Long-Term and Late Effects of Primary Curative Intent Therapy: Neurocognitive, Cardiac, and Secondary Malignancies
Rena D. Callahan
Patricia A. Ganz
The number of women living in the United States with a history of breast cancer has been rising and is estimated to be over 2.8 million (1). Every year, new treatments and refinements of existing treatments are incorporated into the armamentarium against breast cancer. Advances in therapy have led to decreases in breast cancer mortality, and are partly responsible for the large number of survivors. While efficacious, many treatments are associated with persistent difficulties that are especially impactful for those who are long-term disease-free survivors (2, 3). Among the most feared and disabling are cognitive and cardiac dysfunction. Rarely, breast cancer treatment results in a new, second cancer. The ability to better characterize these treatment-related consequences, specifically the incidence, etiology, pathogenesis, and risk factors for development, will facilitate the design of effective preventive strategies and/or therapeutic interventions in the future. To this end, we review what is known about these long-term and late effects of breast cancer treatments, and where appropriate, discuss strategies for prevention and treatment.
NEUROCOGNITIVE EFFECTS OF BREAST CANCER TREATMENT
Etiology and Setting of Cognitive Dysfunction
Cognitive complaints are extremely common during adjuvant chemotherapy, and may be caused by anxiety, depression, supportive care medications (e.g., anxiolytics, steroids, and sedatives), or by the acute effects of cancer therapy such as fatigue. However, for the majority of women, these acute changes resolve in the months following the end of treatment (4). What is more concerning is the persistence of difficulties in cognitive functioning that affect work, self-management, and caring for others that have been identified for 20 years, as something called chemobrain (5). Chemobrain may be a misnomer, since the manifestations that include memory impairment and difficulty with attention and concentration are also seen in breast cancer patients who have not received chemotherapy. A more inclusive term to describe these manifestations is cancer- or cancer-therapy-associated cognitive change (6).
Across clinical studies of treatment associated cognitive dysfunction, the incidence varies greatly, ranging between 15% to 61% (7, 8) and can be seen in association with chemotherapy and with endocrine therapy alone. Additionally, subtle cognitive impairment is present in many patients prior to treatment (9, 10 and 11) and could reflect postsurgical systemic changes or psychological effects on the brain associated with the cancer diagnosis. In one study, 22% of patients with stages I to III invasive breast cancer scored “lower than expected” on cognitive testing prior to chemotherapy compared with the absence of such findings in patients with stage 0 breast cancer (12).
Across clinical studies of treatment associated cognitive dysfunction, the incidence varies greatly, ranging between 15% to 61% (7, 8) and can be seen in association with chemotherapy and with endocrine therapy alone. Additionally, subtle cognitive impairment is present in many patients prior to treatment (9, 10 and 11) and could reflect postsurgical systemic changes or psychological effects on the brain associated with the cancer diagnosis. In one study, 22% of patients with stages I to III invasive breast cancer scored “lower than expected” on cognitive testing prior to chemotherapy compared with the absence of such findings in patients with stage 0 breast cancer (12).
It has been difficult to accurately assess the scope of the problem due to heterogeneity in study design, including patient population and measurement instruments (13). Early studies were cross-sectional in design, and although comparison groups were available, it was difficult to assess causal attribution (14, 15 and 16). Subsequent studies were designed to clarify the epidemiology of chemobrain, with assessments of neurocognitive function before and after treatment, at several timepoints, and using a concurrent control or comparison group. A recent meta-analysis of multiple posttreatment studies of breast cancer patients concluded that there were small, but significant differences in verbal abilities and visuospatial functioning in these survivors who had been treated with standard dose adjuvant chemotherapy (17).
The mechanism by which cancer treatment leads to cognitive dysfunction is not well understood. Additionally, there may be interplay of various mechanisms that collectively impair cognitive functioning. There are excellent reviews of this topic that the reader should consider that are beyond the scope of this chapter (18, 19 and 20). However, in the text that follows, we highlight some of the findings from various studies that relate to cancer-associated cognitive dysfunction.
Risk Factors for Cognitive Dysfunction
There are a number of potential risk factors that interact with each other or work independently influencing cerebral function in the setting of early breast cancer treatment. Ganz and colleagues (21) have recently proposed a breast cancer specific model as part of their Mind Body Study (see Fig. 52-1). Below, we discuss how these factors may be influencing cognitive dysfunction in posttreatment breast cancer survivors.
Psychosocial Factors:
Both the diagnosis and treatment of breast cancer result in psychological distress. The association of depression and deterioration in cognitive performance has been well described, and when severe is termed, pseudodementia (22). For the breast cancer patient, emotional factors can contribute to, but alone cannot explain the treatment-associated cognitive decline. In a prospective study of 41 women with breast cancer and without depression at baseline, who were followed prior to and during chemotherapy treatment, the objective cognitive decline seen was independent of emotional factors (7). However, the development of depression and anxiety did correlate with a self-reported decline in cognitive functioning, even when objective performance remained stable (7). In a report of the Cognitive Impairment in Therapy of Breast Cancer (COGITO) study (23), a validated battery of 12 cognitive tests, self-reports on cognition and emotional state, were assessed prior to and up to 1 year postchemotherapy treatment. In this study, depression and the personality trait negative affectivity predicted for self-reported cognitive impairment. However, there was no statistically significant association between self-reports and objective measures of cognitive changes (23). While asynchrony between self-report and formal neurocognitive testing has been observed in some studies, a recent report by Deprez et al. identified concordance between self-report, neurocognitive testing, and white matter tract changes in a prospective, observational study of women initiating chemotherapy (24). Similarly, in a long-term cross-sectional study comparing controls and breast cancer survivors who had been treated with chemotherapy (25), executive function self-report difficulties corresponded to decreased frontal activation on MRI as well as poorer performance on neurocognitive tests. These imaging studies, with examination of domain-specific cognitive complaints and relevant NP tests, suggest that it is possible to identify neurophysiological correlates of these subjective complaints (25, 26).
Genetic Susceptibility:
There has been considerable interest in establishing potential genetic vulnerability to the development of cognitive dysfunction after breast cancer treatments. Initial interest has focused on risk factors common for dementia in older adults. In a cross-sectional evaluation of long-term survivors of breast cancer and
lymphoma, Ahles et al. (27) found an association between poorer neuropsychological performance and the presence of the apolipoprotein (APOE) E4 allele. This was significant for visual memory and spatial domains, with a trend toward lower scores in the psychomotor domain, when E4 carriers were compared to noncarriers among the survivors.
lymphoma, Ahles et al. (27) found an association between poorer neuropsychological performance and the presence of the apolipoprotein (APOE) E4 allele. This was significant for visual memory and spatial domains, with a trend toward lower scores in the psychomotor domain, when E4 carriers were compared to noncarriers among the survivors.
In another study of breast cancer survivors, Small et al. (28) examined single-nucleotide polymorphisms (SNPs) in the catechol-o-methyl transferase (COMT) gene, which is involved in the metabolism of catecholamines through the methylation of dopamine, an important neurotransmitter. The valine version (val allele) of the gene is more active than the methionine (met allele), and individuals who are homozygous for the val allele are thought to metabolize dopamine more rapidly and thus have lower levels available than those with the met allele, potentially modulating the dopaminergic tone in the frontal cortex. In their study (28), breast cancer patients who had the COMT-val allele who received chemotherapy were found to have poorer neuropsychological performance on attention, verbal fluency, and motor speed in comparison to breast cancer patients who were COMT-met homozygotes.
In our work examining SNPs in the promoter region of several proinflammatory genes, we have found an association between cognitive complaints and the TNFα 308 G > A polymorphism (p = .055), and for a genetic risk index among polymorphisms in the IL-1β -511 C>T (rs 16944), IL6 -174 G>C (rs1800795), and TNF -308 G>A (rs1800629), p = .016) (28a). An additive genetic risk score was computed by summing the number of high expression alleles (0/1/2) across all three polymorphisms, reflecting the potential for interaction among multiple SNPs in leading the risk for a persistent elevation in proinflammatory cytokines as a result of cancer treatment. While this has been most extensively studied in the setting of post-treatment fatigue (29), uncontrolled systemic inflammation may also be affecting neurocognitive processes (see below).
Inflammatory Processes:
A potential etiologic factor in cognitive decline is systemic inflammation. Proinflammatory cytokines have been found to be associated with cancer symptoms as well as age-related cognitive decline (30, 31). Tumor necrosis factor alpha (TNFα), a cytokine involved in the inflammatory response, was elevated in a study of patient with Alzheimer’s dementia (32). In addition to its role in inflammation, TNFα may play a regulatory role in mediating synaptic function in the brain (33). Thus, elevated circulating levels of TNFα from systemic inflammatory processes are implicated in the pathogenesis of Alzheimer’s dementia and possibly other forms of cognitive dysfunction. In mice treated with doxorubicin, increased levels of TNFα in serum and brain tissue have been reported (34). Analysis of the first 93 patients of a prospective, longitudinal study of breast cancer patients who had been treated with surgery, radiation, and chemotherapy revealed increases in TNFα among those who reported cognitive dysfunction (21). In this study, patients were enrolled after chemotherapy, surgery, and radiation and monitored with neuropsychological tests and bloodwork including markers of inflammation. Of the inflammatory cytokines tested (IL-1ra, sTNF-RII, CRP, and IL-6), only sTNF-RII was elevated at baseline in those with memory complaints. Additionally, there was a statistically significant correlation between decrease in memory complaints and decline in levels of sTNF-RII (21). Another recent study in long-term breast cancer survivors (35) found an association between increased IL-6 and TNFα and decreased hippocampal volume, increased cognitive complaints, and poorer verbal memory test performance compared to a healthy control group. These emerging data suggest a potential inflammatory etiology for cognitive dysfunction in breast cancer patients and survivors.
Hormonal Effects and the Brain:
Endocrine therapy has been implicated in therapy-associated cognitive decline among breast cancer patients. Additionally, endocrine effects of chemotherapy may play a role in cognitive impairment after treatment. In a prospective, longitudinal study of women with early-stage breast cancer, the ability to perform various neuropsychological “tasks” was assessed before and after treatment (36). Women who experienced chemotherapy-induced amenorrhea were more likely to show a decline from baseline in multiple tasks (36). Endogenous estrogen appears to play an important role in cognitive function, especially with respect to verbal memory. It has been shown that estrogen receptors are concentrated in the hippocampus, which is involved in cholinergic neurotransmission to areas of the brain involved with memory and learning. A second potential mechanism by which estrogen is neuroprotective lies in its ability to relieve oxidative stress by scavenging oxygen free radicals and reducing the formation of reactive oxygen species (37). A third mechanism by which estrogen affects cognition is via effects on telomeres. Telomeres are DNA protein structures that form caps on the ends of chromosomes, which results in genomic stability. During the process of aging, telomere length shortens. This increase in genomic instability may contribute to various age-related phenomena, including decline in cognitive ability (38). One study of 53 postmenopausal women on 1 year of hormone replacement therapy found that duration of endogenous estrogen exposure (length of reproductive years) was correlated with longer telomere length and decreased telomerase activity. Interestingly, duration of exogenous estrogen exposure did not show the same effects (39). However, in another study of 393 community-dwelling women aged 55 to 89 years who were not using replacement estrogen, there was no relationship of endogenous estrogen levels to higher performance tests of cognitive function, including the mini mental status examination (MMSE) (40).
The role of medications that alter estrogen levels in the body in cognition is unclear. The Women’s Health Initiative Memory Study (WHIMS) assessed annual MMSE scores in 4,532 postmenopausal women enrolled in the larger WHI study who were over age 65 years and free of probable dementia at baseline (41, 42). After a mean follow-up of 4.2 years, no significant improvement in global cognitive function was seen with combined estrogen-progestin therapy compared with placebo (41). In addition, more women in the combined hormone therapy group had clinically significant declines in MMSE scores compared with placebo (6.7% vs. 4.8%, respectively). A similar decline in cognitive ability was shown in women taking estrogen without progestin (43). This analysis was not able to assess the relationship of timing of estrogen replacement to cognitive decline, which is important in interpreting the significance of these data. Estrogen exposure may have differential effects depending on timing in relation to menopause. The critical window hypothesis posits that estrogen serves a neuroprotective role in perimenopause, but has neutral, and even detrimental effects later in the menopausal transition (44). One mechanistic explanation is that “healthy cells,” such as those neurons in the younger female brain, derive benefit from estrogenic effects, whereas the age-damaged neurons of older women do not (45).
Evidence for Brain Abnormalities after Cancer Treatment
Neuroimaging has been utilized to define structural and functional correlates of cognitive function. Treatment-induced memory loss may be attributable to damage to the frontal lobes involved in executive function and the hippocampus, important for memory. Alterations in cerebral blood flow during neuropsychological testing have been observed in patients 5 to 10 years after adjuvant chemotherapy treatment (46). In this cross-sectional comparison study, the alteration was most marked in the inferior frontal gyrus, an area of the brain involved in memory processing, and was most marked in patients who had received both chemotherapy and tamoxifen. In a recent prospective, pretreatment study using MRI in patients with breast cancer treated with chemotherapy compared to breast cancer patients without chemotherapy and healthy controls, McDonald and colleagues (47) observed decreased gray matter density in the bilateral frontal, temporal, and cerebellar regions and right thalamus in both cancer groups that was most prominent after chemotherapy. These changes partially recovered, at 1 year posttreatment. Another prospective study demonstrated changes in white matter detected by magnetic resonance diffusion tensor imaging after chemotherapy treatment (24). White matter abnormalities were not seen at baseline in any group or in patients with breast cancer who did not receive chemotherapy. And in a more recent prospective pretreatment longitudinal study using functional MRI, with similar comparison groups, the investigators observed baseline frontal lobe hyperactivation during working memory tasks in cancer patients versus healthy controls (48). The cancer patients also exhibited a decline in frontal activation 1 month after completion of chemotherapy or at a similar interval for those patients who did not receive chemotherapy. One report of identical twins, one with breast cancer who received chemotherapy and the other without breast cancer, revealed marked differences in structural and functional MRI (49). The twin with breast cancer exhibited greater white matter volume, which is also seen in patients with Alzheimer’s dementia. On functional MRI, she showed a greater spatial extent of brain activation than her untreated twin, a feature seen as a compensatory mechanism in patients with underlying brain dysfunction (49, 50 and 51). Taken together, all of these studies provide increasing support for both structural and functional brain changes that are associated with chemotherapy treatment, along with evidence of both subjective and objective measures of impaired cognitive function in the patients who have been studied (26).
Strategies for Prevention and Treatment
Because the specific causal factors for cancer-related cognitive dysfunction are not well defined, preventive strategies have been difficult to develop. In addition, breast cancer adjuvant therapy has been shown to provide substantial survival benefit, and thus avoidance of therapy to prevent this side effect does not seem warranted at this time. Further, identification of at-risk populations has been difficult, although the earliest studies of chemotherapy-related cognitive problems were associated with the historic cytoxan, methotrexate, and 5-fluorouracil (CMF) regimen, which appears to have had long-lasting effects on cognitive function and brain structure (52, 53 and 54). This regimen might be avoided given these problems, while uncertainty remains about the specificity of harms from regimens that are currently in common use in the adjuvant setting.
Thus, the few treatment approaches that have been developed focus on management of symptoms. Cognitive behavioral therapy has shown promise in smaller trials and is now being explored in larger-scale trials. In a pilot study of a brief cognitive behavioral intervention, improvements in cognitive function, quality of life, and standard neuropsychological test performance were observed (55). This intervention was further developed and evaluated in 40 patients with early-stage breast cancer. The study was randomized with a waitlist-control group. Postintervention, there were significant improvements in verbal memory and quality of life but not for cognitive complaints (56). A recent study examined a group-based cognitive intervention in 23 cancer survivors, compared to 9 waitlist-control cancer survivors and to 23 adults without a history of cancer. In comparison to the control groups, the intervention group demonstrated improvements in cognitive functioning, memory, and visuospatial function that were maintained over 3 months (57). Finally, a randomized waitlist-control trial, which included a computerized task, evaluated two different cognitive training interventions: speed of processing and memory training (58). Both interventions improved objective and perceived cognitive function, symptom distress, and quality of life. Interestingly, the speed of processing training resulted in transferred benefits on memory tests.
It is unclear whether medications have a role in the treatment of cognitive dysfunction. A randomized study was conducted to evaluate modafinil for the treatment of fatigue in patients posttreatment for breast cancer. Secondary analysis of the study to assess for modafinil effect on cognitive function was performed. Improvements in memory and attention skills were seen in the group treated with modafinil. These findings must be viewed with caution given that this study was designed to evaluate treatment of fatigue (59). While the aforementioned cognitive interventions hold promise, larger trials inclusive of a wider cancer population are necessary to establish effective treatment and prevention protocols for therapy-associated cognitive dysfunction.
Summary
As with many late effects of cancer treatment, it is sometimes difficult to assess how much of what is seen in long-term survivors is a direct effect of the cancer treatment or is a manifestation of normal age-related changes. This is certainly the case for cognitive dysfunction, which is common in the aging population. However, when one observes a midlife woman who was previously engaged in high-level intellectual activities who can no longer perform those tasks in the same way, and has great difficulty managing everyday activities, one must acknowledge the likely connection between treatment exposures and the outcomes. The perplexing problem for the clinician is the fact that this problem is apparent only in a handful of patients who receive the very same treatment regimens. Elucidating the mechanisms by which some women are susceptible to this complication of cancer treatment and others are not is now our main challenge. As with all aspects of cancer treatment, the patient or host meets the disease with a variety of personal risk factors and protective factors that will interact with the cancer treatment. Factors such as cognitive reserve may be critical in determining who will experience this problem. Research advances of the past decade and studies currently underway should provide greater precision in identifying vulnerability as well as developing preventive interventions. In the meantime, we should avoid exposing patients to treatments that are not evidence based or medically indicated, given the potential risk for this long-term effect of treatment.
CARDIAC EFFECTS OF BREAST CANCER TREATMENT
The cardiotoxicity of breast cancer therapy has a major impact given the large number of women treated with adjuvant therapy who have excellent long-term survival. This includes older women who may have preexisting subclinical cardiac disease at the time of treatment, as well as younger women who can expect long-term survival and could have accelerated or premature cardiac disease as they age.
Cardiotoxicity Associated with Specific Agents Used to Treat Breast Cancer
Anthracycline-Associated Cardiotoxicity
Mechanism: Cardiotoxicity is a known side effect of anthracycline chemotherapy, which is widely used in the treatment of breast cancer. The mechanisms of cardiac damage are multifactorial and include oxygen free radical production that preferentially damages cardiac myocyte mitochondria. In addition, anthracyclines intercalate into DNA, which affects intracellular signaling and may cause cell death and the disruption of sarcomere maintenance (60). Exposure to anthracyclines may also reduce the numbers of cardiac progenitor cells available to help repair damaged myocardium (61).
Risk Factors: It is well known that anthracycline-induced cardiotoxicity is partially related to dose. The toxicity is cumulative and more common when doses approach 450 mg/m2 of doxorubicin. Von Hoff et al. reported the incidence of congestive heart failure (CHF) was 7% and 18% at doses of 550 mg/m2 and 700 mg/m2, respectively (62). However, a more recent analysis of patients enrolled in three studies of doxorubicin containing chemotherapy for breast or small cell lung carcinoma suggests that the incidence of CHF is actually higher than previously thought (63). This study estimated that doxorubicin-induced cardiotoxicity occurred in 5% of patients at a cumulative dose of 400 mg/m2, 26% of patients at 550 mg/m2, and 48% of patients at 700 mg/m2 (63). Epirubicin is also commonly used in the treatment of breast cancer. In a retrospective analysis of over 1,000 anthracycline-naïve, metastatic breast cancer patients treated with epirubicin, the maximum dose of epirubicin associated with a 5% risk of congestive heart failure was calculated. Based on the patients’ cardiac risk factors, including age, the maximum cumulative dose ranged from 300 mg/m2 to 900 mg/m2, underscoring the importance of taking individual patient characteristics into account when using cardiotoxic chemotherapy (64). In addition to cumulative dose, other risk factors for the development of anthracycline-induced cardiomyopathy include age, cardiovascular risk factors, prior radiation dose, and coexisting drug therapy.
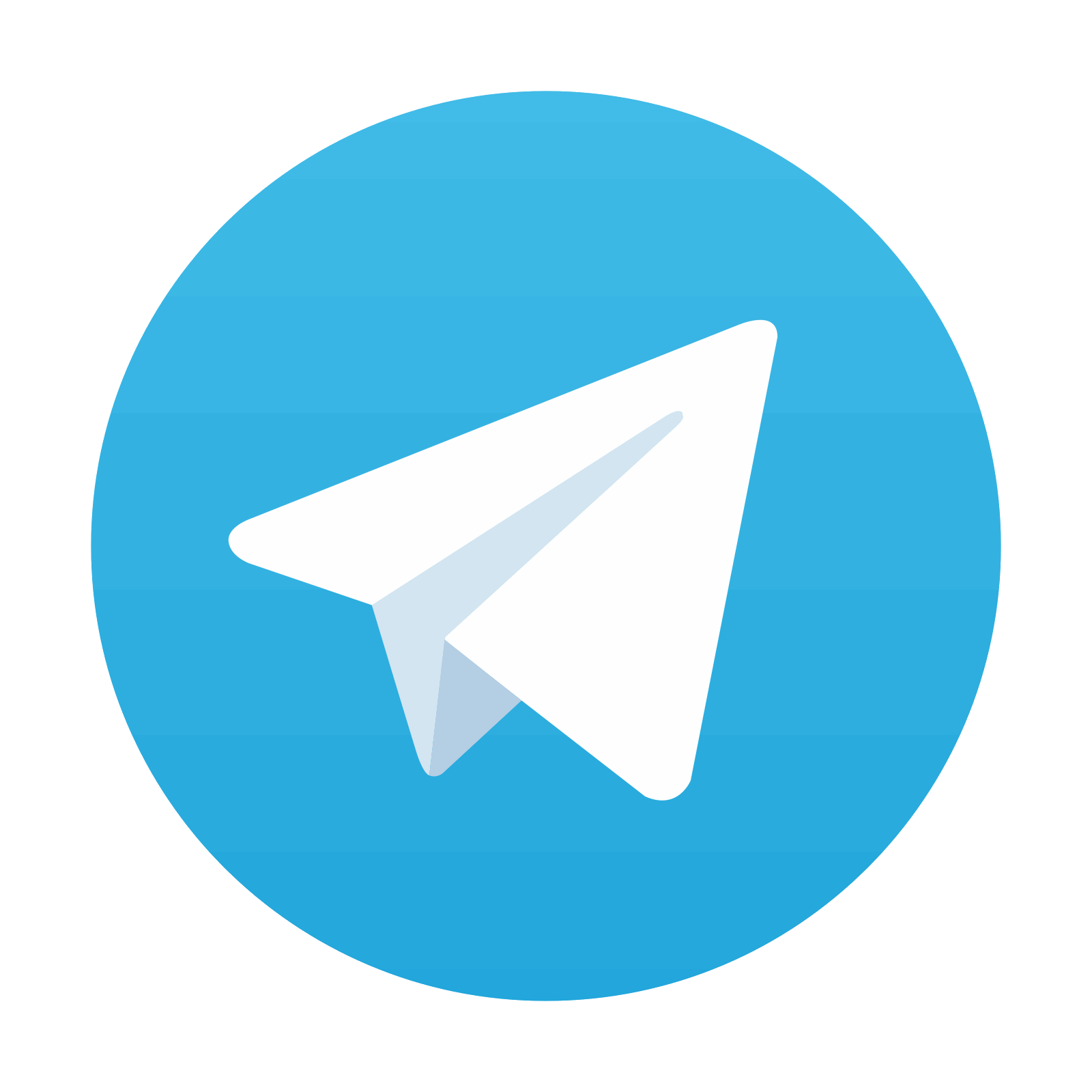
Stay updated, free articles. Join our Telegram channel

Full access? Get Clinical Tree
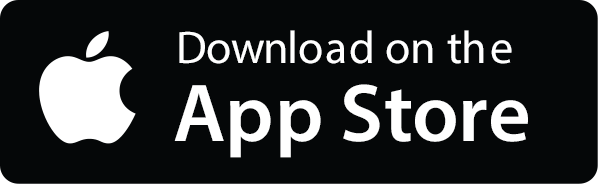
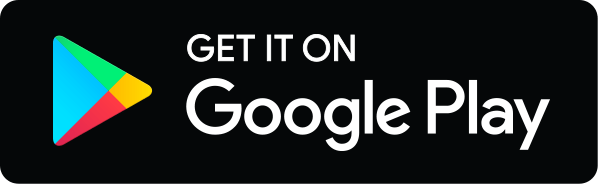
