Imaging and Diagnosis
Imaging is very important in the diagnosis of brain metastases. Although CT scans may be useful in diagnosis, the imaging modality of choice is an MRI as it is more sensitive than CT for determining the number, distribution, and size of lesions.10 The detection of brain metastases may increase after the use of triple dose contrast.11 Typically, brain metastases are solid or ring enhancing lesion(s), pseudospherical in shape, multiple in number, occur in the grey-white junction,12 and occur most frequently in the cerebral hemispheres (80%) followed by the cerebellum (15%) and brain stem (<5%). Hemorrhagic lesions are seen more commonly with germ cell tumors, melanomas, thyroid carcinoma, choriocarcinoma, and renal cell carcinoma. In addition, the leptomeninges can also be involved. The differential diagnosis includes nonmalignant causes such as abscess, infection, demyelination and hemorrhage, and primary brain tumors. If uncertainty exists regarding diagnosis, biopsy or surgery should be performed to confirm diagnosis. For patients with known cancer, restaging scans, which typically include CT scan with and without contrast of the chest, abdomen, and pelvis, should be performed to assess primary and extracranial disease status. The use of fluorodeoxyglucose positron emission tomography alone as the only imaging modality of the brain is not recommended given the high background metabolic use of glucose by the brain.13 After surgery, postoperative MRIs should be done within 48 hours to differentiate tumor recurrence versus blood. For patients undergoing stereotactic radiosurgery (SRS), the MRI changes can be variable with approximately one-third of lesions experiencing a transient increase in tumor volume.14 Using diffusion tensor imaging, regional variation in white matter changes can be seen after WBRT, particularly in the inferior cingulum, corpus callosum, and fornix.15
Recently, the Response Assessment Working Group, an independent, international, collaborative effort, provided suggestions on the design of trials in patients with brain metastases with emphasis on radiographic response.16 In addition, the Response Assessment Working Group provided guidelines on neurocognitive, neurologic, and quality-of-life outcomes for patients with brain metastases.17 The criteria for response rates for patients with brain metastases have been variable between Response Evaluation Criteria in Solid Tumors,18,19 World Health Organization (WHO),20 and those used for high-grade gliomas.21,22
Prognosis
As the overall survival for patients with brain metastases remains poor, the use of prognostic scales helps to guide therapies. One of the earliest and most useful prognostic scales was based on 1,200 patients from three consecutive Radiation Therapy Oncology Group (RTOG) phase 3 brain metastases trials from 1979 to 1993.23 Using recursive partitioning analysis (RPA), three well-defined prognostic groups (RPA class I, II, and III) were identified based on age (<65 or 65 and older), Karnofsky performance status (KPS) of ≥70 or <70, absence or presence of extracranial metastases, and primary tumor status (Fig. 123.1A).
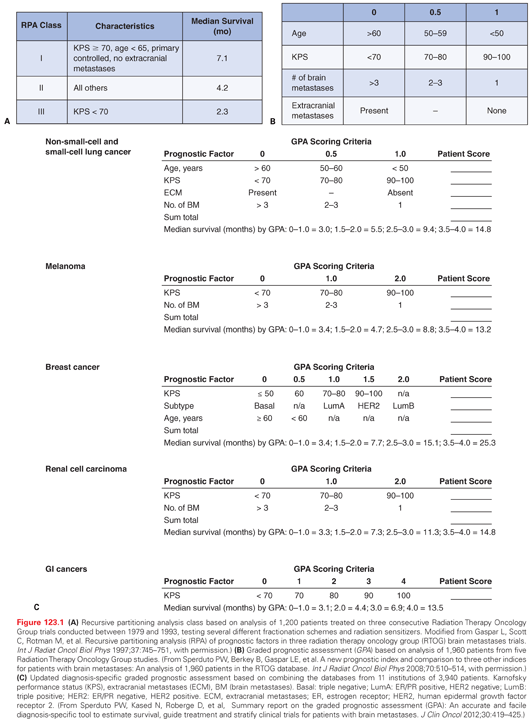
To develop a less subjective and more quantifiable prognostic system, the graded prognostic assessment (GPA) was developed by analyzing data from five RTOG studies.24 The GPA, which is stratified into four prognostic groups, is the sum of scores (0, 0.5, or 1.0) based on age, KPS, presence of extracranial metastases, and number of brain metastases (Fig. 123.1B). Given the heterogeneity of outcomes for patients based on tumor type, an updated diagnosis-specific (DS) GPA was developed by combining the databases from 11 institutions, which was the basis for a summary report on 3,940 patients25 (Fig. 123.1C). Because patients with breast cancer have the best overall survival based on DS-GPA, further stratification incorporates biomarkers such as estrogen receptor (ER), progesterone receptor (PR), and human epidermal growth factor receptor 2 (HER2) status.26 With advances in the understanding of tumor heterogeneity mediated by biomarkers (e.g., B-raf mutations in melanoma; epidermal growth factor receptor mutations in non–small-cell lung cancer [NSCLC]), molecular features will become more important in assessing the prognosis of patients with brain metastases.
An internally validated prognostic nomogram for survival estimates was developed for 2,367 patients based on seven RTOG randomized trials.27 The best-fitting model was the Cox proportional hazards survival model, which included primary site and histology, primary disease status, extracranial spread, age, KPS, and number of lesions. The 12-month survival for each RPA class or DS-GPA group varied.
Symptom Management
Corticosteroids
As brain metastases can cause peritumoral edema, corticosteroids are frequently used to help temporarily control brain edema. Dexamethasone, which has low mineralocorticoid effect, is typically used given its long half-life. Based on a prospective randomized trial of patients who were not in danger of cerebral herniation, higher dose (16 mg/day) demonstrated no advantage compared to lower dose (4 mg or 8 mg/day) dexamethasone.28 Patients receiving higher doses of dexamethasone had more frequent side effects. Common acute side effects may include insomnia, increased appetite, gastritis, fluid retention, mood swings, acne, and elevation of blood sugars. Long-term side effects may include weight gain, facial plethora, pedal edema, immunosuppression, proximal muscle myopathy, cataract formation, aseptic necrosis of the femoral head, and osteoporosis.
Because most brain metastasis trials have not assessed steroid use as endpoints, much controversy exists regarding specific indications and dosing.29 For patients who are neurologically asymptomatic, routine use of corticosteroids is not necessary.30,31 For patients with more severe symptoms from increased intracranial pressure, doses of 16 mg/day or higher should be considered.32 If possible, steroids should be tapered within 1 week of initiation and discontinued within 2 weeks.31 When tapering dexamethasone, it is important to recognize withdrawal symptoms such as headaches, lethargy, weakness, dizziness, anorexia, diffuse arthralgias, and myalgias. To better understand the therapeutic ratio of corticosteroids, future studies should require better control and record keeping of steroid dose for analysis of response to therapy.33
Anticonvulsants
Although seizures are the presenting symptom in 10% of cases and can occur in up to 25% of patients with brain metastases,34 the role of antiepileptic drugs (AED) in patients with brain metastases has very limited class I evidence.35 One study of 100 patients with newly diagnosed brain tumors (60 with brain metastases) and no history of prior seizures randomized patients to AEDs versus no AEDs.36 The primary end point was seizure occurrence at 3 months postrandomization. The trial was terminated early as the seizure rate was only 10%, which was lower than the anticipated rate of 20%.
Given the lack of class I evidence, guidelines on the use of anticonvulsants for patients with metastatic brain tumors have been established.37,38 For patients without seizures, prophylactic use of AEDs is not recommended. Patients who present with seizures or who develop seizures during therapy should receive AEDs, preferably with agents that do not interact with hepatic cytochrome P450 (e.g., levetiracetam). For patients on dexamethasone, phenytoin levels can be affected by its use. In addition, phenytoin can affect clearance of chemotherapy agents and also can cause Stevens Johnson syndrome, which may be precipitated if radiation is used.39 The use of anticonvulsants after surgery is controversial given results of a recent study.40
Venous Thromboembolism
Because patients with brain tumors and thromboembolism are believed to be at higher risk for intracranial hemorrhage with anticoagulation, guidelines were established by the American Society of Clinical Oncology for prophylaxis and treatment of venous thromboembolism in patients with cancer.41 Low-molecular weight heparin is recommended for prophylaxis of hospitalized and higher-risk ambulatory patients given the minimal monitoring of coagulation and rare chance for major bleeding episode.42 Protamine can be used to reverse low-molecular weight heparin in an emergency. For patients with brain tumors and venous thromboembolism, anticoagulation is indicated unless the patient has had an intracerebral bleed or other contraindication for anticoagulation.41 The insertion of an inferior vena cava filter is an alternative but is associated with a higher risk of thrombosis distal to the filter.43
Treatment Options
The optimal management of brain metastases is very controversial given the many factors that influence outcomes, the many modalities available, and the strong opinions that proponents of various treatment modalities have regarding the benefits and risks.44 Even when “experts” are asked to provide guidance on treatment options, the management recommendations can vary widely.45,46 The National Comprehensive Cancer Network guidelines for the treatment of brain metastases are not specific as they allow for physician discretion.47 The guidelines for WBRT, surgery, and radiosurgery for brain metastases by the American Association of Neurological Surgeons and Congress of Neurological Surgeons are more prescriptive, but also allow for flexibility.48–50
Whole-Brain Radiation Therapy
WBRT has been the standard treatment for patients with brain metastases since the initial reports from the 1950s.51 Because WBRT is widely available, is easy to deliver safely and accurately, is effective in providing palliation for the majority of patients, and does not require sophisticated technologies, it has long been considered the gold standard for treatment of brain metastases and represents the most common treatment modality worldwide. Symptomatic improvement has been poorly assessed and is variable. As the response to WBRT is best among patients with small-cell lung cancer, lymphoma, and germ cell tumors, these tumor types are generally excluded from clinical trials due to the relative radiosensitivity of these histologies.
The RTOG has completed numerous phase 3 trials evaluating various fractionation and dose schemes.52–55 These trials have shown that the majority of patients have partial or complete response of their neurologic symptoms, which range from 40% to 70%, and median survival that averages 3 to 5 months. Extended or hypofractionated WBRT has not shown benefit in terms of survival. As result, the most commonly used fractionation schemes in the United States are 30 Gy in 10 fractions or 37.5 Gy in 15 fractions. For patients with poor prognosis, shortened fractionation schemes of 20 Gy in 5 fractions can be used. Based on an international practice survey of 445 individuals, Tsao et al.56 reported that 20 Gy in 5 fractions rather than 30 Gy in 10 fractions was preferred fractionation scheme in Canada and Australia/New Zealand. This practice survey also revealed that 88% chose comfort measures for patients previously treated with WBRT and now presented 6 months later with two to four brain metastases all <4 cm in size with poor performance. Seventy-eight percent would use WBRT alone for two to four brain metastases for patients with uncontrolled extracranial disease and KPS of 70. The Medical Research Council has an ongoing trial (QUARTZ) that randomizes patients with inoperable brain metastases from NSCLC to supportive care with dexamethasone versus WBRT and dexamethasone.
The imaging response rates or stabilization of tumors after WBRT are quite variable and can range from 0% to 71%.57,58 Nieder et al.59,60 studied the CT responses in 108 patients with 336 measurable lesions following WBRT (30 Gy in 10 fractions) and found the overall response rate at up to 3 months was 59% (lesion complete response rate of 24%, partial response rate of 35%).59 The complete response rates varied by histology and were 37% for small cell carcinoma, 35% for breast cancer, 25% for squamous cell carcinoma, 14% for nonbreast adenocarcinoma, 0% for renal cell carcinoma, and 0% for melanoma. Smaller tumor volumes and absence of necrosis influenced complete response rates. Higher response rate was obtained with 40 Gy at 2 Gy per fraction with or without a partial boost to 50 or 60 Gy compared with 30 Gy at 3 Gy per fraction.60
The most common side effects that occur during or shortly following WBRT include hair loss, mild skin erythema, and fatigue. Other side effects may include headache, nausea, vomiting, loss of appetite, change of taste, serous otitis, and somnolence. Secondary to the effect of opposed tangential radiation beams on skin sparing, hair loss is variable and may be permanent in the top and back of the scalp. Skin reactions typically resolve after several weeks, and fatigue usually improves after 2 to 3 months and may respond to methylphenidate. The long-term and frequency of side effects from WBRT have not been well documented as they were discounted given the short survival of these patients. Long-term side effects may include somnolence, fatigue, hearing loss, memory loss, and, in rare cases, dementia.
Two approaches to minimize the neurocognitive effects of WBRT include innovations in radiation delivery and use of medications. RTOG 0933 was a phase 2 trial evaluating hippocampal avoidance–WBRT (HA-WBRT) for 100 evaluable patients with brain metastases.61 As the hippocampus dentate gyrus is thought to house neural stem cells responsible for memory, HA-WBRT using intensity-modulated radiation therapy minimizes dose to this stem cell compartment (<10 Gy). Results from this study demonstrated a 7% decline in delayed recall versus 30% from historical study (p = 0.0003). RTOG 0614 was a phase 3 trial that randomized 554 patients to WBRT (3,750 cGy in 15 fractions) with or without memantine, an N-methyl-D-aspartate receptor antagonist used for patients with mild to moderate Alzheimer disease.62 The memantine arm had significantly longer time to cognitive decline (p = 0.02), lower median decline on Hopkins Verbal Learning Test-Revised (0 in memantine arm versus 2 in placebo arm at 24 weeks; p = 0.059), and fewer patients with decline in Controlled Oral Word Association at 16 weeks (p = 0.004) or Trail Making Test Part A at 24 weeks (p = 0.014). No difference in overall survival and progression-free survival between the two arms was seen. Based on the results of these trials, a phase 3 study with memantine and HA-WBRT is planned.
Prophylactic Cranial Irradiation
Because patients with small-cell lung cancer (SCLC) have a high propensity to develop brain metastases, prophylactic cranial irradiation (PCI) is commonly used to prevent dissemination to the brain especially for patients who achieve a complete response to induction chemotherapy. The Prophylactic Cranial Irradiation Overview Collaborative Group reported the outcomes in seven previously published PCI trials for patients with complete response to systemic therapy and demonstrated a statistically significant survival advantage favoring the patients treated with PCI and significant reduction in the incidence of new brain metastases from 58.6% to 33.3% at 3 years.63 Another meta-analysis of PCI efficacy reviewed 12 randomized trials and included 5 studies that evaluated the role of PCI in patients with SCLC who had a complete response after induction chemotherapy.64 A significant decrease in the incidence of brain metastases was noted when all studies were considered and improvement in survival when PCI was given only to patients who experienced a complete systemic response. A phase 3 international, intergroup study of patients with limited-stage SCLC in complete remission after chemotherapy and thoracic radiotherapy randomized patients to 25 Gy in 10 fractions, 36 Gy in 18 fractions, or 36 Gy in 24 fractions of PCI with the standard dose (25 Gy in 10 fractions) being the recommended fractionation for PCI given the significantly higher risk for mortality seen in the higher dose arms.65 For extensive-stage patients, 286 patients were randomized to WBRT or observation.66 The primary end point was development of symptomatic brain metastases, which was decreased with PCI. In addition, PCI increased overall survival at 1 year from 13.3% to 27.1% (p = 0.003) and 1-year disease-free survival from 14.6% in the observation group to 40.4% in the treatment group (p <0.001).
The cognitive effects of PCI in patients with SCLC were prospectively studied in 300 patients with SCLC controlled systemically who either received PCI or were observed.67 Neurocognitive assessment from baseline and 2 years after PCI revealed no significant difference in cognitive status between the observation and PCI-treated cohorts. Another study demonstrated similar results in 136 patients seen at 1 year posttreatment.68
RTOG 0214 randomized patients with stage III NSCLC without disease progression after treatment with surgery and/or radiation therapy with or without chemotherapy to PCI (30 Gy in 15 fractions) or observation.69 The primary end point of the study was overall survival. Unfortunately, the study closed early secondary to slow accrual. The 1-year overall survival and disease-free survival were not significantly different. The 1-year rates of development of brain metastases were significantly less for PCI at 7.7% versus 18.0% for observation (p = 0.004). Recently, the impact of PCI on self-reported cognitive functioning and Hopkins Verbal Learning Test were analyzed based on the results of RTOG 0214 and RTOG 0212.70 At 6 and 12 months, a significant decline in self-reported cognitive functioning and Hopkins Verbal Learning Test was noted after PCI.
Radiation Sensitizers and Modifiers
Given the poor outcomes with WBRT alone, radiation sensitizers have been explored to improve the therapeutic ratio between tumor and normal tissues. Mehta et al.6 reported on survival and neurologic outcomes in a randomized trial of WBRT (30 Gy in 10 fractions) with or without daily injections of motexafin gadolinium, a redox modulator.6 Based on the results of subset analysis for NSCLC, a confirmatory trial was performed that showed no significant difference in time to neurologic progression.71 Suh et al.72 reported on results of phase 3 randomized trial comparing WBRT (30 Gy in 10 fractions) and supplemental O2 with WBRT following daily injections of RSR-13 (efaproxiral), an allosteric modifier of hemoglobin.72 The phase 3 trial showed no significant difference in overall survival between the arms, although the breast subset appeared to do better. Confirmatory phase 3 trial of 360 women with brain metastases from breast cancer showed no survival improvement with RSR-13 and WBRT.73 A phase 3 trial from the RTOG evaluating thalidomide and WBRT demonstrated no survival benefit over WBRT alone.74
Surgery
Surgery plays an important role for some patients with brain metastases as it can provide immediate and effective relief from symptomatic mass effect and can confirm or establish the diagnosis, which is important for those patients who have unknown primary. In addition, patients with larger tumors (diameter >2 cm) may also benefit from surgery more than other treatment modalities. Advances in imaging and image guidance have improved the rates for complete resection.75 Some of these advances include accurate localization with MRI and CT with stereotaxis, intraoperative imaging with ultrasound, mapping of the important cortices to determine brain function, and fiber tract mapping to avoid damage to important neural pathways to maintain or improve quality of life.
The type of surgical resection also appears to influence the rate of local recurrence. A retrospective review from MD Anderson Cancer Center of 570 patients undergoing surgical resection alone demonstrated that piecemeal resection compared with en bloc resection significantly predicted for higher local recurrence compared to en bloc surgery (hazard ratio = 1.7; p = 0.03).76 Larger tumor volume exceeding 9.71 cm3 compared with smaller tumors also predicted for higher local recurrence (hazard ratio = 1.7; p = 0.02). Histologic subtype did not predict for local recurrence. The rate of leptomeningeal dissemination also appears to be higher after piecemeal rather than en bloc resection of metastases, although larger tumors cannot always be removed without some internal decompression.77,78 A predisposition for leptomeningeal spread is also seen for surgical resection of metastases of the posterior fossa.79 For patients with multiple brain metastases, no strong comparative data exists regarding the role of surgery for these patients,49 but a retrospective report from Bindal et al.80 showed that survival for patients with multiple metastases where all were surgically removed was equivalent to that of surgery for a single removed lesion and superior to those where some lesions were not removed.
Whole-Brain Radiation Therapy with or without Surgery
The first phase 3 study from the University of Kentucky randomized 48 patients with a single brain metastasis to surgical resection and WBRT versus needle biopsy and WBRT (36 Gy in 12 fractions).81 The addition of surgery to WBRT significantly improved survival (40 weeks versus 15 weeks, p < 0.01), local control (80% versus 48%, p < 0.02), and functional independence (38 weeks versus 8 weeks, p < 0.005) compared to biopsy and WBRT. Younger age, no extracranial disease, surgical resection, and longer interval from primary diagnosis to diagnosis of brain metastasis were associated with longer survival. The majority of these patients had NSCLC.
The second phase 3 study from Noordijk et al.82 randomized 63 patients with CT-confirmed brain metastasis to surgical resection and WBRT versus WBRT (40 Gy in 20 fractions). Surgery and WBRT improved median survival (10 months versus 6 months; p = 0.04) and functional independence (7.5 months versus 3.5 months; p = 0.06) over WBRT alone. Patients with active extracranial disease had no survival benefit with surgery (5 months in each arm). Patients without active extracranial disease had significantly improved median survival in the surgery arm (12 months versus 7 months).
Another phase 3 trial from Mintz et al.83 showed no benefit for surgical resection and WBRT (30 Gy in 10 fractions) compared to WBRT alone in 84 randomized patients in terms of survival, 30-day mortality, morbidity, or causes of death. The presence of extracranial metastases was an important predictor of mortality (relative risk = 2.3). Unlike the other two randomized trials, a greater percentage of patients had active systemic disease, had lower baseline KPS, and had several gastrointestinal primary tumors in each group.
Surgery with or without Whole-Brain Radiation Therapy
The role of WBRT following surgical resection was addressed in a phase 3 randomized study of 95 patients who had single metastasis to the brain.84 After gross total resection, patients were randomized to observation versus a protracted course of WBRT (50.4 Gy in 28 fractions). The primary end point was tumor recurrence in the brain with secondary end points of survival, cause of death, and functional independence. The addition of WBRT to surgery decreased the chance for neurologic death (14% versus 44%, p = 0.003), decreased local recurrence (10% versus 46%, p < 0.01), and decreased tumor recurrence anywhere in the brain (18% versus 70%, p < 0.001). The majority of patients (61%) received WBRT at time of recurrence, which resulted in a large crossover of the observation arm to WBRT. Survival was not different, although the study’s end point was local control and was not powered to demonstrate a survival advantage.85
Kocher et al.86 reported the results of a phase 3 European Organisation for Research and Treatment of Cancer study of 359 patients comparing SRS (199 patients) or surgery (160 patients) with or without WBRT.86 The primary end point for this study was time to deterioration of WHO performance status >2. Survival and time to WHO performance status deterioration >2 were not different between the two arms. At 2 years after surgery, WBRT significantly decreased the risk of local and new brain relapse from 59% to 27% (p < 0.001) and 42% to 23% (p < 0.008), respectively.
Due to the previously discussed concerns of the impact of WBRT on NCF, the use of SRS to the tumor bed has recently gained favor despite lack of class I evidence and is discussed later in this chapter.
Stereotactic Radiosurgery
Unlike WBRT, SRS delivers high dose(s) of ionizing radiation to focal target(s) while minimizing the dose to surrounding brain tissue. Although the concept of SRS dates back to the 1950s, this treatment option did not gain acceptance for many years.87 Brain metastases have features that make them ideal targets for SRS, which include pseudospherical shape, location in the gray-white junction, and maximum diameter of <4 cm. In addition, the vast majority of brain metastases have distinct pathologic and radiographic margins, which allows for clear delineation of the target.88 All of these characteristics allow for accurate target delineation, planning, and treatment delivery through the use of multiple intersecting radiation beams, which produce steep dose gradients (“dose falloff”). The linear accelerator, cobalt-60–based units (Gamma Knife; Elekta AB, Stockholm, Sweden), and proton beam unit represent the different types of devices used for SRS. Table 123.2 lists the advantages for SRS and surgery.
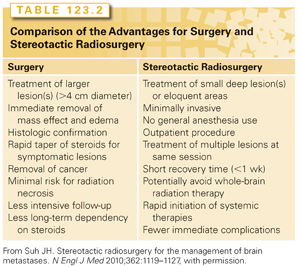
Since the 1990s, numerous institutional and multi-institutional studies have been published on the results of SRS as a primary, adjuvant, and salvage treatment for brain metastases. When prescribing doses of 15 to 24 Gy, the local control rates range from 70% to 95%.89–92 Freedom from local progression depends on dose, volume, histology, and pattern of MRI or CT enhancement at the time of radiosurgery.93,94 In addition, SRS is effective for all types of primary tumor including radioresistant histologies such as melanoma and renal cell carcinoma.95,96 The ideal candidate for SRS is subject of debate, but generally consists of patients with controlled or absence of extracranial metastases and excellent KPS. Historically, the number (one to four) of brain metastases was considered a general contraindication, although recent publications refute this.97–99
To study the relationship between maximum tolerated radiosurgery dose and maximum diameter of the tumor, RTOG 9005 was a phase 1/2 trial for patients with recurrent primary and metastatic brain tumors.100 The maximum tolerated doses for >2 cm to 3 cm and >3 cm to 4 cm were 18 Gy and 15 Gy, respectively; maximum tolerated dose was not reached for tumors ≤2 cm given the reluctance to dose escalate beyond 24 Gy. Because the risk of radiation injury increases with increasing tumor volume and diameter, doses for brain metastases are inversely proportional to the maximum tumor diameter or volume. As a result, the local control rates for smaller targets are generally higher than larger targets, especially when WBRT is not part of the management strategy.101 As the dose falloff is not as sharp for larger targets, which resulted in higher dose to normal brain tissues, radiosurgery targets are usually limited to maximum diameter of 4 cm. Recently, staged radiosurgery with repeat dose 3–4 weeks after initial treatment has been explored prospectively for larger lesions.102
Because the head frame is minimally invasive, there has been growing interest in frameless radiosurgery to allow for fractionation (two to five fractions). This may be helpful for larger tumors and those close to critical structures such as the optic apparatus. The results with frameless radiosurgery appear equivalent to frame-based radiosurgery, although the total number of treated patients is still limited.103–107 For radioresistant tumors, fractionated SRS may be less effective than single-fraction SRS.108
Unlike WBRT, SRS increases the risk for radiation necrosis, which can lead to significant side effects such as steroid dependency. As radiation necrosis can closely mimic tumor recurrence, the diagnosis and treatment of radiation necrosis can be very difficult.109 A recent questionnaire regarding practice patterns for diagnosis and treatment of intracranial radiation necrosis after SRS revealed variation in the management of steroid refractory radiation necrosis.110 Figure 123.2 shows a sequence of images from diagnosis, after SRS, images confirming radiation necrosis, and resolution of radiation necrosis after treatment. Figure 123.3 shows an algorithm that we use to diagnose and treat radiation necrosis. In terms of imaging, cerebral blood volume can have high sensitivity and specificity.111 Based on a double-blind, placebo-controlled phase 3 trial, the use of bevacizumab is the therapy with the best supported evidence for patients who are refractory to steroids.112 One novel approach for treatment of steroid refractory radiation necrosis that is being explored is focused laser interstitial thermal therapy.113
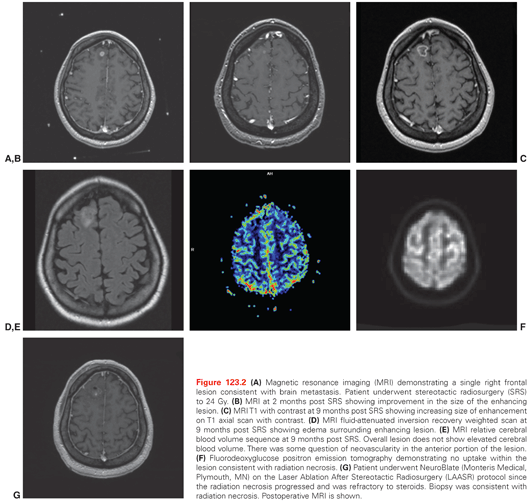
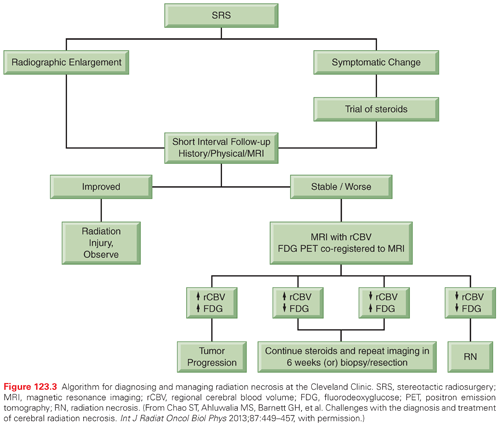
Stereotactic Radiosurgery Versus Surgery
Several attempts at phase 3 trials comparing surgery to SRS have been done with little success. In the 1990s, the Joint Center for Radiation Therapy in Boston attempted to enroll patients onto a phase 3 trial comparing surgery to SRS and enrolled only six patients after 3 years due to patient or physician preference.114 A prospective randomized trial from Germany compared SRS alone versus surgery and WBRT for patients with a single metastasis.115 Unfortunately, this study closed early due to poor accrual with 33 patients in the surgery and WBRT arm, and 31 patients in the SRS alone arm. Overall survival, neurologic death, and local recurrence were equivalent. Shorter hospitalizations, less steroid treatment, and lower central nervous system (CNS) grade 1 and 2 toxicities were noted in the patients treated with SRS. Patients treated with SRS alone developed more distant recurrences (p = 0.04); however, this difference was lost after adjusting for salvage SRS. Another prospective randomized trial of surgery versus radiosurgery for patients with a single brain metastasis who were considered eligible for either treatment was performed at MD Anderson Cancer Center.116 A total of 59 patients were entered into the randomized arm (30 underwent surgery and 29 SRS), and 155 patients were entered into the nonrandomized arm (89 chose surgery and 66 chose SRS). Although the results demonstrated higher local recurrence with SRS alone, the statistical analyses used in this study are controversial. However, as radiosurgery was usually effective, SRS remains their primary management approach with surgery as salvage.117
Whole-Brain Radiotherapy with or without Stereotactic Radiosurgery
The first randomized trial of WBRT (30 Gy in 12 fractions) with or without SRS boost (16 Gy) enrolled functionally independent patients with two to four brain metastases measuring ≤2.5 cm in diameter and >5 mm from the optic chiasm.57 This trial enrolled only 27 patients secondary to early stopping rules. The two arms were well balanced with respect to known prognostic factors such as age, KPS, and extracranial disease status. The local control was 92% in the WBRT+SRS arm versus 0% in the WBRT arm (p = 0.002). Median survival was not significantly different in the two arms (7.5 months for WBRT versus 11 months for WBRT plus radiosurgery; p = 0.22).
The next trial, RTOG 9508, was a phase 3 trial of 333 patients with one to three newly diagnosed brain metastases who were randomized to WBRT (37.5 Gy in 15 fractions) with or without SRS boost using maximum doses from the RTOG 9005 trial.58
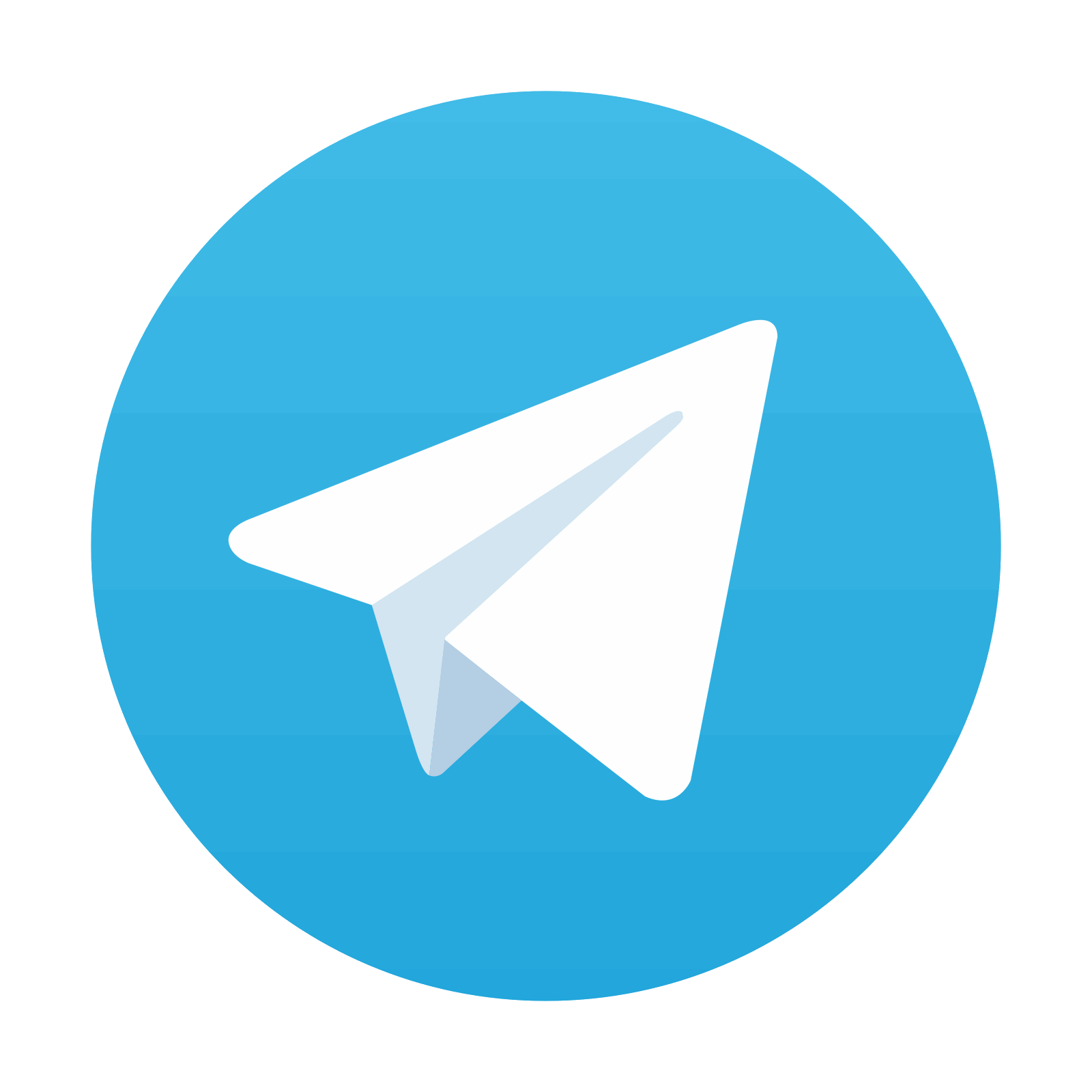
Stay updated, free articles. Join our Telegram channel

Full access? Get Clinical Tree
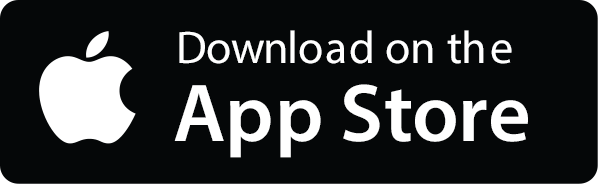
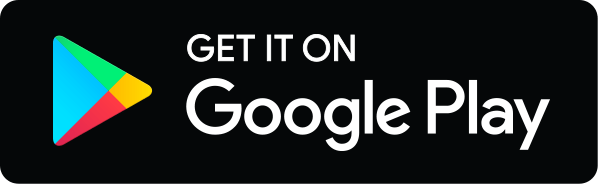