Molecular Basis for Platelet Secretion
Shaojing Ye
Sidney W. Whiteheart
INTRODUCTION
Platelets are small, anucleate, discoid cell fragments produced by bone marrow megakaryocytes. Structurally, platelets are simple, containing plasma membrane with a specialized region known as the open canalicular system (OCS), microtubules, mitochondria, and three types of granules: dense granules, α-granules, and lysosomes. Upon encountering a vascular lesion, platelets become activated and initiate thrombosis. Engaging cell surface receptors initiates a number of intracellular signaling cascades leading to increased intracellular Ca2+ and inducing dramatic alterations in platelet shape mediated by actin cytoskeletal rearrangements. Platelet activation also leads to the release of their granular stores, and the resulting releasate affects the microenvironment at the site of damage. Released molecules attract and activate more platelets to propagate thrombosis and initiate sequelae of thrombosis such as wound healing and leukocyte recruitment. Thus, platelet secretion is not only important for hemostasis, but is also associated with many other vascular processes, both normal and potentially pathologic. Further, understanding the mechanism of platelet secretion has the potential to identify new targets for the development of novel antithrombotic therapeutics to control thrombosis and to affect pathophysiologic aspects of platelet function that participate in atherosclerosis, tumor angiogenesis, and inflammation.
At sites of vascular injury, platelets release cargo from three granular stores: dense granules contain small, bioactive molecules,1,2,3 α-granules contain bioactive peptides and proteinaceous components,4,5 and secretory lysosomes contain hydrolytic enzymes.6 Release of dense granule cargo is clearly important to hemostasis, given the bleeding diatheses associated with genetic defects in dense granule biogenesis (i.e., Hermansky-Pudlak syndrome [HPS]7). Released adenosine diphosphate (ADP) plays a key role in enhancing the responsiveness to other agonists generated at the site of vascular damage.8 Release of α-granule cargo has a more heterogeneous impact. Patients who lack α-granules (i.e., gray platelet syndrome [GPS]) generally have more diverse bleeding phenotypes, ranging from mild to severe.9,10 The role that lysosome release plays is unclear; it is plausible that the released hydrolytic enzymes could be important for thrombus remodeling. Nonetheless, while the physiologic importance of platelet secretion is clear, the nature of the machinery that controls and mediates platelet granule exocytosis is not. This chapter discusses our present state of knowledge of the molecular mechanisms of platelet secretion.
PLATELET GRANULES AND THEIR CARGO
As with other regulated secretory systems, for example, neurons, release of cellular stores occurs in response to external stimuli. The resulting signaling steps mobilize the secretory machinery that then mediates the fusion of cargo-containing granules with the plasma membrane to facilitate cargo release into the extracellular environment. Each of the classes of platelet granules is released upon stimulation, but to differing degrees, depending on the levels of platelet activation.
Dense Granules
Platelets contain approximately three to eight electron-dense organelles, 20 to 30 nm in diameter.3,11 The electron density of dense granules, when viewed in unstained whole mounts, is due to the relative insolubility of calcium salts of the various compounds stored in dense granules (i.e., CaPO4). Dense granules contain high concentrations of serotonin, which is taken up from plasma by a plasma membrane transporter and then concentrated in the granules.3 ADP and adenosine triphosphate (ATP) are also highly concentrated in dense granules. There is more ADP than ATP in the dense granules, which is the reverse of their relative cytosolic concentrations.12 Calcium, polyphosphates, sphingosine-1-phosphate, and epinephrine are also found in dense granules.12,13,14 The concentration of serotonin, and perhaps other dense granule cargo, is the result of the lower pH (˜6.1) maintained in dense granules due to the action of a H+ pumping ATPase on the granule membrane.3
α-Granules
α-Granules are the most abundant granule type in platelets, numbering approximately 50 to 80 per platelet.15,16 They are approximately 200 nm in diameter and show variations in electron density; often, they contain a central electron-dense area termed a nucleoid.17 The more electron-lucent areas often contain tubular elements in which von Willebrand factor (vWF), multimerin, and factor V are preferentially localized.18 Platelets may contain distinct subpopulations of α-granules that undergo differential release of α-granule cargo during activation. For example, some α-granules appear to contain proangiogenic proteins, such as vascular endothelial growth factor (VEGF), whereas others contain antiangiogenic factors, such as endostatin.19 Alternatively, these factors may be distributed in distinct regions within the same membrane-bound granule.20,21 This is yet to be resolved.
α-Granules acquire their cargo through both biosynthesis (predominantly at the megakaryocyte level) and endocytosis (at both the megakaryocyte and circulating platelet levels). Small amounts of many plasma proteins are found in α-granules; thus, the plasma concentrations of these proteins determine the amount present in platelets.22,23 For example, the α-granule pool of immunoglobulins contains most of the platelet immunoglobulin;
therefore, total platelet immunoglobulin is more affected by changes in plasma immunoglobulin levels than by changes in surface immunoglobulin. Other proteins, such as VEGF, factor V, and fibrinogen appear to be specifically endocytosed and trafficked to α-granules for subsequent release. Consistently, clathrin-coated pits have been observed budding from the specialized region of the plasma membrane, the OCS.24 P2Y1 and P2Y12 receptor desensitization and resensitization are also thought to occur through an endocytosis, re-expression pathway.25 Though it clearly occurs in vivo, the mechanism, the extent, and the biologic importance of platelet endocytosis are largely unknown and understudied.
therefore, total platelet immunoglobulin is more affected by changes in plasma immunoglobulin levels than by changes in surface immunoglobulin. Other proteins, such as VEGF, factor V, and fibrinogen appear to be specifically endocytosed and trafficked to α-granules for subsequent release. Consistently, clathrin-coated pits have been observed budding from the specialized region of the plasma membrane, the OCS.24 P2Y1 and P2Y12 receptor desensitization and resensitization are also thought to occur through an endocytosis, re-expression pathway.25 Though it clearly occurs in vivo, the mechanism, the extent, and the biologic importance of platelet endocytosis are largely unknown and understudied.
The pathways that regulate α-granule assembly are also not fully understood, but several studies suggest that multivesicular bodies (MVBs) play a crucial intermediary role in α-granule biogenesis.26 These membranous compartments, containing numerous small vesicles, develop from budding vesicles in the Golgi complex within megakaryocytes and can interact with endocytic vesicles. They are abundant in immature megakaryocytes and decrease in numbers with cellular maturation, suggesting that they are the precursors of α-granules and/or dense bodies. MVBs may also function as a sorting hub to route proteins into distinct classes of α-granules.
Lysosomes
Lysosomes are a key component of the endosomal membrane system. Platelet lysosomes contain acid hydrolases (e.g., β-glucuronidase, cathepsins, aryl sulfatase, β-hexosaminidase, β-galactosidase, endoglucosidase [heparitinase], β-glycerophosphatase, elastase, and collagenase).2 Similar to other hematopoietic cells, platelets secrete lysosomes upon activation, but lysosomal release is slower and less complete than that of α- and dense granules.6 Thus, stronger agonists are required to induce lysosomal enzyme release. The elastase and collagenase activities released from platelet lysosomes may contribute to vascular damage at sites of platelet thrombus formation.27 The heparitinase may be able to cleave heparin-like molecules from the surface of endothelial cells, and the resulting soluble molecules appear to inhibit growth of smooth muscle cells.28
Platelet Secretome
Present estimates suggest that fully activated platelets can secrete hundreds of different molecules.4,5,29 While dense granules predominantly contain only small molecules, α-granules contain a myriad cargo that comprises the bulk of the platelet “secretome.” Some of these proteins are produced at the megakaryocyte stage and are packaged into granules during their biosynthesis.26 It is generally thought that patients with GPS have a defect in this process. Other α-granule cargo (e.g., fibrinogen and factor V) are not made by megakaryocytes, but are thought to be endocytosed by circulating platelets.30 Once internalized, these cargo molecules are transported to α-granules.26,31
This “secretome” can be classified by granular source or proposed function.32 Dense granules mainly contain nonpeptide, small molecules: for example, ADP, serotonin, and calcium, which are critical for platelet activation and vasoconstriction. As discussed below, failure to release these components leads to defects in thrombosis. α-Granules contain proteinaceous components which have a wide array of functions.
Functionally, the “secretome” can be divided into even more categories (reviewed in Ref.33). The most obvious class of proteins is the adhesive proteins such as fibrinogen, fibronectin, vitronectin, and thrombospondin that are released from α-granules and function in platelet-platelet cohesion and subsequent thrombus formation. Other proteins, such as P-selectin, are present on the α-granule membrane and are exteriorized to the surface upon granule-plasma membrane fusion. Platelets also release fibrinolytic agents such as PAI-1 and TAFI which are important for clot remodeling. Perhaps the most diverse category is the mitogens such as IGF-1, VEGF, and FGFβbFGF. These proteins are thought to promote wound healing and angiogenesis by stimulating chemotaxis, cell proliferation, and maturation of the cells surrounding the wound site. Finally, platelets release a number of chemokines and cytokines such as RANTES, IL-8, and MIP1α that promote activation of passing leukocytes and lead to a range of immune responses. This releasate catalog suggests that platelet secretion is pivotal in establishing the microenvironment at the wound site. Therefore, controlling release from the granule stores, either specifically or globally, may prove to be an effective strategy to manipulate this microenvironment.
Granule Heterogeneity
It is becoming increasingly clear that our old views of platelet granules may be overly simplistic. Recent studies have suggested that α-granules are heterogeneous in content and that heterogeneity may result in differential release of granule cargo. Italiano and colleagues have shown that α-granules can be distinguished based on the pro- or antiangiogenic factors that they contain.19 Antiangiogenic factors, such as endostatin, reside in different granules than do proangiogenic factors such as VEGF. A similar degree of granule heterogeneity was initially reported by Storrie and colleagues who examined the localization of fibrinogen and vWF.20,21 Functionally relevant granule heterogeneity is seen in other hematopoietic cells such as mast cells and neutrophils. In these cells, differential release in response to distinct agonists appears to be mediated by different SNARE proteins.34,35
The diversity of the platelet releasate suggests that platelet secretion could be pivotal in establishing the microenvironment at an injury site. However, it is unclear whether platelet secretion is a controlled, thematic process or a random event. Several studies found that some angiogenesis regulators are packaged into distinct α-granule populations. Immunofluorescence and immunoelectron microscopy show distinct localizations for pro- and antiangiogenic factors.19 Other studies have also shown that stimulating platelets with different agonists (i.e., PAR1 and PAR4 agonists) causes differential release of certain cargo molecules.19,36,37 This led to the proposal that platelet secretion is contextually thematic, that is, capable of releasing specific sets of cargo (e.g., pro- vs. antiangiogenic factors) in response to specific agonists. Other data suggest that cargo is spatially segregated into subregions of the same membrane-bound granules and that there is no “thematic” colocalization of factors with like functions.20,21 Though these data are intriguing, it is yet difficult to ascertain the extent to which platelet secretion is heterogeneous. If there is heterogeneity in cargo packing and/or secretion, regulating the release of specific α-granule subpopulations may allow
specific manipulation of the microenvironment at an injury site without disturbing hemostasis.
specific manipulation of the microenvironment at an injury site without disturbing hemostasis.
PLATELET SECRETORY MACHINERY
SNARE Overview
Platelet granule-plasma membrane fusion is analogous to exocytosis in neurons where detailed studies have shown the importance of a core set of integral membrane proteins called soluble N-ethylmaleimide Sensitive Factor (NSF) attachment protein receptors (SNAREs)38,39 (FIGURE 32.1). Since the original description of SNAREs in neurons,40 there have been significant advances to our understanding of how SNAREs function. Recent models of SNARE function posit that vesicle/granuletarget membrane fusion is governed, in part, by the matching of a vesicle SNARE (v-SNARE), with a heterodimeric or trimeric, protein complex in the target membrane (t-SNAREs). This transmembrane complex is the minimal element required for membrane fusion.41 v-SNAREs are type II integral membrane proteins of generally low molecular weight (15 to 25 kDa) that contain a characteristic 60 to 70 amino acid SNARE motif.38 At present, approximately 20 different v-SNARE isoforms have been identified in humans. t-SNAREs are of two types: the synaptosomal-associated protein 23/25 (SNAP-23/25) type (SNAP-23, SNAP-25, SNAP-29, Sec9p), and the syntaxin type (syntaxin 1-19, etc.). The SNAP-23-like proteins have two SNARE motifs, lack transmembrane domains (TMDs), and are generally anchored to the membrane through thioester-linked acyl groups attached to a central cysteine-rich domain. The type II membrane protein syntaxins contain the characteristic SNARE motif, an N-terminal regulatory domain, and generally, a C-terminal TMD.42 SNAREs employ the SNARE motifs to form a four-helix bundle arranged as a parallel coiled-coil.43 This structure is stabilized by core hydrophobic interactions running the length of the bundle. Based on this inherent stability, the temporal and spatial control of SNARE complex formation and disassembly represent important tasks for the secretory machinery. Three groups have contributed to our present knowledge of the function of platelet SNAREs (for reviews see Refs.44,45). To date, four vesicle-associated membrane proteins (VAMPs -2, -3, -7, -8), four syntaxins (-2, -4, -7, -11), and SNAP-23, SNAP-25, and SNAP-29 have been detected in platelets.46,47,48,49,50,51,52,53,54
Plateletv-SNAREs
In human and mouse platelets, the v-SNAREs are VAMP-2/synaptobrevin, VAMP-3/cellubrevin, VAMP-7/TI-VAMP, and VAMP-8/endobrevin, with the latter being most abundant46,52,53,54 (FIGURE 32.1). Assigning roles for these v-SNAREs was initially ambiguous, perhaps because in the in vitro exocytosis assays used, the v-SNARE-effecting agents were being added to permeabilized platelets. Flaumenhaft et al.49 found that P-selectin exposure (a metric of α-granule release) was inhibited by pretreatment of permeabilized platelets with Tetanus neurotoxin, a heterodimeric, zinc-dependent, endopeptidase specific for VAMP-1, -2, and -3.55 Subsequently, Feng et al.56 showed that an antipeptide antibody to the divergent, N-terminus of VAMP-3 could inhibit α-granule release, suggesting a role for VAMP-3. However, secretion by platelets from VAMP-3-/- mice was normal.54 In fact, secretion for these platelets seemed faster.53 Polgar et al.52 showed that the cytoplasmic domains of VAMP-3 or VAMP-8, containing the SNARE motif, could inhibit release from dense and α-granules. Since the isolated cytoplasmic domains are promiscuous in their associations in vitro,57,58 it was uncertain whether the inhibitory effects were illustrative of a v-SNARE role or of t-SNARE heterodimer binding preferences.
Ren et al.53 showed that VAMP-8/endobrevin is the primary v-SNARE in mouse platelets. VAMP-8-/- platelets had a clear secretion defect, which was not seen in VAMP-3-/-, VAMP-2+/-, VAMP-2+/-/VAMP-3-/- platelets or from either permeabilized human or VAMP-3-/- platelets that had been treated with the catalytic Tetanus toxin light chain. However, the secretion defect in VAMP-8-/- platelets was not complete. α-granule and lysosome release were significantly affected even at high agonist doses and upon extended incubation, but dense granule release was only attenuated and eventually approached wild-type levels. The VAMP-8-independent release could be eliminated by treatment of permeabilized VAMP-8-/- platelets with Tetanus toxin, suggesting
that the secondary mechanism employed either VAMP-2 or -3. Experiments using permeabilized human platelets treated with Fab fragments prepared from VAMP-8-specific immunoglobulins showed that the role of VAMP-8 in mouse and human platelets is similar (Ren Q and Whiteheart SW, unpublished data, 2008).
that the secondary mechanism employed either VAMP-2 or -3. Experiments using permeabilized human platelets treated with Fab fragments prepared from VAMP-8-specific immunoglobulins showed that the role of VAMP-8 in mouse and human platelets is similar (Ren Q and Whiteheart SW, unpublished data, 2008).
The redundancy in v-SNARE usage is analogous to that seen in chromaffin cells59 where there is a primary v-SNARE (VAMP-2) required for rapid and efficient, agonist-induced release, and a secondary v-SNARE (VAMP-3) which functions, albeit less efficiently, in the absence of the primary v-SNARE. Full ablation of release from chromaffin cells requires deletion of both VAMP-2 and VAMP-3. The redundancy in VAMP usage observed in ex vivo platelet secretion assays is consistent with the in vivo thrombosis phenotype of the VAMP-8-/- mice. Upon laser injury, VAMP-8-/- mice have a delay in thrombus formation and an increase in embolization, although the overall extent of platelet accumulation is not statistically different from wild type.8
In light of the role of VAMP-8 in platelets, it is noteworthy that a SNP (single nucleotide polymorphism) in the human VAMP-8 gene strongly correlates with early onset myocardial infarction (MI).60,61 The authors of these studies suggest that this SNP might affect VAMP-8 expression levels, given its location in the 3′-untranslated region of the gene. It is conceivable that increased amounts of VAMP-8 could increase the likelihood that a platelet could release its granule cargo and thus be hyperthrombotic. Consistent with this possibility, expression of a microRNA controlling VAMP-8 expression correlated with platelet hyperactivity.62 Normal donors, whose platelets were hyperresponsive to epinephrine, showed increased expression of the micro-RNA. Transfection studies suggested that increased VAMP-8 might make platelet hypersecretory, thereby explaining their hyperresponsiveness. Further analysis will be required to determine if VAMP-8 levels are a thrombotic risk factor.
Platelet t-SNAREs
There are two classes of t-SNAREs: the SNAP-23/25/29 type and the syntaxin type (FIGURE 32.1). Human and murine platelets contain syntaxins-2, -4, -7, and -1149,50,63 as well as SNAP-23, -25, and -29.49,50,64 All three SNAP-23/25-like t-SNAREs have been detected in platelets, although SNAP-25 and SNAP-29 levels appear to be low. Experiments introducing specific antibodies, Fab fragments, and inhibitory peptides have demonstrated that SNAP-23 is required for all three granule secretion events.47,48,49,50,52,64,65 SNAP-23 is phosphorylated upon activation64,66 and preliminary data from Karim et al. (manuscript in preparation, 2012) suggest that SNAP-23 might be an important link between platelet activation and membrane fusion. Polgar et al.,64 using ion trap mass spectrometry, showed that human platelet SNAP-23 could be phosphorylated at Ser23/Thr24 and Ser61 after activation by thrombin. In murine mast cells and platelets, SNAP-23 phosphorylation sites are at Ser95 and Ser120.66 SNAP-23 is important for full assembly of fusigenic SNARE complexes and, in mast cells, phosphorylation appears to contribute to that role.66
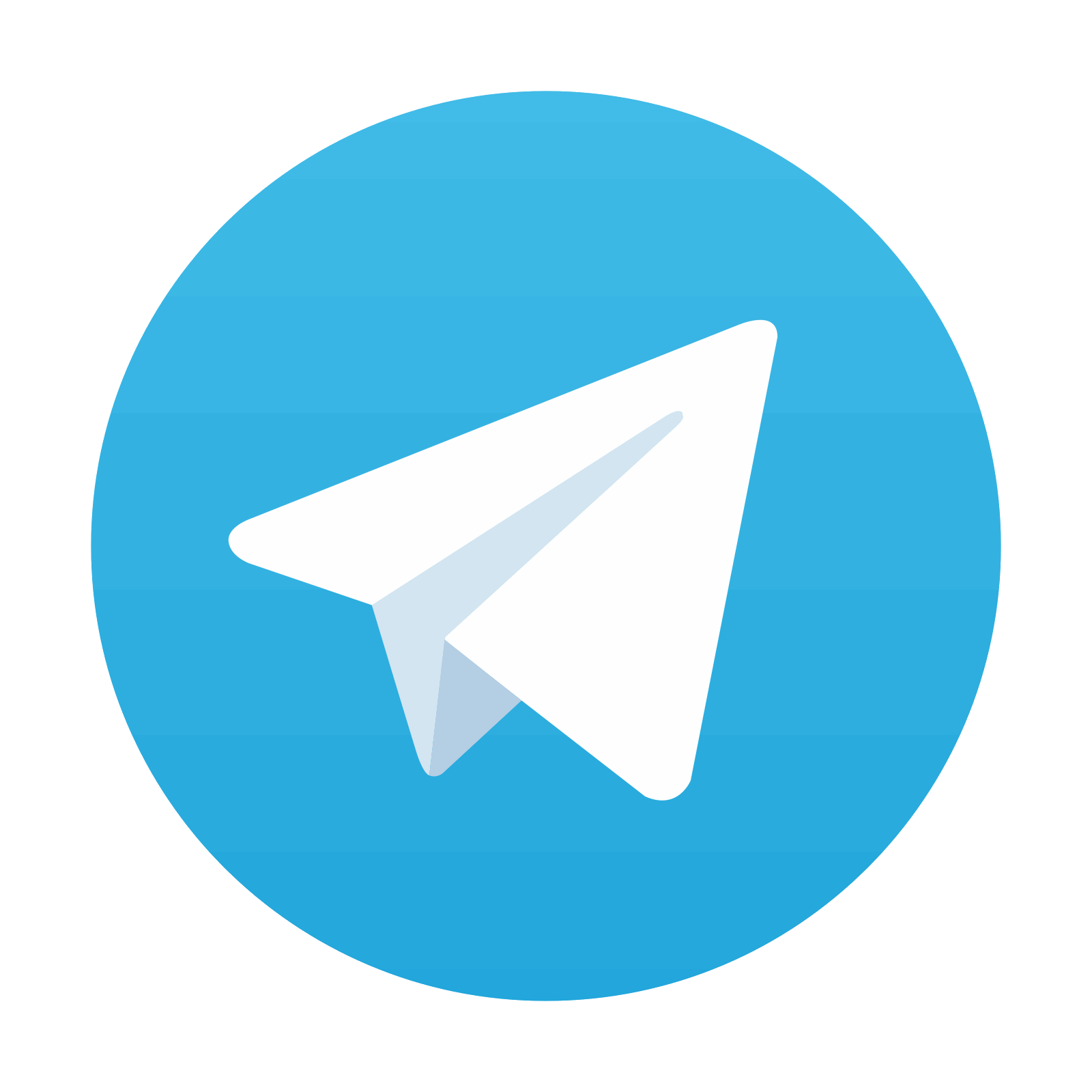
Stay updated, free articles. Join our Telegram channel

Full access? Get Clinical Tree
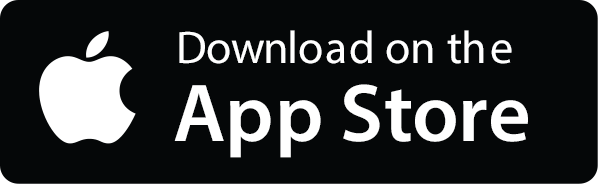
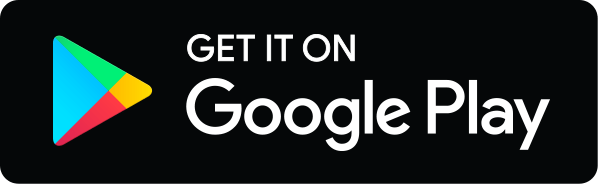
