized to the temporomandibular joints and the bilateral temporal lobes. However, coverage may not be sufficient for tumors with extensive posterolateral extension to the parapharyngeal spaces or caudal extension to the oropharynx. To remedy this deficit, an additional dose is delivered by a posterolateral field with avoidance of neurologic structures.155
FIGURE 41.16. Conventional two-dimensional radiotherapy using Ho’s technique. A: Phase I, lateral-opposed faciocervical fields (I–II) and lower anterior cervical field (IVb). B: Phase II, sagittal view showing lateral-opposed facial fields and noncoplanar anterior facial field (III). C: Coronal view of anterior facial field (III). D: Anterior cervical field for whole neck (IV).
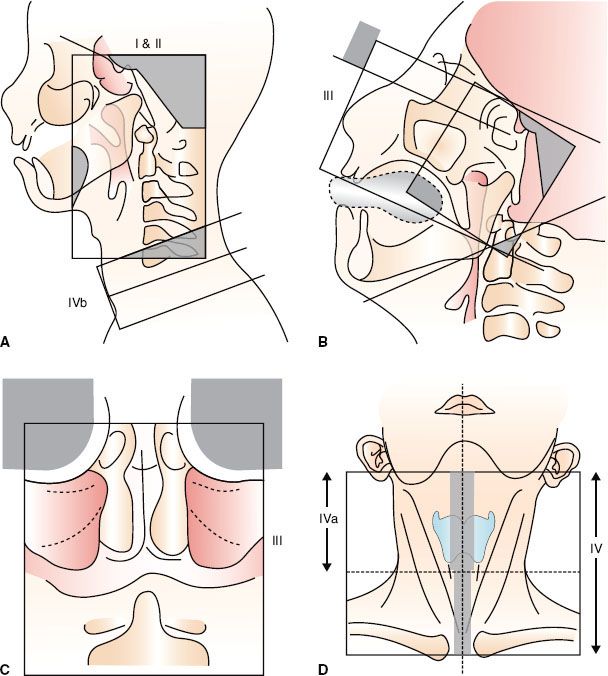
Three-Dimensional Conformal Treatment Techniques
Nasopharyngeal carcinoma presents most typically as a concave tumor, allowing for computerized three-dimensional (3D) treatment plans to be an important technical advance for improved radiation delivery. Several investigators designed multifield conformal plans, including the seven-field technique used at Memorial Sloan-Kettering Cancer Center (MSKCC) (New York, NY)156 and the “Boomerang” technique used at Peter MacCallum Cancer Institute (East Melbourne, Australia).157 When compared to conventional 2D plans, 3D planning demonstrated better tumor dose coverage while decreasing normal tissue dose in several studies.87,158,159
Leibel et al.160 from MSKCC demonstrated that the target volume underdosed at the 95% isodose level was lowered with 3D plans when compared with 2D plans (7% vs. 22%). On average, the mean tumor dose increased 13%, leading to an estimation that tumor control would increase by 15%. However, a subsequent study in which 68 patients received this technique for a boost of 19.8 to 25.2 Gy following phase I conventional 2D treatment for 50.4 Gy to a total dose of 70.2 to 75.6 Gy did not show significant improvement; the 5-year L-FFR was 77% and late toxicity grade ≥3 was 25%.156
More encouraging results were obtained by Jen et al.,161 who compared 72 patients treated with 3D conformal technique with 108 patients treated with 2D technique. A significant improvement in 3-year L-FFR for T4 (86% vs. 47%) and event-free survival for both stage III (80% vs. 56%) and stage IV (82% vs. 33%) was observed. Furthermore, the incidence of xerostomia at 3 years was significantly less with 3D conformal treatment (69.2% vs. 98.0%), although for most other late toxicities little difference was seen.
IMRT Techniques
IMRT has supplanted conventional radiotherapy in the treatment of NPC in an increasing number of institutions throughout the world. The intensity of the radiation beams can be modulated to deliver a high dose to the tumor with a superior target volume coverage while significantly limiting the dose to surrounding normal tissues.162–165,166,167 Following the initial publication168 and the subsequent update on IMRT for NPC from the University of California, San Francisco (UCSF),166 several other institutions have utilized IMRT with similar excellent treatment outcomes.169–170,171–175
Another point of interest is the possibility of biologic enhancement by simultaneous modulated accelerated-radiation therapy (SMART), also known as dose painting, as a new way of delivering an accelerated fractionation (AF) schedule, a concept that was first reported by Butler et al.176 for the treatment of other head and neck cancers with IMRT.
These differing methods and dose fractionation regimens for IMRT are being investigated by different groups. Table 41.9 summarizes the key features along with reported results. The majority of the patients in these series received additional chemotherapy and/or enhanced RT with boosts or AF. All reported encouraging early results, with local control in >90% at 2 to 4 years.
At UCSF patients were typically prescribed 70 Gy to the PTVgross disease and involved lymph nodes in 2.12- to 2.25-Gy fractions, while PTVhigh-risk subclinical patients were prescribed 59.4 Gy in 1.8 fractions, and a clinically negative neck (PTVlow-risk subclinical) patient received 54 Gy at 1.64-Gy fractions, all in conventional once-daily fractions.166,177 Bucci et al.177 reported the updated results of 118 patients and confirmed excellent locoregional control of 96%. Nonetheless, distant failure remained high (28%) despite broad use of concurrent-adjuvant CRT. OS was 74% at 4 years.
At MSKCC, Wolden et al.169 reported their experience with 74 patients: 59 were treated with AF using the concomitant boost method and 15 by the SMART method/dose painting. For the SMART cohort, a total dose of 70.2 Gy at 2.34 Gy/fraction was given to the gross disease, and the “microscopic” PTV received 54 Gy at 1.8 Gy/fraction. There was a trend, but no statistically significant improvement, in 3-year L-FFR than for patients treated by 3D conformal boost (91% vs. 79%, p = .11) Additional dose escalation by SMART boost in 50 patients with T3 to T4 tumors was reported by Kwong et al.178 from Queen Mary Hospital (Hong Kong). They sought to deliver a total dose of 76 Gy at 2.17 Gy/fraction to the gross tumor. The early result for locoregional control was excellent (96% at 2 years); however, serious late toxicities were observed, including 4% of patients having a life-threatening hemorrhage from carotid artery pseudoaneurysm, and another 4% developing temporal lobe necrosis with a median follow-up of 2.1 years.
Two different IMRT approaches are being utilized by different centers: (i) an extended-whole field (EWF) IMRT technique, in which the total target volume is encompassed in the IMRT plan, or (ii) a split-field (SF) IMRT technique, in which the target volumes superior to the vocal cords are treated with an IMRT plan and the lower neck nodes are treated with a conventional low anterior neck field.179–181 Discussions among practitioners on which IMRT technique is best have been persistent. Concerns of potential failures at the SF matchline due to potential underdosing, or even complications resulting from overdosing, have caused many centers to implement EWF IMRT even when no clinically involved neck nodes are evident in the matchline region. These concerns may be caused by the treatment delivery system that is used at centers where a perfect match between the IMRT fields and the low anterior neck field is not possible. However, with a EWF technique, an unnecessary dose of radiation is delivered to the normal glottic larynx,182 whereas with the SF IMRT technique, the dose to the vocal cords is minimal due to shielding by a midline Cerrobend block or the multileaf collimator (MLC). No IMRT matchline failures or complications have been reported with the SF IMRT technique.168
TABLE 41.9 INTENSITY-MODULATED RADIATION THERAPY FOR NASOPHARYNGEAL CARCINOMA: METHODS AND RESULTS BY DIFFERENT CENTERS
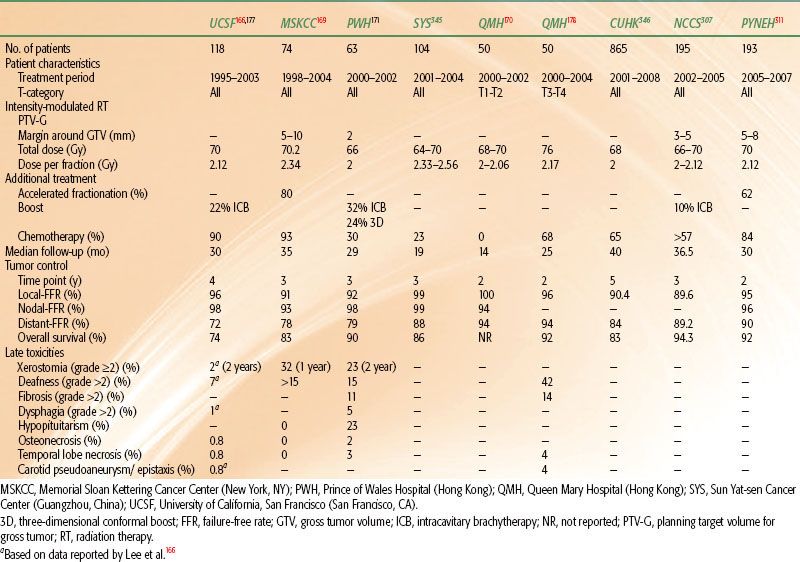
FIGURE 41.17. Intensity-modulated radiation therapy for a patient with T2N2M0 nasopharyngeal carcinoma treated at Memorial Sloan-Kettering Cancer Center, showing delineation of gross tumor target (GTV) and planning target volume (PTV) for 70 and 54 Gy, the dose distribution, and the dose volume histogram (DVH) for GTV, PTV70, and PTV59.4.
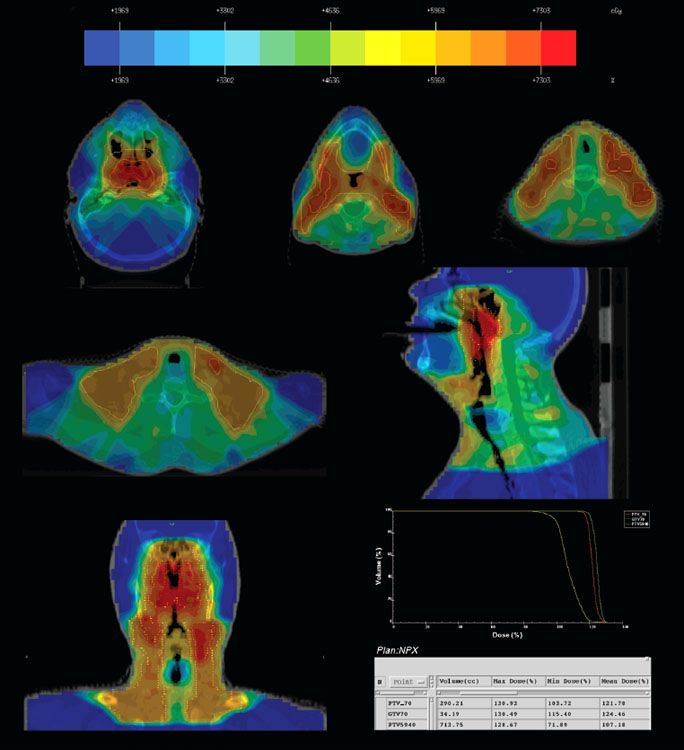
Figures 41.17 and 41.18 show examples of MSKCC IMRT plans delivered with the dynamic MLC system using a sliding-window technique to patients with early and advanced disease, respectively. A total dose of 70 Gy at 2.12 Gy/fraction to the PTVgross disease and 59.4 Gy to the PTVhigh-risk subclinical patients is given over 33 once-daily fractions (Table 41.8). For the low neck, if split-field IMRT is used, a dose of 50.4 Gy at 1.8 Gy/fraction/day is generally prescribed. However, if the low neck is included in the IMRT fields and is considered at low risk for nodal involvement, the PTVlow-risk subclinical patient typically receives 54 Gy at 1.64 Gy/fraction per day.
Inverse planning involves the appropriate specification of normal tissue dose constraints. It is important to note that overstringent use of normal tissue constraints might result in inadequate cover of tumor targets, and therefore optimal balance is essential. Different dose constraint guidelines have been suggested.183,184 An example of dose-constraint guidelines is provided in Table 41.10, which displays the guidelines used at Memorial Sloan-Kettering Cancer Center.
Dose Escalation
Excellent local tumor control has been reported by delivering an additional boost to patients with early disease treated by conventional 2D technique.
FIGURE 41.18. Intensity-modulated radiation therapy for a patient with T4, N2, M0 nasopharyngeal carcinoma treated at Memorial Sloan-Kettering Cancer Center, showing delineation of gross tumor volume (GTV) and planning target volume (PTV) for 70 and 54 Gy and the dose distribution.
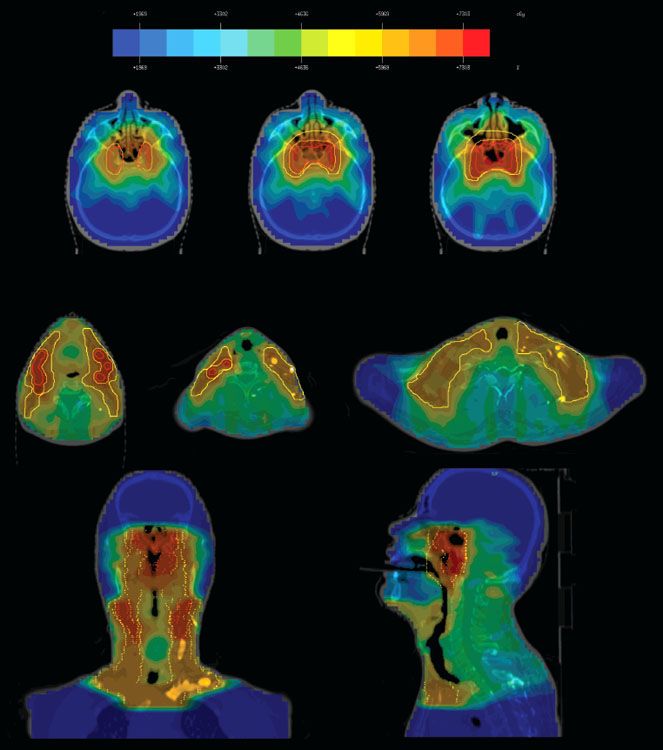
TABLE 41.10 INTENSITY-MODULATED RADIATION THERAPY FOR NASOPHARYNGEAL CARCINOMA: AN EXAMPLE OF NORMAL TISSUE DOSE CONSTRAINTSA
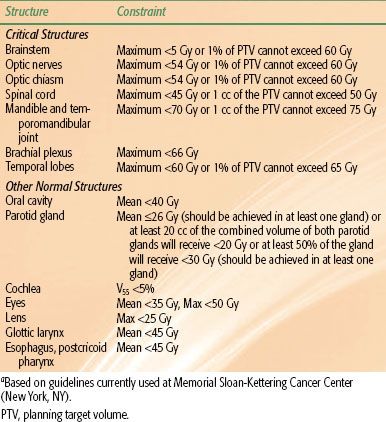
Brachytherapy
The most commonly used method is brachytherapy. Intracavitary insertions185–193 or interstitial implants194–197 have been used in T1 to T3 nasopharyngeal carcinomas as a boost treatment following external beam irradiation (EBRT) or in the treatment of recurrent disease, either alone or in combination with EBRT. Brachytherapy is not suitable for treatment of tumors with intracranial extension because of the rapid reduction of dose as distance from the radioactive source increases. Since the advent of IMRT as primary radiotherapy for nasopharyngeal carcinoma and with its demonstration of excellent local control, the use of brachytherapy as a boost treatment following definitive IMRT has dramatically declined. Multiple applicators and techniques have been developed for the delivery of intracavitary brachytherapy.187,188,189,191,193,198 In the past, intracavitary brachytherapy was delivered using low–dose rate (LDR) techniques. However, at present, remote afterloading, fractionated high–dose rate (HDR) techniques are more commonly used (Fig. 41.19).198
Table 41.11 summarizes reports on the use of brachytherapy as a boost for dose escalation. Most studies demonstrated that local control of up to 90% to 95% could be achieved for T1-2 tumors without excessive late damages. A retrospective comparison by Wang188 from Massachusetts General Hospital (Boston, MA) reported that T1 to T2 patients who received a 10- to 15-Gy LDR brachytherapy boost after 60 to 64 Gy by EBRT had a 5-year L-FFR of 90% versus 54%, respectively, with p = .001 for patients receiving EBRT alone to 65 to 70 Gy. A similar study by Teo et al.199 in which delivery of 18 to 24 Gy in three fractions by HDR brachytherapy showed significant improvement of the 5-year L-FFR of 95% compared with 90% 5-year L-FFR for EBRT-only patients (p = .016).
However, a report by Ozyar et al.200 of patients with T1 to T4 tumors treated with HDR brachytherapy boost of 12 Gy in three fractions did not show improvement over EBRT alone (3-year L-FFR,86% vs. 94%; p = .23). More recently, a prospective trial by the International Atomic Energy Agency studied 275 patients with locoregionally advanced NPC disease (TNM stages III or M0 stage IV) who were all treated by induction chemotherapy followed by concurrent chemoradiotherapy to 70 Gy; one randomized arm then received a brachytherapy boost of 11-Gy LDR or three fractions of 3-Gy HDR. With a median follow-up of 29 months, the authors reported no additional benefit of brachytherapy boost compared with chemoradiotherapy alone toward 3-year OS (63.3% vs. 62.9%, p = .742, respectively), locoregional-FFR (54.4% vs. 60.5%, p = .647), or distant-metastasis–free survival (52.6% vs. 59.8%, p = .496).201
One major limitation of brachytherapy is that the dose delivered is adequate only for superficial nonbulky tumors. Furthermore, optimal positioning of the applicators depends both on the individual clinician’s skill and the patient’s anatomic features.
Stereotactic Radiosurgery
Stereotactic radiosurgery (SRT) or fractionated radiotherapy allows for precise delivery of highly conformal RT with a rapid dose falloff and provides an alternative for dose escalation. Hara et al.202 reported a study of 82 patients with T1 to T4 tumors showing excellent 5-year L-FFR of 98% after receiving a median SRT boost of 12 Gy (range, 7 to 15 Gy) following EBRT to 66 Gy. However, despite the addition of concurrent chemotherapy in 76% of the patients, the distant failure rate was 32% and OS was 69%. With a median follow-up of 40.7 months for living patients, 12.1% of patients developed radiographic temporal lobe necrosis (only 2.4% were symptomatic with seizures), and 3.6% developed retinopathy. The risk was especially high in patients with T4 tumors.
FIGURE 41.19. Endocavitary brachytherapy for nasopharyngeal carcinoma. A: The Rotterdam nasopharyngeal applicator. B: The simulator check-film showing the position of the radioactive sources and the dose distribution.
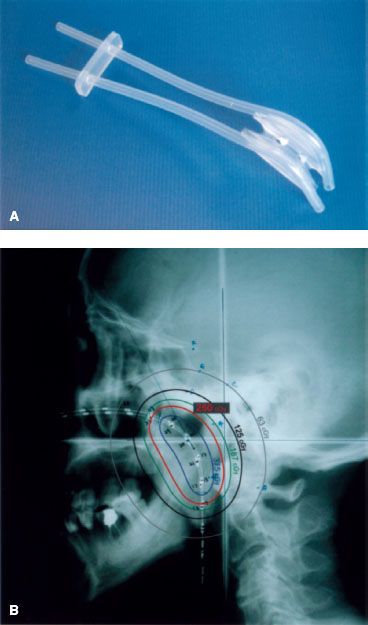
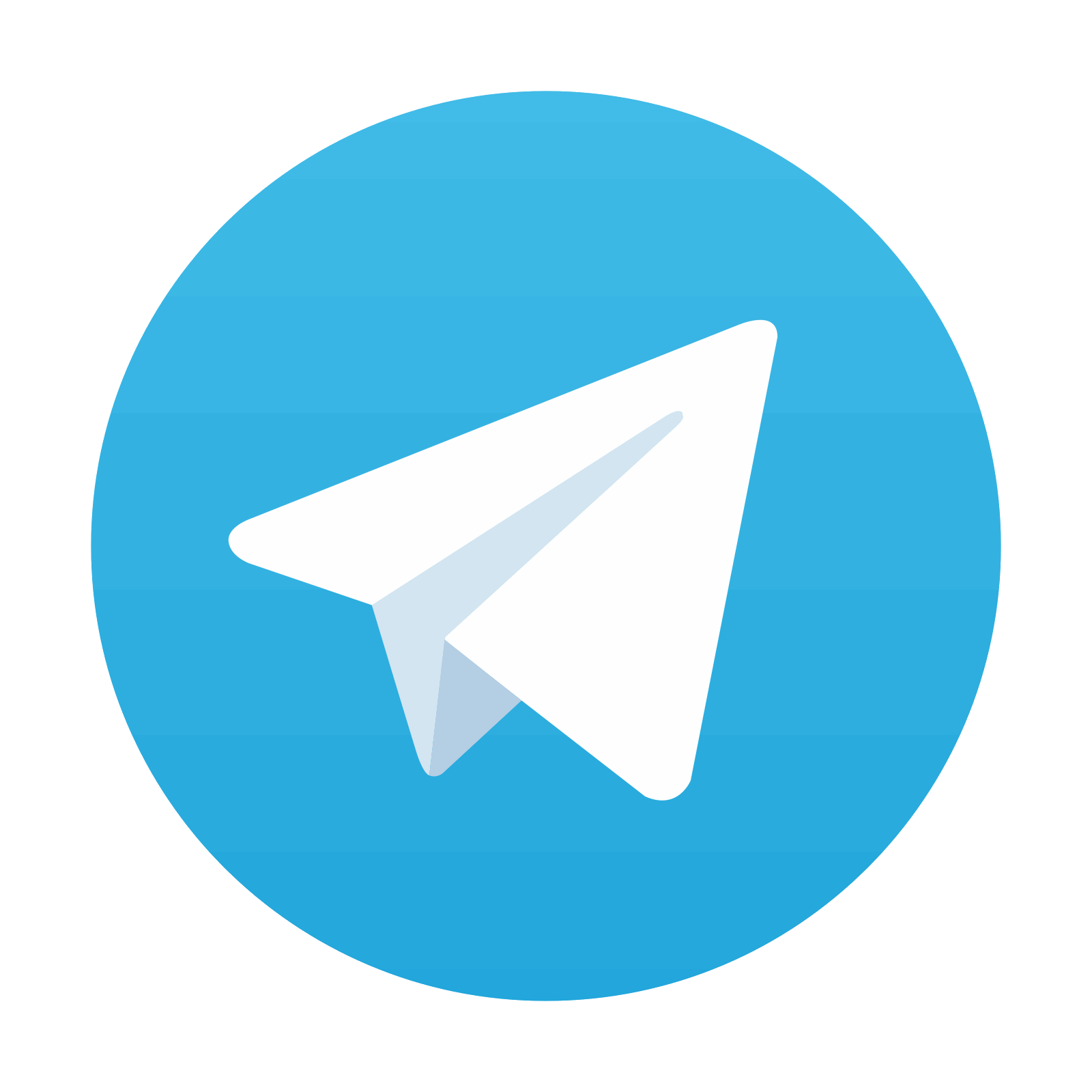