Major Platelet Glycoproteins: Integrin αIIbβ3 (GPIIb-IIIa)
Feng Ye
Mark H. Ginsberg
The first insight into the involvement of the integrin αIIbβ3 in hemostasis came from the observation that two major glycoprotein bands were either abnormal or completely missing from the platelets of some patients with Glanzmann thrombasthenia (GT),1,2 a rare inherited bleeding disorder characterized by the absence of platelet aggregation. The two glycoproteins, named glycoproteins IIb (GPIIb) and IIIa (GPIIIa), were later found to form a cation-dependent complex.3,4 The Phillips lab then successfully purified and reconstituted a functional complex containing these two glycoproteins, demonstrating biochemically that GPIIb and IIIa are subunits of the same protein.3,5
In the mid-1980s, a family of functionally and structurally related adhesion receptors, including GPIIb/IIIa,6,7,8 emerged from research in a variety of biologic disciplines including immune biology and vascular biology and development. This family of proteins functionally integrates their extracellular ligands with the intracellular cytoskeleton and was formally named integrin in 1987. GPIIb/IIIa was renamed as integrin αIIbβ3.9
Further research demonstrated that the interaction between αIIbβ3 and its ligands was a critical event for platelet aggregation, hemostasis, and thrombosis.10,11,12 Biochemical and structural details of the interaction between αIIbβ3 and its physiologic ligands facilitated the development of αIIbβ3 antagonists that are currently used in the treatment of acute arterial thrombosis by percutaneous coronary intervention (PCI).13,14,15,16 Work over the past two decades underscored that integrins, including αIIbβ3, are not merely adhesion molecules but also signaling receptors.17,18,19 Signals originating from inside the cell can modulate ligand binding to integrin extracellular domains (inside-out signaling). Inside-out signaling is tightly controlled, both temporally and spatially, to achieve hemostasis and prevent unwanted vessel occlusion. At the same time, signals initiated by ligand binding to the integrin extracellular domains (outside-in signaling) can trigger intracellular signals to regulate anchoragedependent cellular responses. In the case of platelets, these include full aggregation and granule secretion as well as clot retraction. In this chapter, we will discuss integrin αIIbβ3 and its role in platelet function and bleeding disorders.
EXPRESSION AND STRUCTURE OF INTEGRIN αIIbβ3
αIIbβ3 expression is limited primarily to cells of the megakaryocytic lineage. However, the β3 subunit is widely expressed as the beta subunit of the integrin αvβ3. Therefore, restriction of αIIbβ3 expression is limited by the promoter activity of αIIb in cell types other than megakaryocytes.20,21 Only a few exceptions have been reported. For example, αIIbβ3 is detected in certain cancer cells.22 αIIbβ3 has also been detected on early hematopoietic progenitors and on mast cells where it plays a role in adhesion and in the response of the latter cells to chronic inflammation.23,24 Efficient surface expression of the αIIbβ3 complex requires αIIb and β3 in a ratio of 1:1.25,26,27 Excess expression of one results in the accumulation of the excess in the endoplasmic reticulum where association of nascent αIIb and β3 subunits occurs. Indeed, a subset of patients with GT results from mutations that alter the biosynthesis of only one subunit. In turn, this impairs the surface expression of the complete αIIbβ3 complex.28,29
αIIbβ3 is the most abundant protein on the platelet surface: there are approximately 80,000 copies on the surface of unactivated platelets.30 An intracellular pool of αIIbβ3, located in the membrane of α-granules, can be translocated to the cell surface following platelet activation, increasing the amount of αIIbβ3 by 30% to 50%.30,31,32 This high level of expression is important for efficient platelet function as platelets need to strongly adhere to multiple adhesive proteins to form a stable clot.
β3 is expressed as a single polypeptide of 762 amino acid that folds into 10 distinct domains: a plexin-semaphorin-integrin (PSI) domain at the N-terminal, a βA domain that is inserted into the hybrid domain, followed by hybrid, EGF-1, EGF-2, EGF-3, EGF4, and βTD domains, a transmembrane domain (TMD) embedded in the lipid bilayer and a cytoplasmic domain33,34 (FIGURE 26B.1). Folding is facilitated by 28 disulfide bonds formed from 56 cysteine residues. The β3 βA domain has three divalent cation-binding sites: a metal ion-dependent adhesion site (MIDAS), a site adjacent to MIDAS, and a ligandinduced metal binding site, also referred to as the synergistic metal ion binding site. The β3 A-domain assumes a nucleotide binding (or Rossmann) fold in which a central six stranded β-sheet is surrounded by eight α-helices33,34 (FIGURES 26B.1, 26B.2 and 26B.3).
αIIb has 1,008 amino acid and folds into six domains: β-propeller, thigh, calf-1, calf-2, transmembrane, and cytoplasmic domains.33,34 αIIb is initially synthesized as a single polypeptide chain. During protein folding and maturation, it is proteolytically cleaved at a site in the calf-2 domain into heavy and light chains by a furin-like enzyme in the Golgi complex. However, the heavy and light chains remain covalently associated via a disulfide bond.35 Thus, in nonreduced sodium dodecyl sulfate gels, αIIb has an apparent molecular weight of 130 kDa. In reducing gels, however, it consists of a 115 kDa fragment containing most of its extracellular domains and a 25 kDa fragment that contains part of the calf-2 domain, as well as the transmembrane and the cytoplasmic domains. At its N-terminus, αIIb consists of seven β sheets containing approximately 60 amino acids each that fold into a seven-bladed β-propeller (FIGURE 26B.2A). Four divalent cation binding sites are found at the base of the
propeller.33,34 A flexible loop between the thigh and the calf-1 domain (often referred to as the “genu” region) then allows the molecule to undergo a functionally important global structural rearrangement33,34,36,37 (FIGURE 26B.1).
propeller.33,34 A flexible loop between the thigh and the calf-1 domain (often referred to as the “genu” region) then allows the molecule to undergo a functionally important global structural rearrangement33,34,36,37 (FIGURE 26B.1).
αIIb and β3 associate to form a noncovalent heterodimer. Several interfaces hold the heterodimer together: a large interface between the β-propeller and βA domain that buries approximately 1,600 Å2 of surface area and with Arg261 of the βA domain protruding off-center into the β-propeller’s channel33,34,38 (FIGURE 26B.2B); an interaction between the αIIb and β3 TMDs; and an interaction between the membrane proximal (MP) portions of each cytoplasmic domain.39,40 Divalent cations are involved in this association because exposure to ethylenediaminetetraacetic acid causes the heterodimer to dissociate.4,41 Although no divalent cation is observed at the α and β interface, cations in the β-propeller and thigh-calf-1 interface might allosterically contribute to α and β interactions.33 The Arg-Gly-Asp or RGD motif present in many integrin ligands inserts into a crevice between the βA and β propeller domains. The Arg side chain inserts into a narrow groove on the top of the β-propeller, and the side chain of Asp protrudes into a cleft in the βA domain. The Gly residue lies at the interface between the α and β subunits where it makes several hydrophobic contacts. Overall, 355 Å2 of surface area or 45% of the total surface area of the peptide is buried, thereby contributing to a stable ligand-receptor interaction42 (FIGURE 26B.3).
Structures of the αIIbβ3 TMD heterodimer, determined by nuclear magnetic resonance (NMR) spectroscopy, revealed that
the αIIb and β3 TMD pack together at two main interfaces with a vertical αIIb TMD and tilted β3 TMD.39 At the outer membrane region, αIIb and β3 tightly pack against each other via a groove formed in the αIIb G972XXXG976 motif and β3 G708 (termed outer membrane clasp or OMC). The β3 TMD forms a continuous helix that extends to the MP region of the cytoplasmic domain. A hydrophobic patch in the membrane-proximal region of β3 is inserted into the membrane as a part of the TMD. Consequently, the β3 TMD is long and crosses the lipid bilayer at an angle of 25 degrees. In contrast, the αIIb TMD is a short and straight helix that ends at Gly991. Distal to Gly991, αIIb makes a sharp turn toward β3, enabling Phe992 and Phe993 to embed in the membrane and pack against β3 residues Trp715 and Ile719. In addition, this places αIIb Arg995 in close apposition to Asp723 of β3, enabling an electrostatic interaction between them. The Phe packing interfaces and Arg995-Asp723 interaction completes the inner membrane interface (termed inner membrane clasp, or IMC)39 (FIGURE 26B.4).
the αIIb and β3 TMD pack together at two main interfaces with a vertical αIIb TMD and tilted β3 TMD.39 At the outer membrane region, αIIb and β3 tightly pack against each other via a groove formed in the αIIb G972XXXG976 motif and β3 G708 (termed outer membrane clasp or OMC). The β3 TMD forms a continuous helix that extends to the MP region of the cytoplasmic domain. A hydrophobic patch in the membrane-proximal region of β3 is inserted into the membrane as a part of the TMD. Consequently, the β3 TMD is long and crosses the lipid bilayer at an angle of 25 degrees. In contrast, the αIIb TMD is a short and straight helix that ends at Gly991. Distal to Gly991, αIIb makes a sharp turn toward β3, enabling Phe992 and Phe993 to embed in the membrane and pack against β3 residues Trp715 and Ile719. In addition, this places αIIb Arg995 in close apposition to Asp723 of β3, enabling an electrostatic interaction between them. The Phe packing interfaces and Arg995-Asp723 interaction completes the inner membrane interface (termed inner membrane clasp, or IMC)39 (FIGURE 26B.4).
STRUCTURAL MECHANISMS OF INTEGRIN REGULATION
The combination of crystal and NMR structures and mutational studies has provided a detailed picture of integrin regulation. Integrin TMDs have two main interaction interfaces with three main contacts.39,40 Mutations that disrupt these interactions favor the active form of the integrin. Charge reversal mutations of either αIIb Arg995 or β3 Asp723 disrupt the IMC and favor integrin activation, whereas combined charge reversals shift the equilibrium toward the inactive form.43 Similarly, Phe to Ala mutations in the αIIb MP region disrupt the IMC, inducing constitutive αIIbβ3 activity.43
Activating mutations are also found in the OMC. Most are substitutions of the Gly residues in both the αIIb G972XXXG976 motif and β3 Gly708 with residues containing bulky side chain, such as Leu, Ile, or Asn.44,45,46 The side chains of these residues disrupt the tight packing interface between αIIb G972XXXG976 motif and the β3 TMD.39,47 Furthermore, the 25-degree tilting angle of the β3 helix is required to enable simultaneous interactions at the IMC and OMC.39 Mutations that change the β3 tilting angle, such as Lys716 to Ala, also result in disruption of the association of the β3 TMD with αIIb.17 In short, integrins are kept in an inactive form by TM and cytoplasmic domain interactions. Following inside-out signaling, the αIIb and β3 TMD rearrange, causing integrin activation.
Integrin extracellular domains have multiple conformations, including those associated with inactive, active, and active ligand-occupied forms. The transition from one conformational state to another results in characteristic exposure of epitopes. A large inventory of conformation-specific αIIbβ3 antibodies has accumulated to study the relationship between αIIbβ3 conformation and function. How do the TMD and cytoplasmic domain rearrangements propagate to the integrin head
piece to cause higher ligand binding affinities? One hypothesis proposes that a bent integrin conformation has a low affinity for ligand, while a more open and extended conformation is associated with high ligand affinity37 (FIGURE 26B.5). It has also been proposed that TMD and cytoplasmic domain separation results in extension of integrin extracellular domain at the genu region and swing out of the β3 hybrid domain.48 The β3 hybrid domain movement is coupled through the βA α7-helix to rearrange the ligand binding crevice at the interface between β-propeller and βA domains.38 This global structural rearrangement increases the affinity of αIIbβ3 for its ligand37 (FIGURE 26B.5). An alternative hypothesis posits that the bent conformation can be an active conformer, and that extension of the molecule is a post-ligandbinding event. This theory also has experimental support.33,42,49,50 This is an area where there is likely to be continued debate.
piece to cause higher ligand binding affinities? One hypothesis proposes that a bent integrin conformation has a low affinity for ligand, while a more open and extended conformation is associated with high ligand affinity37 (FIGURE 26B.5). It has also been proposed that TMD and cytoplasmic domain separation results in extension of integrin extracellular domain at the genu region and swing out of the β3 hybrid domain.48 The β3 hybrid domain movement is coupled through the βA α7-helix to rearrange the ligand binding crevice at the interface between β-propeller and βA domains.38 This global structural rearrangement increases the affinity of αIIbβ3 for its ligand37 (FIGURE 26B.5). An alternative hypothesis posits that the bent conformation can be an active conformer, and that extension of the molecule is a post-ligandbinding event. This theory also has experimental support.33,42,49,50 This is an area where there is likely to be continued debate.
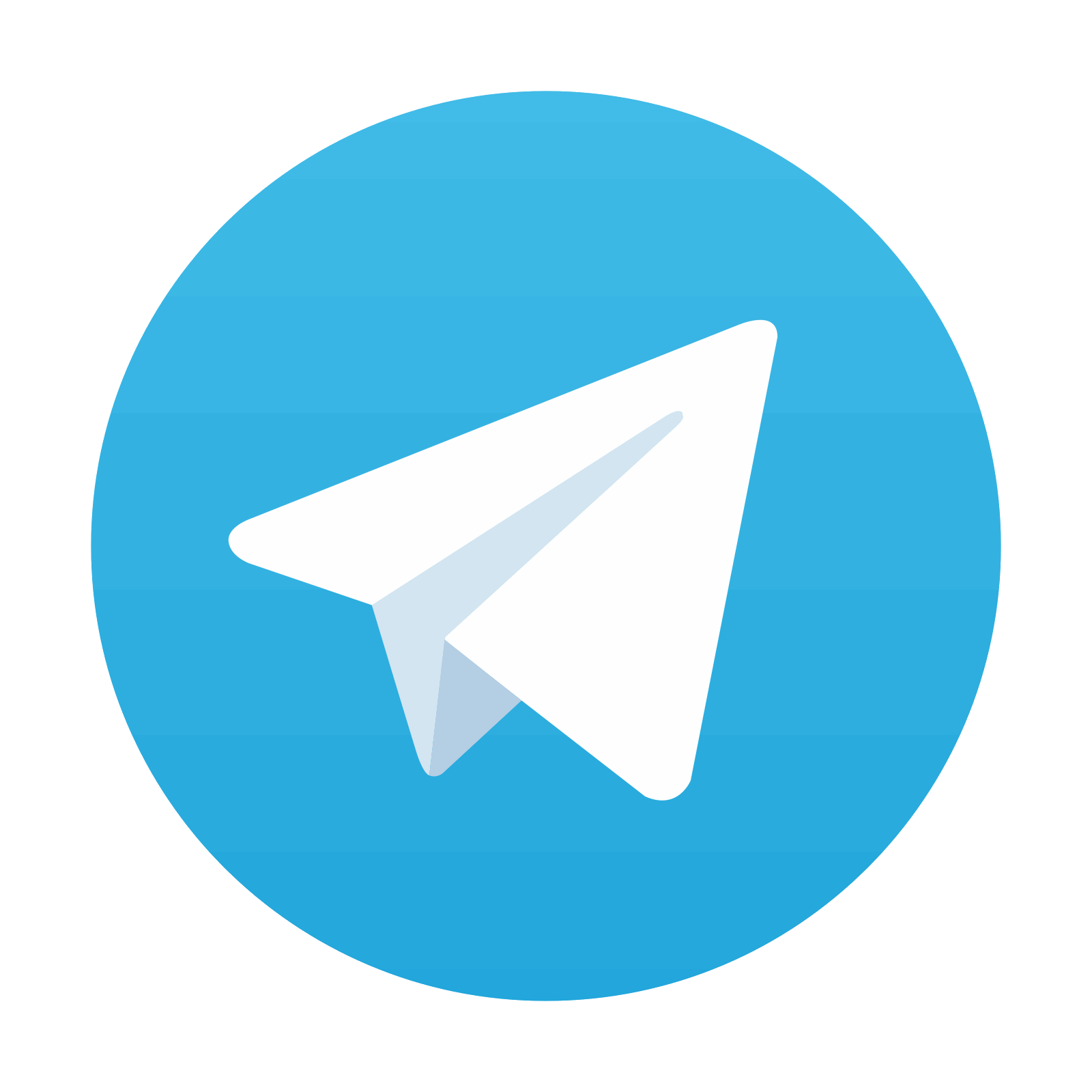
Stay updated, free articles. Join our Telegram channel

Full access? Get Clinical Tree
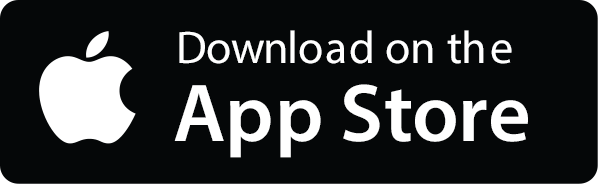
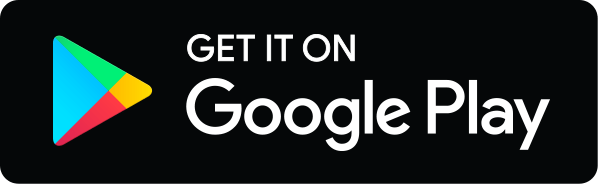