Intercurrent illness (with acute phase inflammation)
Increased VLDL (TG), reduced HDL-C, LDL-C
Hemoconcentration (dehydration, upright posture, prolonged tourniquet, squeezing to obtain fingerprick sample)
Proportionate increase in most analytes, including lipoproteins
Medications, menstrual cycle
Variable
Winter/summer
LDL-C lower in summer
Stress
Small unexplained increase is LDL-C reported
Food or caloric intake
Increased chylomicrons (TG) sufficient to undermine standardisation for purposes of classification and LDL-C calculation
Hemolysis or analytical delay
Predominantly affects other analytes (e.g. glucose and potassium levels) rather than lipids
Lipaemia
May require dilution
May interfere with turbidimetric analysis of Apolipoproteins
Presence and type of anticoagulant
Direct HDL-C now less likely to be affected
One of the most clinically relevant sources of variability is the presence of intercurrent illness because the associated inflammatory response modifies the lipid and lipoprotein profile. It is important to note that the lipoprotein response to intercurrent illness shares some of the features of that associated with Type 2 diabetes, as will be described later. The magnitude of modifications associated with an inflammatory response is usually proportional to the severity of the underlying illness [12], but proportionately smaller responses should also be anticipated in association with minor intercurrent illnesses [13].
Routine Lipoprotein Assessment
Clinical evaluation of lipoprotein metabolism in diabetes usually involves the measurement of total cholesterol, HDL-C and TG following a 12-h fast. LDL-C is derived from the fasting results by application of the Friedewald equation [14] [LDL-C in mg/dl = TC−HDL-C−(TG/5), LDL-C in mmol/l = TC−HDL-C−(TG/2.2)], but this calculation becomes less reliable as TG levels increase beyond approximately 4 mmol/l (350 mg/dl). Non-fasting samples have been shown to be a more sensitive marker for the detection of individuals with increased risk of CVD [15], but the unstandardized nature of non-fasting samples [16] makes them unsuitable for the characterization or serial monitoring of lipid status in diabetes. Indeed, even fasting levels of TG and other lipids show considerable within-individual variability [17]. This has implications for the serial measurement of LDL-C and the fasting TG from which it was calculated. A change in a serial measurement can only be attributed to clinical factors if it is greater than would be expected due to other sources of variability [18]. The considerable biological variability of fasting TG will increase the proportion by which a serial measurement of fasting TG (and hence LDL-C) must differ in order to indicate a clinically significant alteration.
Increased levels of TRL may also cause variable interference with automated “direct” HDL-C measurements due to TRL cholesterol content. This may have resulted in a positive bias in the past. Method comparison studies prior to 2000 suggested good agreement between “separation” HDL-C methods and the reference method [19], even in the presence of Intralipid [20] or TRL [21]. Where positive bias occurred, it was attributed to incomplete precipitation with the comparator method [22–24] or the presence of Apolipoprotein E-containing HDL [25], but the sources of TG used in these studies had a relatively low cholesterol content. “Direct” HDL methods initially involved the use of α-cyclodextrin, and positive interference from TRL was described in some [26], but not all [23] studies. Since methods involving α-cyclodextrin have been superseded, several recent studies of “direct” HDL methods have reported positive biases which were attributed to TRL [27] or the presence of diabetes [28]. This is an important issue because any overestimation of HDL-C leads to a risk of under-diagnosis of the metabolic syndrome and insulin resistance, as well as under-calculation of LDL-C and NHDL-C. These combined effects would result in a substantial underestimation of absolute risk of CVD, leading to loss of opportunity to effectively identify and treat patients on the basis of their metabolic risk factors. It is possible that TRL may also interfere with “direct” LDL-C assays that utilize a similar strategy based on selective effect of detergents [29].
The accuracy of standard lipid measurements is extremely important because this quantitative information is applied directly to patient management. The atherogenic effect of LDL-C and other Apo B-containing lipoproteins and the probable anti-atherosclerotic effects of HDL-C represent independent risk factors for CVD. Whereas LDL-C (or TC) originally provided thresholds for initiation of treatment and targets for management, management decisions are now seen in a wider context that encompasses the overall (absolute) CVD risk of the patient. This incorporates the classic modifiable and non-modifiable risk factors to varying extents. The predominance of age is one of several inevitable limitations to the performance of the absolute risk calculation algorithms. Diabetes is no longer regarded as “coronary risk equivalent”, but rather the presence or absence of diabetes is treated as a categorical variable, usually without adjustment for severity. Clinical uncertainty associated with intermediate levels of CVD risk has led to efforts to “reclassify” patients in this category by a variety of methods. Consequently, some algorithms allow adjustment for factors such as ethnicity, duration of diabetes, HbA1C level and the presence or absence of microalbuminuria (e.g. www.yourheartforecast.co.nz/). Whilst the additional CVD risk posed by the presence of diabetes often justifies active management of the lipid profile, clinicians need to remember that the presence of massive hypertriglyceridemia poses the more immediate risk of acute pancreatitis.
LDL Composition and Particle Number
Clinical decision-making based purely on quantitative assessment of LDL-C and HDL-C is no longer appropriate, particularly in the presence of elevated TG, which is often the case in Type 2 diabetes. Increased levels of TRL promote the action of cholesteryl ester transfer protein (CETP), which leads to a reduction in HDL-C levels and a depletion in the amount of cholesterol carried per LDL particle. The extent of this process may depend on the severity of postprandial lipemia [30] such that these changes in HDL-C and LDL composition [31] are not completely reflected by the accompanying fasting TG level. Furthermore, the relationship between LDL-C and CVD risk becomes confounded [32] because the formation of “small dense LDL” results in an LDL-C concentration that is low relative to the number of LDL particles. This is illustrated by the superiority of other risk markers [33] such as NHDL-C (calculated as the difference between TC and HDL-C levels) which reflects the full range of potentially atherogenic lipoproteins. This superiority is thought to reflect the greater atherogenicity of the “small dense LDL” and hence the pre-eminence of particle number as the main determinant of the pro-atherogenic effect on non-HDL lipoproteins [34]. This conclusion is based on quantitative ultracentrifuge studies which are usually too tedious to perform for clinical purposes. Electrophoresis based on sizing gel techniques has attempted to circumvent this problem, leading to designation of so-called “pattern A” and “pattern B” profiles or estimations of LDL diameter. These methods are non-quantitative with respect to the number of atherogenic lipoprotein particles, so their clinical value may be marginal.
A more promising approach is based on the measurement of serum Apo B level [35]. All particles that contain Apo B (including chylomicrons, which contain Apo B48, and VLDL, LDL IDL and Lp(a), which contain Apo B 100) are capable of transporting lipid to peripheral sites and, as such, might be considered potentially atherogenic. All such particles contain a single molecule of Apo B, so Apo B provides a direct measurement of the number of particles. Human Apo B derived from the intestine is the product of post-translational modification (m-RNA editing) that yields a product that consists of the N-terminal fragment that represents 48 % of the complete Apo B protein. These two products are designated Apo B 48 and Apo B 100, respectively. Polyclonal antibodies, or monoclonals targeting the first half of the molecule, can be used to quantify both forms. Apo B levels do not change very much after a meal because the transport of dietary fat is largely accommodated by an increase in TG content, rather than an increase in total Apo B. This also reflects the fact that the number of Apo B 100 particles is large relative to the number of Apo B 48 particles. Hence Apo B measurement need not depend on fasting [36] or the ability to differentiate the Apo B 100 isoform.
The degree to which large TG-rich Apo B-containing particles can directly damage the artery wall is debateable, but all apo B 100-contaiining particles (with the exception of Lp(a)) are potential precursors of LDL. As a result, Apo B 100, which is largely reflected by total Apo B levels, quantitatively represents the pro-atherogenic potential of the lipoprotein profile. Furthermore, the predominance of LDL particles means that Apo B largely reflects LDL particle number. Evidence suggests that Apo B measurement is superior to LDL-C or NHDL-C for CVD risk assessment [37]. When combined with LDL-C measurement, the LDL-C:Apo B ratio can reflect the degree to which cholesterol depletion of LDL has led to the formation of “small, dense LDL”.
Nuclear magnetic resonance (NMR) spectroscopy is a non-destructive analytical technique applied to plasma or serum that may be used to reflect the physical composition of lipoprotein particles, particularly their size and number. Consequently, NMR spectroscopy has been used to provide a more detailed picture of lipoprotein size distributions, including HDL species. The technique is unable to distinguish between LDL and Lp(a). Nevertheless studies suggest that NMR spectroscopy may provide additional benefit in terms of the clinical assessment of lipoprotein-associated CVD risk [38].
Tables 1.2, 1.3, and 1.4 are provided as a means of extending the benefits of Apo B measurement to include diagnosis.
Table 1.2
An algorithm for the prediction of the likely class of lipoproteins responsible for dyslipidemia in approximate order of prevalence in Type 2 diabetes (adapted from de Graaf et al.) [23]
Apolipoprotein B level | TG > 1.5 mg/dl (Y/N) | TG:ApoB ≥10 (Y/N) | TC (mg/dl):ApoB ≥6.2 (Y/N) | Lipoprotein |
---|---|---|---|---|
Apo B <1.2 g/l | N | N | N | Normal |
Apo B <1.2 g/l | Y | N | N | VLDLa |
Apo B ≥ 1.2 g/l | Y | N/A | N/A | LDL and VLDLa, b |
Apo B ≥ 1.2 g/l | N | N/A | N/A | LDLb |
Apo B = 0.75–1.2 g/l | Y | Y | N | Chylomicron and VLDLa |
Apo B <1.2 g/l | Y | N | Y | IDL or “remnants” |
Apo B <0.75 g/l | Y | Y | N | Chylomicrons alonea |
Table 1.3
Causes of secondary dyslipidemia, including diabetes
Excess lipoprotein accumulation | Causes |
---|---|
VLDL | Type 2 diabetes Obesity/insulin resistance Chronic renal impairment Hemodialysis Alcohol excess Estrogen use Glucocorticoid use Retinol analogues Other |
LDL and VLDL | Type 2 diabetes Obesity/insulin resistance Cholestasis Nephrotic syndrome Peritoneal dialysis Systemic lupus erythematosus Polycystic ovary syndrome Glucocorticoid use HIV and highly active antiretroviral therapy Antipsychotic drug use Pregnancy Other |
LDL | Nephrotic syndrome Hypothyroidism Anabolic steroids Other |
Chylomicron and VLDL | Type 2 diabetes Obesity/insulin resistance Chronic renal impairment Alcohol excess Oestrogen use Glucocorticoid use Pregnancy Other |
IDL or “remnants” | Triggered or exacerbated by Type 2 diabetes Obesity/insulin resistance Chronic renal impairment Alcohol excess Oestrogen use Glucocorticoid use Other |
Chylomicrons alone | Acquired Apo C2 deficiency in systemic lupus erythematosus |
Table 1.4
Causes of primary dyslipidemia which may coexist with diabetes
Excess lipoprotein accumulation | Primary causes |
---|---|
VLDL | Polygenic gene/environment interactions Familial hypertriglyceridemia |
LDL and VLDL | Polygenic gene/environment interactions HyperApobetalipoproteinaemia preferred instead of “familial combined hyperlipidemia” |
LDL | Polygenic gene/environment interactions Familial hypercholesterolaemia |
Chylomicron and VLDL | Polygenic gene/environment interactions Exacerbation of familial hypertriglyceridemia or familial hyperchylomicronaemia |
IDL or “remnants” | Dysbetalipoproteinaemia |
Chylomicron | Familial hyperchylomicronaemia |
Etiological Assessment
The clinical implications of dyslipidemia depend on the type of lipoprotein responsible for the alteration in lipid levels and the etiological reason for its accumulation. The atherogenic effect of various lipoproteins may differ depending on the etiological context in which they arise, and it should not be assumed that the lipoprotein profile in Type 1 or Type 2 diabetes is solely and necessarily based on that condition alone. Other secondary causes may modify the lipoprotein abnormality, whilst intercurrent primary lipoprotein disorders may influence or even dictate the lipoprotein profile. Tables 1.2, 1.3, and 1.4 provide a framework for diagnostic considerations that may modify clinical management. The first step in this process is the consideration of which lipoprotein class is responsible for any dyslipidemia in a person with diabetes. Though this may be inferred from the results of the automated laboratory tests, it cannot be relied upon. Traditionally, identification of the excess lipoproteins was achieved by lipid electrophoresis, but this non-quantitative method does little to enhance prognostic information. Tables 1.2, 1.3, and 1.4 present an extension of the use of Apo B levels to provide this information in an alternative and potentially more useful format [35]. The different lipoprotein patterns are presented in approximate order of their prevalence in Type 2 diabetes, but as will be explained, the first four are somewhat interchangeable. The last two are substantially less common.
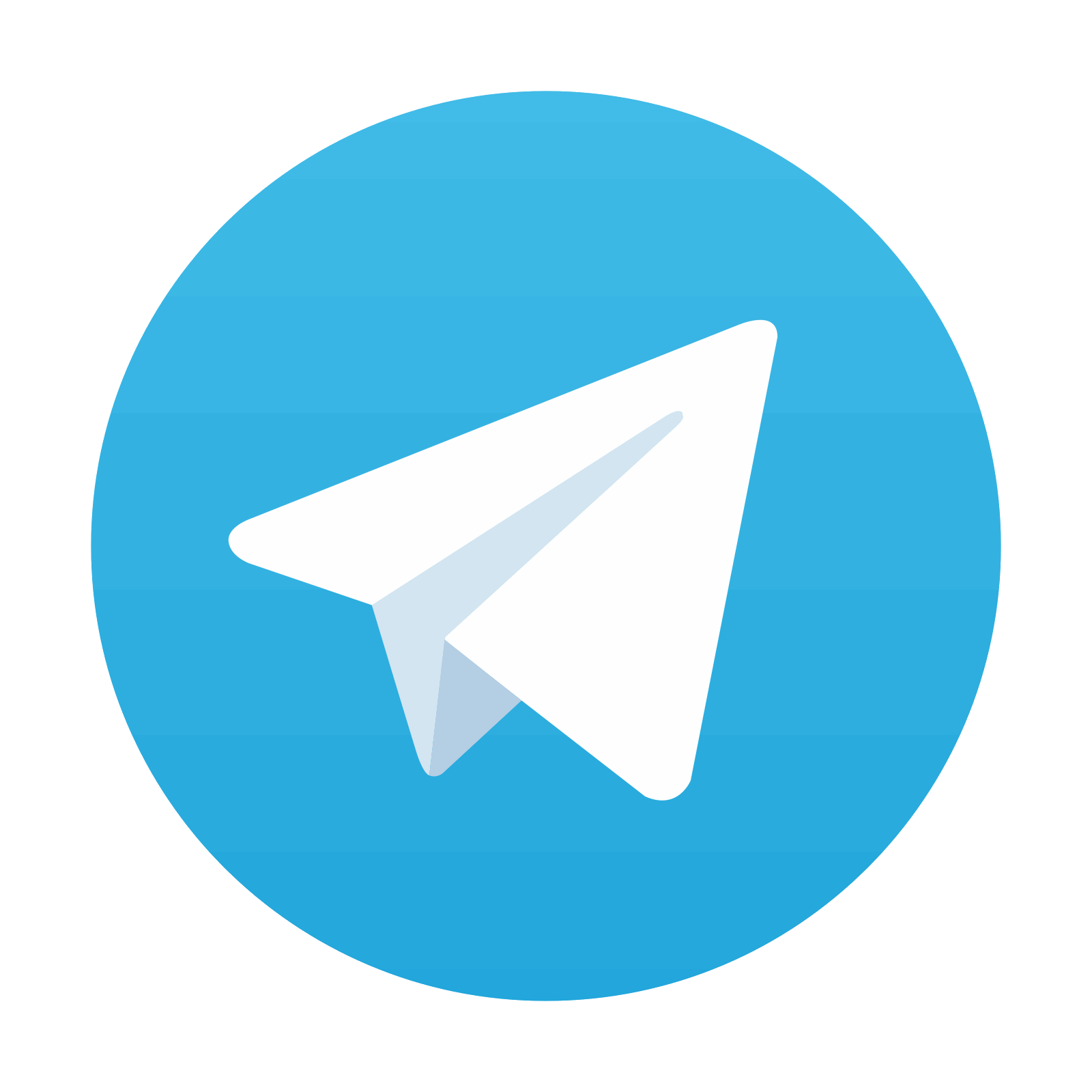
Stay updated, free articles. Join our Telegram channel

Full access? Get Clinical Tree
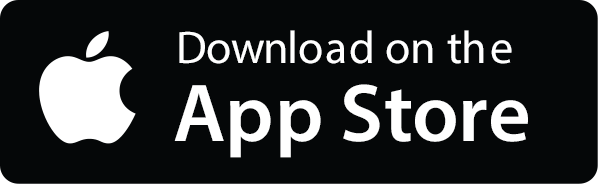
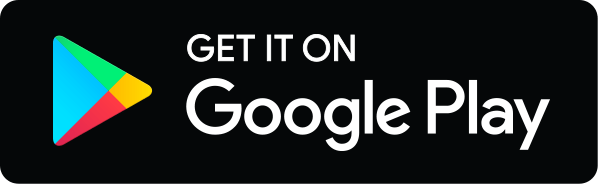