Breast cancer remains a leading cause of morbidity and mortality in women,1 mainly due to the propensity of primary breast tumors to metastasize to regional and distant sites and the failure of effective clinical management of metastatic disease.2,3 Primary therapy for breast cancer usually involves surgical resection of the tumor (lumpectomy or mastectomy), alone or in combination with local radiotherapy. As discussed in later chapters, factors such as tumor size, grade, lymph node involvement, and hormonal status provide valuable information for prognosis. If the patient is felt to have a reasonably high probability of harboring micrometastases, then adjuvant systemic drug therapy (cytotoxic or hormonal) is usually recommended.4 This adjuvant approach has several problems, including both unnecessary treatment of patients who may have been truly cured by their primary treatment alone, as well as the fact that many patients may relapse despite treatment (adjuvant therapy typically only reduces the risk of recurrence by 25-30%).5 A better understanding of the biology of metastasis can improve clinical management and provide the potential for developing novel prognostic and/or therapeutic strategies to combat metastatic breast cancer. This chapter will review what is currently known about the metastatic process, including the timing and steps involved the metastatic cascade, the organ-specific nature of breast cancer metastasis, the contribution of specific molecular factors to metastatic disease, and the issue of tumor dormancy.
The prevailing paradigm of cancer development suggests that tumor cells sequentially accumulate multiple genetic mutations that allow them to evolve from a nonmalignant epithelial cell to a highly aggressive cancer cell, a process called multistep carcinogenesis.6,7 Until recently, it was believed that metastasis was therefore a late event in disease progression, such that additive genetic mutations allowed cancer cells to acquire the capacity to escape from the primary tumor and disseminate to distant organs as a final step in carcinogenesis. Certainly, the clinical reality that patients with large primary breast cancers are more likely to die from metastasis than patients with small tumors8 was believed to reflect the late onset of metastatic spread. However, experimental and clinical observations have challenged this idea, and suggested that metastatic dissemination may in fact be an early event in the overall evolution of breast cancer.9 For example, the observation that metastases can develop in patients with small primary tumors, before the diagnosis of the primary tumor, or even in the absence of a detectable primary tumor (so-called cancers of unknown origin)10,11 indicates that a successful metastatic cell does not necessarily need to undergo a lengthy evolution within the primary site in order to be deadly. Furthermore, recent studies have indicated that the presence of individual tumor cells in the blood or bone marrow of breast cancer patients is an important early indicator of the potential for metastatic disease and poor prognosis.12 Finally, global gene expression analyses of primary breast cancers have identified specific molecular signatures that predict the development of distant metastases,13,14 suggesting that the ability to metastasize can be acquired early in breast cancer development.
Over the past decade or so, significant inroads have been made towards elucidating the physical steps involved in metastasis. It is now widely accepted that, similar to multistep carcinogenesis, the metastatic process is comprised of a complex series of sequential events. In order for clinically relevant metastases to form, cancer cells must successfully complete each of these steps (Fig. 3-1).2,3
Figure 3-1

Metastasis is a complex, multistep process. The metastatic process is comprised of a complex series of sequential events. In order for clinically relevant metastases to form, cancer cells must successfully complete each of these steps. (1) Escape from the primary tumor. (2) Dissemination from the primary tumor via the blood or lymphatic system. (3) Survival within the circulation. (4) Extravasation into a secondary site. (5) Initiation of growth into micrometastases. (6) Maintenance of growth as a vascularized macrometastases. Based on the complexity of the metastatic process, it seems unlikely that all cancer cells would be able to successfully complete all the steps necessary to form macrometastases. Indeed, it is known that metastasis is a highly inefficient process, and that not all the steps of the metastatic process are equally inefficient. The principal rate-limiting steps are initiation of growth into micrometastases (step 5) and maintenance of growth into macrometastases (step 6).
Once a primary breast tumor grows beyond a certain size (~1-2 mm3), there is a requirement for the growth of new blood vessels (angiogenesis) in order to supply the tumor with factors needed for metabolism and continued proliferation.15 Vascularization of the primary tumor enhances the opportunity for tumor cells to enter the bloodstream, a process called intravasation. This requires cell–cell interactions between tumor cells and endothelial cells, which in turn leads to tumor cell adhesion, migration, and invasion through the extracellular matrix into the vasculature.2,16 Since these newly formed blood vessels often lack an intact endothelial cell wall, they can provide a relatively accessible escape route for tumor cells to enter the circulation.17 In addition, cells may also disseminate from the primary tumor through the lymphatic system. However, because there is no direct flow from the lymphatic system to other organs, cancer cells that escape via this route (a common occurrence in breast cancer) must still enter the venous system in order to be distributed to remote parts of the body.2,3,18
Cells that disseminate from the primary tumor and survive the challenges of host anti-tumor immune responses and hemodynamic shear stresses in the circulation are then carried to the capillary beds of secondary organs. Tumor cells are usually arrested by size restriction in the first capillary bed that they encounter, although some may pass through the first capillary bed and travel on to other secondary sites depending on regional blood pressure and deformability of the cells.2,19 Arrested tumor cells can escape from the circulation (extravasate) by invading and migrating back through the endothelial cell wall, through the extracellular matrix, and into the secondary organ. Once in the new site, cells must initiate and maintain growth to form micrometastases, and (as with the primary tumor) these micrometastases require angiogenesis for nutrition and growth in order to grow into macroscopic tumors that are sufficiently large enough to cause physiologic effects on the patient.15
The successful metastatic cell must therefore negotiate a number of different steps, including dissemination from the primary tumor via the blood or lymphatic system, survival within the circulation, extravasation into a secondary site, initiation of growth into micrometastases, and maintenance of growth as a vascularized macrometastases. Considering the onerous nature of this process, it is not surprising that several lines of experimental and clinical evidence indicate that metastasis is inherently inefficient20 Experimental studies have shown that early steps in hematogenous metastasis (ie, survival in the bloodstream, extravasation) are remarkably efficient, with greater that 80% of cells successfully completing the metastatic process to this point. However, only a small subset of these cells (~ 2%, depending on the experimental model) can initiate growth as micrometastases, and an even smaller subset (~ 0.02%, depending on the experimental model) are able to persist and grow into macroscopic tumors.21 It is thus the later steps in metastasis involving growth at the secondary site that have been shown, at least in experimental models, to be highly inefficient. Indeed, these findings are supported by clinical observations that, despite the fact that patients diagnosed with cancer may have hundreds to thousands of single disseminated cancer cells that can be detected in the bloodstream and/or sites remote from the primary tumor, only a very small percentage progress to form overt macrometastases.20,22
Regulation of growth at the secondary site also differs depending on which organ the tumor cells metastasize to, and many cancers show an organ-specific pattern of metastasis. It is well established that breast cancer favors metastasis to regional lymph nodes, lung, liver, bone, and brain (Fig. 3-2).2,3 From a pathohistologic point of view, breast tumor morphology from one metastatic site to the next can be very similar, and is often used as evidence of probable common origin when of the same morphology and grade as the primary breast tumor. It is believed that there are several factors that influence the organ-specific nature of breast cancer metastasis, and these are discussed next.
Figure 3-2


Breast cancer has “favorite” sites of metastasis. It is well established that breast cancer favors metastasis to specific organs including lymph node, lung, liver, bone, and brain. In breast cancer patients, pathohistologic analysis of hematoxylin and eosin (H&E) stained tissues from regional or distant organ sites can reveal the presence of metastatic tumor cells and provide a basis for prognosis and treatment decisions. A. Breast cancer metastasis to regional lymph node. In this patient, the metastatic breast tumor (T) has almost entirely occupied the node, with only a small amount of normal lymph node architecture (LN) still in evidence. B. Breast cancer metastasis to lung. In this patient, numerous nests of breast tumor cells (T) are evident in the lung parenchyma and invading into the pleura. It is believed that there are several factors that influence the organ-specific nature of breast cancer metastasis, including circulatory patterns, mechanical arrest, tumor cell homing, and the host tissue microenvironment. (Images courtesy of Dr. Alan B. Tuck, London Health Sciences Centre Pathology Department, London, Ontario.)
The movement of tumor cells within and between secondary organs is not random; rather, it depends to a large extent on the location of the primary tumor relative to the body’s natural pattern of blood flow. For example, tumor cells that enter the circulation from most parts of the body (breast, liver, bone) are carried by the systemic venous system directly to the heart and then circulated to all organs of the body via the systemic arterial system. In contrast, cells entering the bloodstream from splanchnic organs such as the colon are circulated first through the liver via the hepatic portal vein, and then into the venous system.16,22 In the 1920s, James Ewing proposed that blood flow patterns alone were sufficient to account for both the physical delivery of tumor cells to secondary organs and for patterns of organ-specific metastasis.23 However, several theories have challenged this idea by proposing that there are additional, molecular-level mechanisms that explain why and how cancer cells can arrest and grow in “favorite” metastatic sites.
The homing theory proposes that different organs produce chemoattractant factors, which can direct specific types of tumor cells to “home” to and arrest in a particular organ.24 The most well-characterized of these molecules is the chemokine stromal-derived factor 1 (SDF-1) and its receptor CXCR4. In normal physiologic states, the SDF-1/CXCR4 axis is used for directed migration of several cell types, including stem cells, immune cells, and neurons.25 SDF-1 is an ideal candidate for aiding in metastasis because of its ability to induce motility, homing responses, adhesion, and secretion of proteases and angiogenic factors in cells that express the CXCR4 receptor. Interestingly, it has been observed that CXCR4 is highly expressed in malignant breast cancer but not normal breast tissue, and that its ligand SDF-1 is expressed in organs to which breast cancer cells often metastasize (lymph node, lung, and bone).26 In addition to chemokines, it has also been observed that endothelial cells forming the capillary beds in various organs can express a unique complement of cell surface molecules that serve as specific “homing addresses” or “vascular zip codes” for facilitating organ-specific metastasis. These molecules may offer specialized docking sites for breast cancer cells that express certain adhesion molecules on their surface, such as the integrin αvβ3.27
Another theory that has gained favor in recent years is the idea that epithelial–mesenchymal transition (EMT) can contribute to the metastatic process. First identified in embryonic development, EMT involves conversion of epithelial cells to a mesenchymal phenotype via loss of polarity, cell–cell contacts, and dramatic cytoskeletal remodeling.28 Cells undergoing EMT also acquire expression of mesenchymal proteins and develop an enhanced ability to migrate, thus assisting in cell distribution throughout the embryo and organ development. In metastasis, it is believed that epithelial tumor cells may be able to somehow activate this primitive developmental program, thus converting differentiated epithelial cancer cells into de-differentiated cells that possess more mesenchymal characteristics. The EMT phenotype in cancer has been associated with increased resistance to apoptosis, increased motility and invasiveness, and enhanced metastatic ability.29 These phenotypic transitions are reversible, and it is hypothesized that once tumor cells have reached their destination, they may transform back into an epithelial phenotype (mesenchymal-to-epithelial transition; MET) in order to facilitate optimal tumor growth in the secondary site.
Finally, the most central of metastasis theories is the “seed and soil” hypothesis, first proposed in 1889 by Stephen Paget.30 Paget predicted that a cancer cell (the “seed”) can survive and proliferate only in secondary sites (the “soil”) that produce growth factors appropriate to that type of cell, and this theory has largely withstood the test of time.31 In a meta-analysis of published autopsy study data, Weiss showed that, in many cases, metastases detected at autopsy were in proportion to the blood flow from the primary tumor site to the secondary organ.22 However, in some cases, more metastases (notably breast cancer metastasis to bone) or fewer metastases were detected than would be expected by blood flow alone, indicating that the “soil” or microenvironment in the secondary organ is likely very important. In addition to mediating growth of tumor cells, it has been suggested that microenvironmental factors in the secondary site can also have significant influence on supporting tumor dormancy through suppression of immune mechanisms, angiogenesis, and alteration of growth-related signaling pathways32 (discussed further below). Thus, the role of the microenvironment in the metastatic process is significant, particularly for the initiation/maintenance of metastatic growth and perhaps for the persistence of tumor dormancy within a secondary site.
It is unlikely that all of these theories are mutually exclusive; rather, the increasing number of molecular players that are thought to be involved in the metastatic cascade (Table 3-1) instead suggests that these various factors cooperate with mechanical influences such as blood flow in order to contribute to the ability of breast cancer cells to establish themselves as metastases in various target organs.
Type of Molecule | Example(s) | Function in Metastasis | Targeted Therapies |
---|---|---|---|
Oncogenic proteins | Ras | Survival and growth at the metastatic site | Farnesyltransferase inhibitors (FTIs): Tipifarniba Lonafarniba |
Growth factors/receptors | EGF/EGFR | Angiogenesis, motility, survival, growth | Trastuzumabb Lapatiniba Bevacizumabb Sunitiniba Sorafeniba |
VEGF/VEGFR | |||
Integrins | αvβ3 | Motility, invasion, growth | Vitaxina Etaracizumaba |
Chemokines/cytokines | CXCR4 | Adhesion, motility, invasion, growth, survival, angiogenesis | AMD3100a,c |
OPN | ?? | ||
Proteases | MMPs | Adhesion, motility, invasion, survival, organ-specific metastasis, growth | MMP inhibitorsc |
Metastasis suppressors | NM23 | Initiation and maintenance of growth at the metastatic site | MPAc |
Treatment for metastatic breast cancer has traditionally consisted of hormonal therapies and broad-spectrum chemotherapeutics. An improved understanding of cancer biology and the development of new technologies has led to a new generation of targeted agents directed against molecular factors involved in breast cancer progression and metastasis. A number of metastatic cell behaviors (angiogenesis, adhesion, migration, invasion, and growth) also play an important role in tightly regulated normal biological processes such as reproduction, embryogenesis, and wound healing. The fundamental difference between normal cells and cancer cells is thought to lie in their ability to be regulated at the biochemical level: in cancer cells, the molecules that start, maintain, or stop these cellular processes are often expressed at inappropriate levels or at an inappropriate time and place.7 A growing number of molecular factors have been identified as being dysregulated during metastasis.33,34 Furthermore, the metastatic process requires the coordinated interplay of a variety of cell types, including tumor cells, endothelial cells, stromal cells, and immune cells. These critical cell–cell and cell–matrix communications are regulated by pro-metastatic and anti-metastatic molecular signals that facilitate the successful completion of the various steps of metastasis (Table 3-1). A selection of these factors and their potential as therapeutic targets are discussed below.
Metastasis-promoting factors can contribute to the acquisition of a metastatic cellular phenotype through increased expression and/or activation, usually as result of abnormal regulation. These include a variety of different protein types, such as oncogenic proteins, growth and angiogenic factors, integrin receptors, chemoattractant molecules, and enzymes that degrade the extracellular matrix.
Oncogenes code for proteins that are important for the regulation of cellular growth control and responsiveness to extracellular signals. When these control mechanisms are constitutively up-regulated in the absence of external growth signals, the uncontrolled proliferation that is typical of cancer cells can occur.7 Members of the Ras oncogene family provide an example of how activated oncogenes can lead to phenotypic changes that promote metastasis.35 Ras oncogenes encode a family of membrane-bound proteins that act as cellular transducers, relaying signals from the cell surface to the cytoplasm, which in turn activate signaling cascades that regulate the expression of a number of different genes. In addition to activating the Ras/Raf/ERK-MAPK (extracellular signal-regulated kinase/mitogen-activated protein kinase) pathway, Ras is also involved in activation other signaling pathways, such as the phosphatidylinositol 3-kinase (PI3K) pathway. Oncogenes such as Ras thus have the potential to contribute to all steps in the metastatic process via activation of angiogenic factors, adhesion molecules, proteases, growth-related genes, and/or survival factors.
Breast cancers have been reported to have a very low (< 2%) incidence of Ras mutation; however, dysregulation of Ras and its associated signaling pathways is believed to be common.36 The rate-limiting step in Ras activation is farnesylation by farnesyl protein transferase. A class of drugs called protein farnesyltransferase inhibitors (FTIs; tipifarnib and lonafarnib) are potent inhibitors of farnesyl transferase and have shown efficacy in preclinical models of breast cancer. Clinically, phase I/II trials in breast cancer patients have also shown encouraging results with FTIs, particularly as combination therapy with taxane-based chemotherapies and endocrine therapy.36,37 Although the particular step(s) of metastasis that are influenced by oncogenes remain to be fully elucidated, studies have demonstrated that Ras activation is important for regulating the ability of tumor cells to initiate and maintain growth of micrometastases once they have arrived at the secondary site.35 It has been observed that the balance between proliferation and apoptosis can be tipped in favor of growth in micrometastases with activated Ras, and in favor of death in Ras-negative micrometastases, suggesting that disruption of the proliferation- to- apoptosis equilibrium to favor progressive growth is one mechanism by which oncogenic signaling can influence metastatic potential.
A number of growth/angiogenic factors and their cognate receptors have been identified as important contributors to breast cancer metastasis. The best characterized of these include members of the epidermal growth factor receptor (EGFR) family (most notably ErbB-2/HER-2), and vascular endothelial growth factors (VEGF) and their receptors (VEGFR).
The EGFR family is comprised of four closely related tyrosine kinase growth factor receptors: EGFR (ErbB-1), HER-2 (ErbB-2), HER-3 (ErbB-3), and HER-4 (ErbB-4). These receptors bind growth factor ligands such as epidermal growth factor (EGF) and transforming growth factor α (TGF α). After ligand binding, the receptor is activated by phosphorylation, which in turn initiates a cascade of downstream intracellular events via the Ras/Raf/MAPK signaling pathway. The EGFR family functionally contributes to a variety of processes that are crucial to metastasis, including cytoskeletal reorganization, adhesion, motility, invasion, angiogenesis, survival, and growth.38
In breast cancer, HER-2 has been found to be an important prognostic and predictive factor both in the adjuvant and metastatic settings. HER-2 is amplified/overexpressed in 20% to 25% of breast cancers, and is associated with aggressive disease and poor prognosis.39 The HER-2 targeting monoclonal antibody trastuzumab (Herceptin) is approved for the first-line treatment of HER2-positive metastatic breast cancer in combination with chemotherapy, and provides a clear advantage in improving clinical outcome.40,41 Trastuzumab is also approved for use as a single agent in patients who have received at least one prior chemotherapy regimen, and has shown promise in phase III investigation for use in the adjuvant and neoadjuvant settings.42 Overall, trastuzumab and other emerging HER-2 agents such as lapatinib (which also targets EGFR) provide an exciting opportunity to provide tailored therapy and change the standard of care for this patient subset.
Another example of important growth factor/receptor pathways in breast cancer is the VEGF/VEGFR family. Through paracrine and autocrine interactions with endothelial cells, tumor cells, and immune cells, VEGF and VEGFR have been shown to play a variety of roles in the tumor microenvironment, including stimulation of angiogenesis, protection from host anti-tumor immune responses, and promotion of tumor and endothelial cell survival/growth. VEGF gene expression can be regulated by a number of stimuli, including oncogenes, tumor suppressors, other growth factors (eg, HER-2), and tumor hypoxia.15,43 The expression of VEGF has been shown to be up-regulated in a number of different human tumor types, and has been correlated with poor prognosis in breast cancer. Studies have also indicated that there is a correlation between high VEGF levels and lack of response to radiation or chemotherapy.44,45 The monoclonal antibody therapy bevacizumab directly targets VEGF and has recently been approved for first-line treatment of metastatic breast cancer in combination with chemotherapy. In addition, other antiangiogenic small-molecule inhibitors targeting VEGFR are being investigated in phase II/III breast cancer trials, including sunitinib, sorafenib, and several others.43 Because of their central role in angiogenesis, VEGF and VEGFR are therefore promising therapeutic targets in breast cancer.
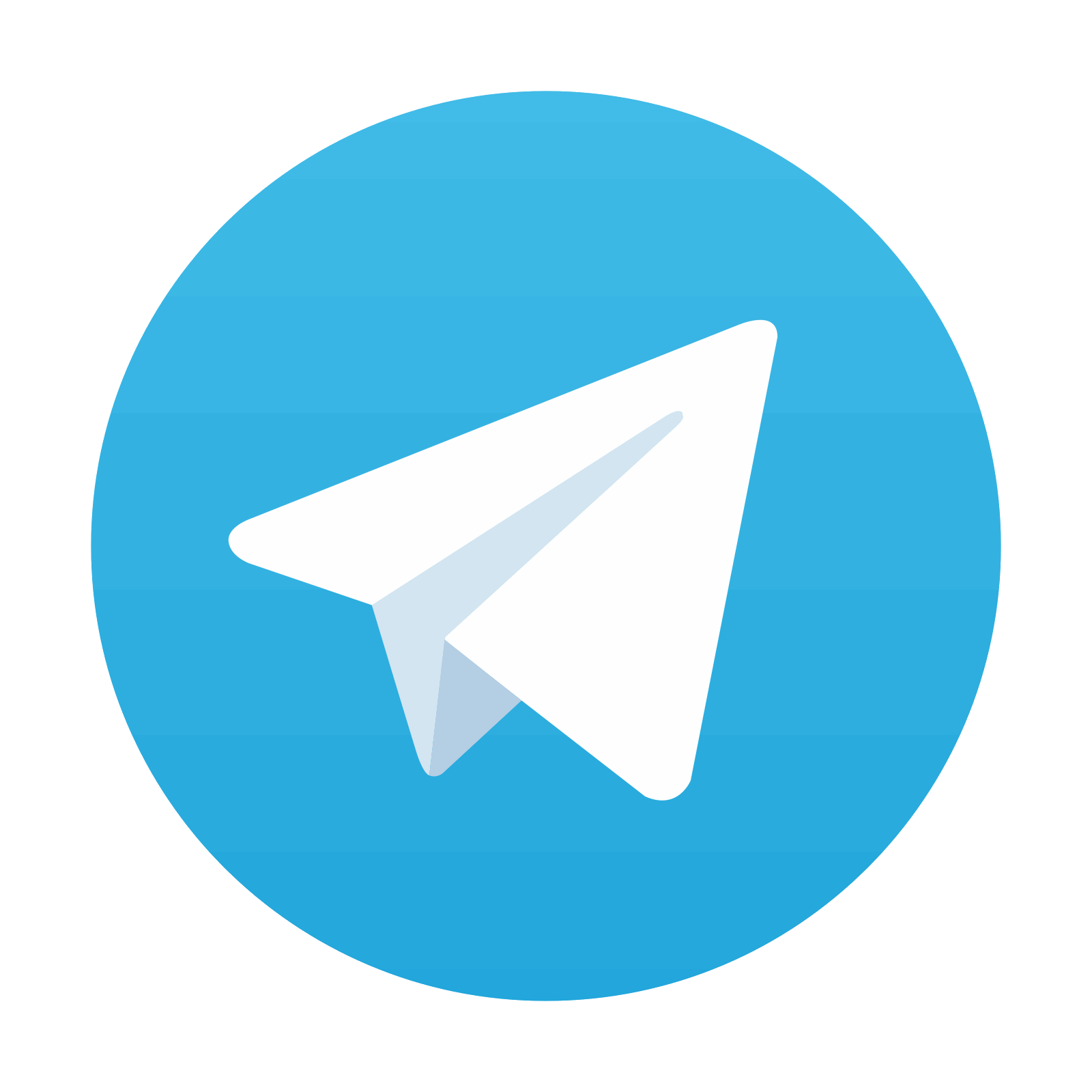
Stay updated, free articles. Join our Telegram channel

Full access? Get Clinical Tree
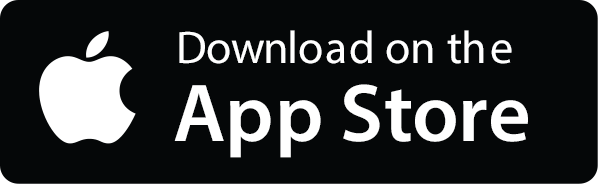
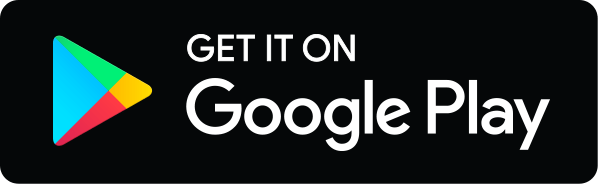