Management of Acute Severe Asthma
Thomas Corbridge
Susan Corbridge
Acute severe asthma (ASA) all too commonly results from inadequately controlled asthma—particularly in the subgroup of patients that depends on emergency-oriented management and under uses inhaled corticosteroids (1). Each year in the United States, acute asthma accounts for approximately 1.8 million emergency department (ED) visits, 480,000 hospitalizations, and 4,000 deaths, most of which occur in the outpatient setting (2,3). While these statistics have improved recently, a gender and racial gap exists with women and African Americans at greatest risk for hospitalization and death (4).
It follows that management of an acutely ill asthmatic involves not only treatment of the exacerbation but also prevention of future attacks by educational efforts, initiation of controller therapy, and scheduling follow-up appointments with an asthma specialist (5). The focus of this chapter, however, is on the more immediate concern of improving airflow rates through the appropriate use of pharmacologic therapy, and, when required, the use of nonpharmacologic means to support ventilation. Fortunately, β-agonist bronchodilators with or without anticholingerics and systemic corticosteroids are sufficient in most cases. For patients with acute respiratory failure requiring intubation, a strategy that minimizes lung hyperinflation improves outcome (6,7).
Pathophysiology of Acute Airflow Obstruction
The speed with which ASA develops varies (8). In sudden asphyxic asthma, severe obstruction develops in less than 3 hours from a more pure form of smooth muscle-mediated bronchospasm. Patients have fewer airway secretions and may respond quickly to bronchodilators alone (9,10). Triggers of sudden attacks include medications such as nonsteroidal anti-inflammatory agents and β blockers in susceptible patients, allergen or irritant exposure, sulphites, and inhalation of illicit drugs (11). Respiratory tract infection is not common; often no cause is identified (12).
Most attacks evolve over 24 hours with progressive airway wall inflammation, accumulations of thick intra-luminal mucus, and bronchospasm. Mucus obstructs large and small airways and consists of sloughed epithelial cells, eosinophils, fibrin, and other serum components that have leaked through the denuded airway epithelium. These types of exacerbations are triggered by a variety of infectious, allergic, and nonspecific irritant exposures and take longer to resolve. Importantly, they epitomize clear and missed opportunities to increase anti-inflammatory medications in the outpatient setting.
The critical endpoint of ASA, regardless of tempo, is expiratory airflow obstruction. It takes longer to exhale through bronchospastic, inflamed, and mucus-filled airways. Indeed it may take as long as 60 seconds for termination of expiratory flow during tidal breathing, but, of course, with respiratory rates in the 20s or 30s, there are only 1 to 2 seconds available for expiration. The result is incomplete emptying of gas and dynamic lung hyperinflation (DHI). Fortunately, DHI may be self-limiting because hyperinflation increases lung elastic recoil pressure and airway diameter, which improves expiratory flow.
At the end of exhalation, incomplete gas emptying elevates alveolar volume and pressure, a state referred to as auto–positive end-expiratory pressure (auto-PEEP). Auto-PEEP is a threshold pressure that must be overcome during inhalation which increases inspiratory work of breathing. At the same time diaphragm force generation is diminished by the adverse effects of DHI and possible respiratory acidosis to create an imbalance between strength and load that predisposes to hypercapneic respiratory failure (13).
Airway obstruction further decreases ventilation (V) relative to perfusion (Q) in alveolar-capillary units, resulting in hypoxemia (14). Because this is not shunt
physiology as is seen in alveolar filling processes, supplemental oxygen typically corrects hypoxemia, even in severely affected patients. Refractory hypoxemia is rare and suggests other problems such as pneumonia, aspiration, atelectasis, or pneumothorax.
physiology as is seen in alveolar filling processes, supplemental oxygen typically corrects hypoxemia, even in severely affected patients. Refractory hypoxemia is rare and suggests other problems such as pneumonia, aspiration, atelectasis, or pneumothorax.
There is a rough correlation between severity of airflow obstruction as measured by spirometry and hypoxemia. However, there are no cut-off values that accurately predict hypoxemia. Furthermore, hypoxemia may occur sooner and/or resolve later than spirometric measures of airflow (15).
Cardiovascular complications of ASA include accentuation of the normal inspiratory reduction in stroke volume and blood pressure termed the pulsus paradoxus (PP). Wide swings in pleural pressure during labored breathing are responsible for this phenomenon. During vigorous inspiration to overcome the effects of auto-PEEP, intra-thoracic pressure falls and blood flow increases to the right heart. The right ventricle fills early in inspiration and shifts the intra-ventricular septum leftward causing diastolic dysfunction of the left ventricle (LV) and incomplete LV filling. Large negative pleural pressures further impair LV emptying by increasing LV afterload (16). Rarely, these effects cause pulmonary edema. During forced exhalation, however, positive intra-thoracic pressure decreases venous return to the right heart setting up the cyclical changes in venous return that underlie the development of PP. Further complicating the cardiovascular response to asthma is the potential for lung hyperinflation to increase pulmonary vascular resistance and pulmonary artery pressures (17). A widened PP can be a valuable sign indicating asthma severity. However, the absence of a widened PP does not ensure a mild attack because PP decreases in the fatiguing patient unable to generate large swings in pleural pressure.
Clinical Presentation, Differential Diagnosis, and Severity Assessment
Analysis of multiple factors, including the medical history, physical examination, objective measures of airflow obstruction, initial response to therapy, arterial blood gases, and chest radiography is all important in the assessment of acutely ill patients (18). Risk factors for asthma-related death including prior intubation should be identified. (Table 21.1) (11,19,20).
Table 21.1 Risk Factors for Fatal or Near-fatal Severe Asthma | |||||||||||||||
---|---|---|---|---|---|---|---|---|---|---|---|---|---|---|---|
|
Differential Diagnosis
“All that wheezes is not asthma” is a fitting clinical saw worth considering during the initial evaluation. An extensive smoking history suggests chronic obstructive pulmonary disease and a more fixed form of expiratory airflow obstruction. Congestive heart failure may present with wheezing (termed cardiac asthma) that responds to bronchodilators (21). However, heart failure is typically associated with an enlarged cardiac silhouette, vascular redistribution, interstitial or pulmonary edema, and small lung volumes. Regardless of concerns regarding heart failure, myocardial ischemia should be considered in patients at risk for coronary artery disease, particularly those receiving high-dose bronchodilators. Moreover, ASA alone can trigger an imbalance between myocardial oxygen supply and demand (22).
Foreign body obstruction occasionally mimics asthma. It should be considered in the very young and old, in patients with altered mental status or neuromuscular disease, and when symptoms occur after eating or dental work. Localized wheeze and, rarely, asymmetric hyperinflation on chest radiography are clues to foreign body aspiration.
Upper airway obstruction including vocal cord dysfunction (VCD) should also be considered in the differential diagnosis of ASA. In contrast to asthma, classic upper airway (extra-thoracic) obstruction flattens the inspiratory portion of the flow-volume loop. Fiberoptic laryngoscopy should be considered when VCD is suspected because of normal oxygenation, lack of response to bronchodilators, normal airway pressures after prior intubation, or stridor to confirm paradoxical vocal cord movement (23). Significant response to helium-oxygen mixtures (heliox) also suggests upper airway obstruction, although heliox response likely occurs in some asthmatics and should not be used to distinguish upper
from lower airway obstruction. In cases of suspected tracheal stenosis (e.g., from prior intubation), fiberoptic bronchoscopy, or spiral computed tomography (CT) are the tests of choice.
from lower airway obstruction. In cases of suspected tracheal stenosis (e.g., from prior intubation), fiberoptic bronchoscopy, or spiral computed tomography (CT) are the tests of choice.
Pneumonia complicating asthma is unusual, but it should be considered in patients with fever, localizing signs, and refractory hypoxemia. Antibiotics are frequently prescribed for asthmatics with increased sputum production alone. Antibiotics have not been shown to improve outcome and should be reserved for treatment of concurrent sinusitis or pneumonia (19,24).
Wheezing occurs rarely in pulmonary embolism (25). When dyspnea is out of proportion to objective findings, particularly the peak expiratory flow rate (PEFR), consider evaluation with chest CT angiography, ventilation-perfusion imaging, d-dimer analysis, or lower extremity Doppler ultrasonography.
Physical Examination
The general appearance of the patient (posture, speech, positioning, and alertness) provides a quick guide to severity, response to therapy, and need for intubation. Patients assuming the upright position have a higher heart rate, respiratory rate, and pulsus paradoxus, and a significantly lower partial pressure of arterial oxygen (PaO2) and PEFR than patients who are able to lie supine (26). Diaphoresis is associated with an even lower PEFR. Accessory muscle use and a widened PP indicate severe asthma; however, their absence does not exclude a severe attack (27). Depressed mental status suggests impending arrest and is an indication for intubation (19).
Examination of the head and neck helps identify barotrauma (crepitus) and upper airway obstruction (prolongation of inspiration and stridor). Tracheal deviation, asymmetric breath sounds, mediastinal crunch, and subcutaneous emphysema variably suggest pneumomediastinum, pneumothorax, or atelectasis. The mouth and neck should be inspected quickly for mass lesions, signs of previous surgery, and angioedema.
Chest auscultation typically reveals expiratory phase prolongation and wheeze. However, wheeze is not a reliable indicator of the severity of airflow obstruction (28). A silent chest indicates severe obstruction and impending respiratory arrest. (In this situation emerging wheeze signals improvement.) Localized wheeze or crackles may represent mucus plugging and atelectasis, but should prompt consideration of pneumonia, pneumothorax, endobronchial lesions, or foreign body.
Tachycardia is common (29). Heart rate generally decreases in improving patients, but some patients remain tachycardic despite clinical improvement because of medications. The usual rhythm is sinus tachycardia but supraventricular and ventricular arrhythmias occur; bradycardia is an ominous sign of imminent arrest (19).
Measurement of Airflow Obstruction
Measuring PEFR or forced expiratory volume in 1 second (FEV1) helps assess the severity of airflow obstruction. Objective measures are important because physician estimates of severity are often wrong—with errors equally distributed between over- and underestimates of the actual PEFR. However, PEFRs are not always obtainable in severely dyspneic patients. Indeed it is wiser to defer measurements in the sickest subgroup because they rarely alter initial management and performing a PEFR maneuver may worsen bronchospasm even to the point of respiratory arrest (30).
According to the Expert Panel Report 3 of the National Institutes of Health, mild attacks are characterized by PEFR >70% of predicted or personal best, moderate attacks by PEFRs between 40% to 69%, severe attacks by PEFR <40%, and life-threatening attacks by PEFR <25% (19). Measuring the change in PEFR or FEV1 is one of the best ways to predict the need for hospitalization. Several studies have demonstrated that inconsequential changes or deterioration after 30 to 60 minutes of therapy predicts a more severe course and need for hospitalization (31).
Arterial Blood Gases
In patients with severe or life-threatening ASA, arterial blood gas analysis demonstrates the degree of hypoxemia and allows for acid-base analysis. In the early stages of ASA, mild hypoxemia and respiratory alkalosis are common. As the severity of airflow obstruction increases, PaCO2 generally increases so that eucapnia and hypercapnia are worrisome findings; however, hypercapnia alone is not an indication for intubation as many of these patients respond adequately to pharmacotherapy (32). Conversely, the absence of hypercapnia does not rule out a life-threatening attack (33).
Patients with persistent respiratory alkalosis compensate by wasting serum bicarbonate which manifests later as a normal anion gap metabolic acidosis (referred to as post hypocapneic metabolic acidosis). Metabolic acidosis with an elevated anion gap may stem from lactic acidosis secondary to increased work of breathing, tissue hypoxia, or intracellular alkalosis. Lactic acidosis indicates a severe exacerbation and occurs more commonly in men and in patients receiving parenteral β agonists (34).
Serial blood gases are
not necessary to determine clinical course. In most cases, valid judgments follow serial examinations with attention to patient posture, accessory muscle use, diaphoresis, chest auscultation, pulse oximetry, and PEFR measurements. Patients who deteriorate on these grounds are candidates for intubation regardless of PaCO2. Conversely, patients improving by multifactorial assessment should not be intubated despite hypercapnia. Serial blood gases are required in mechanically ventilated patients to guide ventilator management.
not necessary to determine clinical course. In most cases, valid judgments follow serial examinations with attention to patient posture, accessory muscle use, diaphoresis, chest auscultation, pulse oximetry, and PEFR measurements. Patients who deteriorate on these grounds are candidates for intubation regardless of PaCO2. Conversely, patients improving by multifactorial assessment should not be intubated despite hypercapnia. Serial blood gases are required in mechanically ventilated patients to guide ventilator management.
Chest Radiography
In classic cases of ASA, chest X-rays rarely alter management (35). In one study (36) reporting radiographic abnormalities in 34% of cases, the majority of findings were likely attributable to common asthma features of airway wall thickening and intraluminal mucus. Chest radiography is thus best reserved for cases with localizing signs or symptoms and when the diagnosis is in question. In mechanically ventilated patients, chest radiography further confirms proper endotracheal tube position.
Emergency Department Management
Patients with mild to moderate attacks responding well to initial therapy may be considered for discharge. Observation in the ED for at least 60 minutes after the last β-agonist treatment helps ensure suitability for discharge. Patients should receive written medication instructions, a written asthma action plan, and instructions for follow-up. Invariably patients should be discharged on inhaled and/or oral steroids. Patients presenting with a mild exacerbation that completely resolves after bronchodilators may be discharged on inhaled steroids or the combination of a long-acting β agonist and inhaled steroid, particularly if they were not previously on controller therapy. Patients with incomplete responses or attacks of greater severity should receive a course of oral steroids such as subcutaneously an 8-day course of 40 mg/day prednisone (37). Alternatively, a single dose of triamcinolone diacetate 40 mg intramuscularly also has been reported to be as effective as prednisone 40 mg/day for 5 days (38). When considering hospitalization, health care providers should err on the side of admission when there is a risky home environment or directly observed therapy is needed in the case of noncompliance.
Clearly patients with more severe attacks, or who demonstrate a lackluster response or a deteriorating response to initial therapy require hospital admission. Indications for intensive care unit admission include frank or impending respiratory arrest, progressive hypercapnia, mechanical ventilation, altered mental status, cardiac arrhythmias, myocardial injury, and need for frequent bronchodilator treatments (19).
Pharmacologic Therapy
Oxygen
Supplemental oxygen by nasal cannula is titrated to maintain arterial oxygen saturations greater than 92% (>95% with pregnancy and ischemic heart disease). Adequate oxygenation is generally not difficult to achieve with low-flow supplementation (see above) and is important in maintaining oxygen delivery to tissue beds and minimizing hypoxic pulmonary vasoconstriction. Supplemental oxygen further protects against hypoxemia resulting from β agonist–induced pulmonary vasodilation and increased blood flow to low V/Q units (39).
β Agonists
Inhaled short-acting β agonists such as albuterol and levalbuterol are used to treat the smooth muscle–mediated bronchoconstriction of ASA. Approximately two-thirds of patients respond convincingly to initial inhaled therapy in the ED, leaving one-third of patients with a lackluster response. This latter group invariably requires prolonged treatment in the ED or admission to hospital. In the study by Rodrigo and Rodrigo, 67% of patients improved significantly and were discharged from the ED after 2.4 mg albuterol (Fig. 21.1) (40). Half of the responders in this study met discharge criteria
after receiving only 12 puffs of albuterol. Similarly, Strauss and co-workers found that two-thirds of patients with acute asthma could be discharged after three 2.5-mg doses of albuterol by nebulization every 20 minutes (41).
after receiving only 12 puffs of albuterol. Similarly, Strauss and co-workers found that two-thirds of patients with acute asthma could be discharged after three 2.5-mg doses of albuterol by nebulization every 20 minutes (41).
The optimal dose of albuterol in ASA has yet to be established. McFadden and colleagues compared two 5.0-mg nebulized treatments of albuterol over 40 minutes with three 2.5-mg albuterol every 20 minutes in 160 ED patients (42), demonstrating a dose–response relationship between albuterol and PEFR. One 5 mg albuterol dose achieved the same effect as two doses of 2.5 mg, and 10 mg albuterol resulted in higher PEFRs than 7.5 mg. The 5-mg regimen increased peak flows more rapidly and to a greater extent than the standard 2.5-mg approach. Patients receiving 5-mg doses reached discharge criteria quicker and left the ED with higher PEFRs. There was also a trend toward fewer hospitalizations in the high-dose group (25 of 80 patients, 31%) than in the lower dose group (37 of 80 patients, 46%) (p = 0.06). However, Emerman and colleagues compared the effects of three doses of 2.5 or 7.5 mg albuterol every 20 minutes in 160 acutely ill asthmatics, finding no differences in spirometry or admission rates (43). In sum, these data support the standard recommendation of administering albuterol 2.5 mg by nebulization every 20 minutes during the first hour of treatment (i.e., 3 doses); for more severe exacerbations 10 mg to 15 mg of albuterol by continuous nebulization may be used (19).
Albuterol should be used in a continuous or repetitive manner until there is convincing improvement or side effects limit further administration (Table 21.2
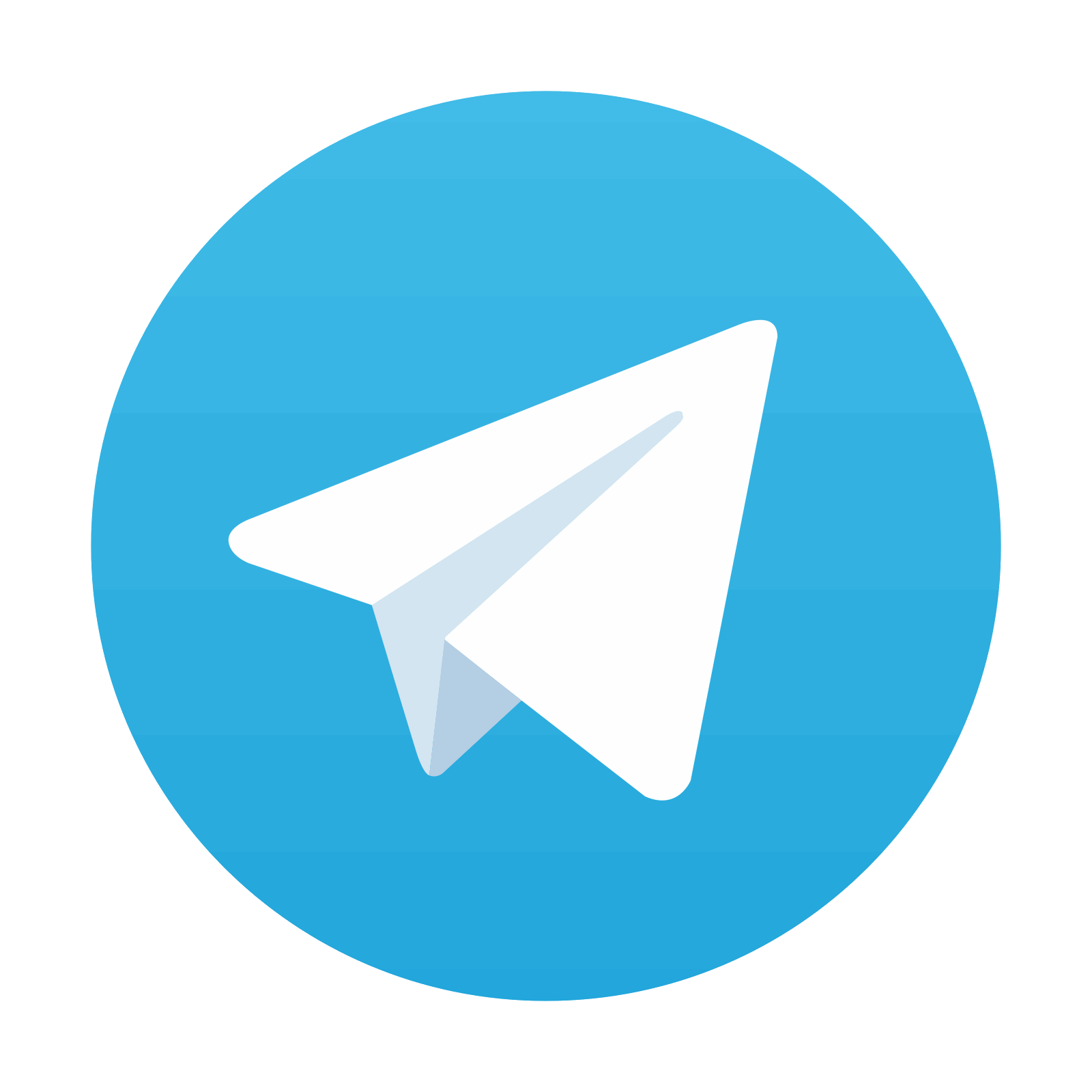
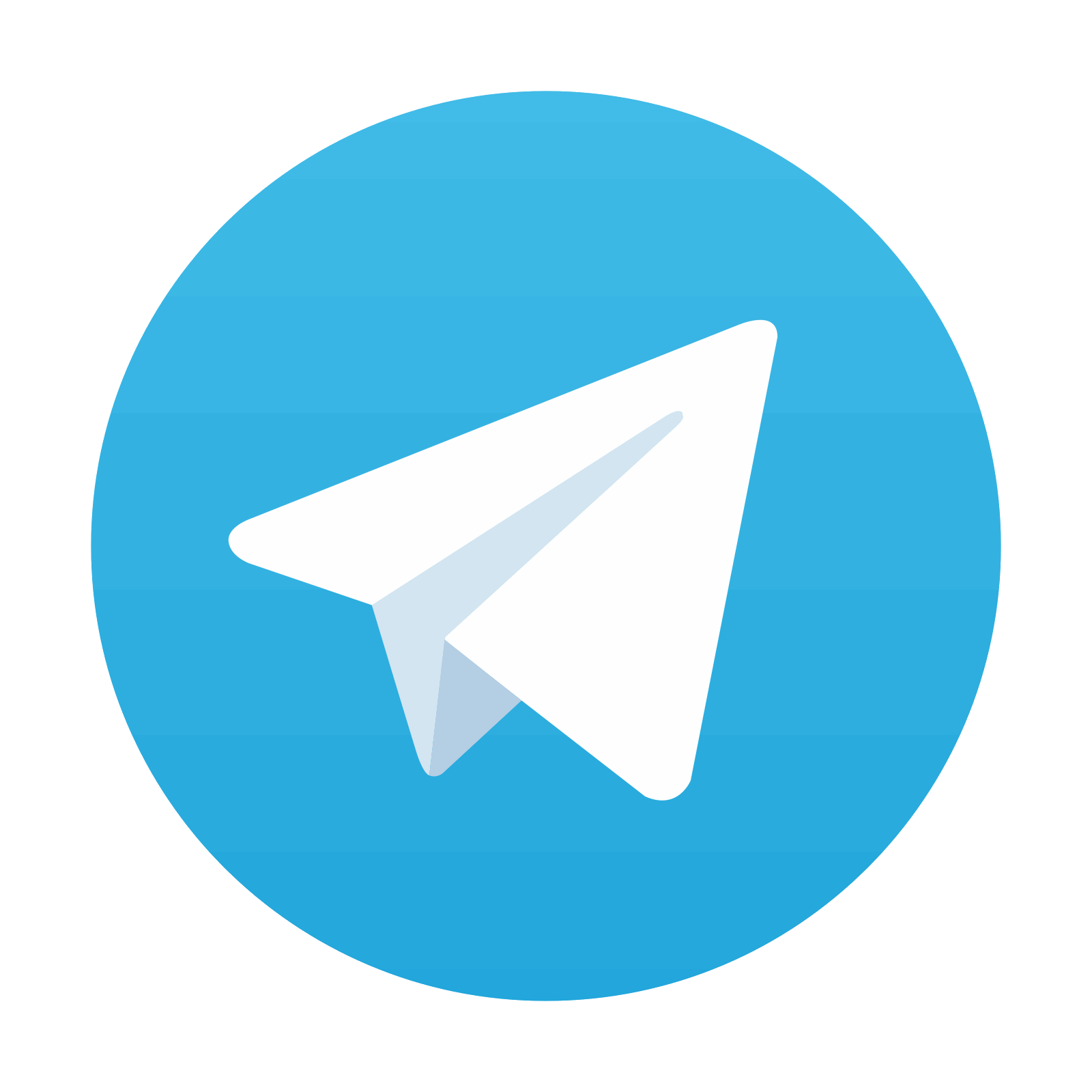
Stay updated, free articles. Join our Telegram channel

Full access? Get Clinical Tree
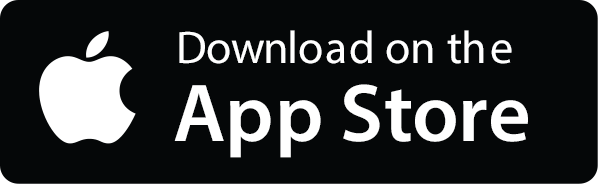
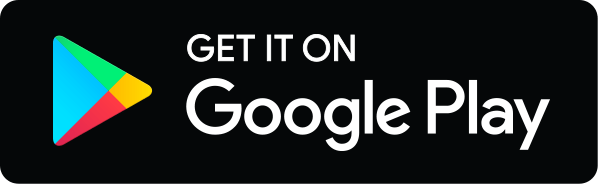
