Intraoperative Radiotherapy
Intraoperative radiotherapy (IORT) involves the delivery of radiation during surgery using various types of radiation sources/technologies, including intraoperative electron radiation therapy (IOERT), high-dose-rate brachytherapy (HDR-IORT), and electronic brachytherapy/low-kilovoltage x-rays (KV-IORT). The major advantage of IORT is the potential for delivery of higher effective radiation doses to a tumor or, more ideally, to the bed of a grossly resected tumor, while limiting the radiation dose to adjacent, dose-limiting, normal tissues or organs. This normal tissue sparing is accomplished by the dosimetric features of these different IORT technologies and by surgical approaches and/or customized lead shielding to exclude/limit normal tissues during IORT. Although IORT may be used alone following resection of certain primary or recurrent cancers, it is more often (and ideally) combined with external beam radiotherapy (EBRT), typically administered preoperatively with or without concurrent chemotherapy. Indeed, based on clinical IORT data generated over the last three decades, one can conclude that the acute and late normal tissue toxicities and local tumor control rates are quite acceptable in patients with a variety of solid cancers treated with curative intent combining EBRT (± chemotherapy) with gross total resection and IORT. Effective use of IORT requires a multidisciplinary approach, including close cooperation with surgical oncology, medical physics, and surgical nursing personnel. However, despite encouraging clinical data and more widespread use of IORT throughout the world over the last decade, level 1 evidence based on large phase III trials is lacking, with the possible exception of its use in the treatment of breast cancer.
This chapter begins with an overview of the radiobiology of IORT, based largely on comprehensive preclinical experiments using canine models that established normal tissue and organ-specific guidelines, principally for IOERT ± EBRT. Using American foxhounds and beagles as models, acute and late normal tissues responses to IOERT ± EBRT were categorized using experimental designs that mimicked thoracic and abdominal cavity surgeries where IOERT was anticipated to be used clinically. Next, the physics and technical applications of IORT are described with details regarding the specific uses of IOERT, HDR-IORT, and KV-IORT. Finally, a summary of the available clinical IORT data is presented for some tumor sites where IORT has shown efficacy as a component of multimodality treatment. A discussion of potential future clinical uses of IORT is also presented in this final section.
RADIOBIOLOGY PRINCIPLES AND EXPERIMENTAL STUDIES OF IORT
Radiobiologic Modeling for the Determination of Equivalent Single-Fraction IORT Doses
The principal advantage of IORT is the ability to maximally exclude adjacent normal tissues by surgical mobilization and/or the use of customized lead wafer shielding in the cases of IOERT and HDR-IORT. However, the clinical application of IORT to treat minimal gross (R2 resection) or suspected microscopic residual disease (R1 resection) in a resected tumor bed necessitates inclusion of partial volumes of adjacent normal tissues or organs based on tumor location, tumor size, and extent of infiltration of adjacent normal tissues, including regional lymph node basins. Based on typical in vitro clonogenic studies and in vivo small animal studies, where large single doses of 5 to 20 Gy have often been used, it is evident that IORT doses of 15 to 25 Gy could provide a theoretical disadvantage with respect to IORT-related tumor cell kill (or local control) versus normal tissue toxicities.1,2
Typically, the radiation dose–response curve for acute reacting normal tissues, such as gastrointestinal epithelium and bone marrow, is very steep, and a small change in the radiation dose near the tissue tolerance level can result in a significant risk of toxicity. In contrast, the radiation dose–response curve for late-reacting normal tissues, such as heart muscle, peripheral nerve, large arterial blood vessels, and bone, is less steep and correlates directly with the volume of tissue included within the irradiated field. Extrapolating from the linear-quadratic model using clonogenic survival data, one can extrapolate the shape of the radiation survival curve for tumor and normal tissues using the α/β ratio. A late-reacting normal tissue has a low α/β ratio (usually <5 Gy), whereas acute-reacting normal tissues, as well as most solid tumors, have α/β ratio of greater than 7 Gy. Using these α/β ratios, one can estimate the single-fraction IORT dose (compared to conventional fractionated EBRT) to control tumor while limiting specific normal tissue toxicities. For example, for a squamous cell carcinoma with minimal residual disease following surgery, an EBRT dose of 60 Gy using 2-Gy fractions (D2Gy = 60) is required, and the α/β ratio for squamous cell carcinoma cells is 10 Gy. We can use the formula
DIORT = ½[(α/β)2 + 4D2Gy(α/β + 2)0.5 − α/β]
to calculate the equivalent DIORT to be 22.3 Gy, which is supported by multiple single-institution IORT clinical studies that included patients with recurrent or locally advanced head and neck cancers.3 Similarly, such an equivalent IORT dose can be calculated for a late-reacting normal tissue, such as peripheral nerve, using D2Gy = 70 and α/β ratio = 2 Gy. The calculated DIORT is 16 Gy, which is again supported by experimental canine and human clinical data.4–6
Although the DIORT calculation has some clinical utility, as these two examples illustrate, many other clinical variables are not factored into the calculation, such as prior or recent EBRT ± chemotherapy; the inclusion of multiple types and volumes of acute- and late-reacting normal tissues within the IORT field; and other patient-specific comorbidities, for example, cardiovascular disease, diabetes, and connective tissue disease. Indeed, in patients treated with preoperative or postoperative EBRT ± chemotherapy, the IORT boost dose calculation should include these additional treatment effects, particularly with regard to both acute- and late-reacting normal tissues. There are experimental canine data, specifically from the beagle dog model, to assist in the DIORT boost calculation, which is often in the 10- to 15-Gy range. In addition, from a radiobiologic perspective, it is recognized that tumor cell hypoxia is the major factor determining tumor radioresponse, as other biologic factors, such as repair, reoxygenation, and repopulation, are not operative with single-fraction treatment.
Another radiobiologic effect that is not included within the DIORT calculation for normal tissue toxicity is the risk of a radiation-induced cancer. Radiation-induced cancers are typically a late effect, often occurring a decade or more after radiation therapy. For an IORT-induced cancer, the cancer should arise within the IORT field and should have a different histology than the original primary. In the canine studies of normal tissue tolerance to varying IORT doses, long-term follow-up (up to 5 years) demonstrated IORT-induced sarcomas of bone and soft tissue, evident at autopsy, in up to 20% to 25% of animals receiving greater than 25 Gy.7 No IORT-induced cancers have been reported in humans, although many IORT-treated patients have very aggressive primary or recurrent cancers and often die from progressive metastatic disease within 1 to 2 years after IORT without autopsy evaluation of the IORT volume. Because IORT is being used for some pediatric oncology cases, it will be especially important to follow these patients closely for the potential risk of an IORT-induced cancer as a late effect.
Experimental Studies of IOERT with or without EBRT to Establish Normal Tissue Tolerance Guidelines
Comprehensive studies of acute and late normal tissue responses to IOERT ± EBRT were performed using two canine models. The American foxhound model was principally used for IOERT studies at the National Cancer Institute (NCI), whereas a beagle dog model was used for IOERT ± EBRT studies at Colorado State University (CSU). Both canine species are large enough to allow surgical procedures in the thoracic and abdominal cavities that mimic surgeries for human cancers for which IORT may be applied. The goal of these studies was to establish dose guidelines of IOERT alone (NCI) and IOERT ± EBRT (CSU) to reduce acute and late normal tissue toxicities in selected intact and surgically manipulated tissues that mimicked clinical situations in which IOERT might be used. After IOERT ± EBRT, dogs were closely followed clinically and by various sequential diagnostic studies for up to 5 years. All dogs were subjected to complete autopsy with detailed histologic analyses of irradiated tissues.
A description of the NCI IOERT studies is presented in Table 18.1. A more comprehensive overview of these studies is summarized in two reviews.8,9 A total of 13 sites or target tissues were studied using escalating IOERT doses and a fixed IOERT volume based on the target site. In total, these NCI studies involved 227 dogs, with 196 receiving IOERT and 31 receiving sham IOERT (0 Gy). At CSU, adult beagles were randomized to receive IOERT alone (5 × 8 cm field; 6 MeV; dose range 17.5 to 55 Gy); EBRT alone (5 × 10 cm field; 6-MV photons; dose range 60, 70, and 80 Gy in 30 fractions of 2, 2.33, and 2.67 Gy, respectively); or initial EBRT (5 × 10 cm field; 6-MV photons; dose 50 Gy in 25 fractions of 2 Gy) followed by IOERT (5 × 8 cm field; 6 MeV; dose range 10 to 45.5 Gy). A summary of the clinical and pathologic data regarding normal tissue tolerance from these collective NCI and CSU canine studies are presented in Table 18.2 and briefly described below.
Vascular Tissues
Because the vasculature determines, in large measure, the viability and functionality of all normal tissue systems, an understanding of the tolerance thresholds of IORT on blood vessels is critically important. Intact large vessels (aorta, vena cava) appear to tolerate large single IOERT doses without significant clinical sequelae, based on serial follow-up radiographic studies for up to 5 years.10 At autopsy, no pathologic changes were found following a 20-Gy dose, and only mild to moderate subintimal fibrosis was found after 30- and 40-Gy doses, respectively. However, the combination of 50-Gy EBRT and >20-Gy IOERT resulted in an IOERT dose-related luminal narrowing with thrombus formation and moderate mural fibrosis pathologically, with up to 2 years of follow-up.11 To evaluate the IORT tolerance of intraoperatively mandated vascular repairs and anastomoses, a transection and end-to-end reanastomosis of the infrarenal abdominal aorta was performed, followed by IOERT doses of 20 to 45 Gy.10 Pathologically, moderate medial wall fibrosis was found at doses greater than 30 Gy. Although anastomotic integrity was maintained at all doses, follow-up arteriograms showed anastomotic occlusion at doses greater than 30 Gy within 6 to 12 months. However, occlusion was sufficiently slow to allow formation of arterial collaterals around the occlusion, preventing any clinical or pathologic signs of ischemia distal to the anastomosis. At 45 Gy, development of a late arteriovenous fistula at the anastomotic site in one dog suggested the potential for IORT dose-limiting, clinically relevant, toxicity.
The tolerance of vascular grafts to IORT was investigated in beagles, for which a segmental resection of the infrarenal aorta was performed with reconstruction using a polyfluorotetraethlene graft.12 IOERT up to 30 Gy was immediately delivered following the prosthetic grafting, and half of the dogs were randomized to receive postoperative EBRT to 36 Gy in 10 fractions over 4 weeks. Arterial graft occlusion occurred acutely in both treatment groups but was correctable by thrombectomy. During clinical follow-up, most dogs receiving ≥30-Gy IOERT ± EBRT developed late graft occlusion (within 12 months) but again showed evidence of progressive collateralization by arteriography, preventing distal ischemia–related complications.
TABLE 18.1 NATIONAL CANCER INSTITUTE INTRAOPERATIVE ELECTRON RADIATION THERAPY STUDIES OF NORMAL TISSUE TOLERANCE
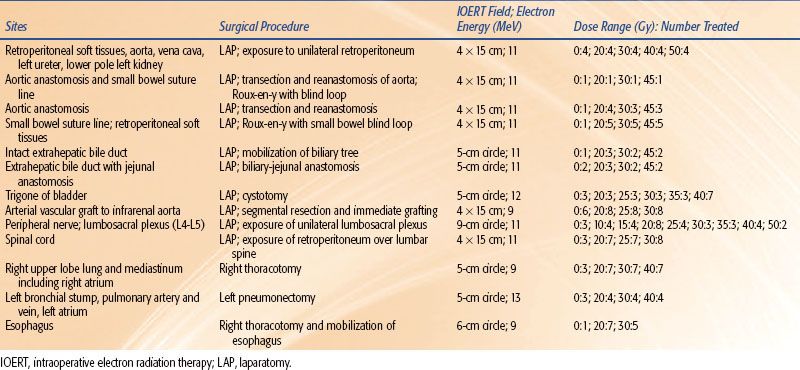
TABLE 18.2 TOLERANCE DOSES TO INTRAOPERATIVE RADIOTHERAPY IN CANINE MODELS
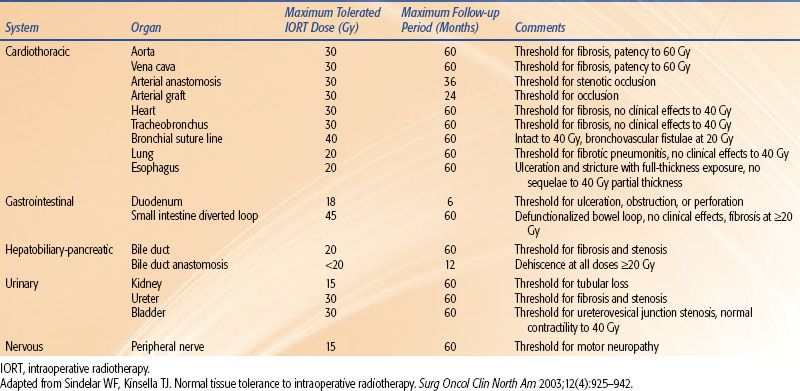
Gastrointestinal Tissues
Due to its rapidly proliferating mucosa and rich blood supply, the small and large intestines are among the most radiosensitive normal tissues. As such, the intestine needs to be surgically mobilized and excluded from IORT portals. NCI investigators assessed the IORT tolerance of small intestinal suture lines where a jejunal blind loop was created surgically and intestinal continuity maintained by distal jejunojejunostomy.13 The IOERT field encompassed the suture line and the full thickness of the intestinal wall, and doses of 20 to 45 Gy were delivered. No immediate histologic changes were seen, and no clinical evidence of suture line dehiscence was found with 5 years of follow-up. However, internal interloop fistulae occurred following 45 Gy. Thus, whereas acute IOERT tolerance of a defunctionalized bowel loop was acceptable to 45 Gy, chronic complications suggest a maximum of 30 Gy. However, functional small and large bowel showed clinical and pathologic manifestations of ulceration, stricture, and perforations following doses as low as 15 to 20 Gy.
Hepatobiliary Tissues
Bile duct tolerance to IOERT was studied at the NCI using a 5-cm IORT portal to the subhepatic space, which encompassed the extrahepatic bile duct.14 When using a dose range of 20 to 45 Gy, no acute complications were noted, but progressive and IOERT dose-related chemical evidence of partial biliary obstruction was seen within 2 to 8 months of follow-up. Frank biliary cirrhosis developed in one-half of dogs receiving 30 Gy or more that were followed for a minimum of 12 months. The tolerance of biliary-enteric anastomoses was also studied using a similar IOERT dose range. After laparotomy and formation of a jejunal biliary loop, the bile duct was transected and anastomosed to the jejunal loop, followed by immediate IOERT to the anastomotic site. All animals developed acute complications within several weeks, with either anastomotic disruption or subacute anastomotic obstruction, resulting in cholangitis or bile duct necrosis. Thus, although the intact bile duct may tolerate doses of up to 20 Gy, a bile duct anastomosis cannot be included within an IORT field.
Urinary Tract Tissues
In the IOERT study involving the intact unilateral retroperitoneum,10 a 12-cm segment of ureter, as well as the inferior pole of one kidney, were irradiated to IOERT doses as high as 50 Gy. Dogs were followed clinically with renal function testing and radiographic pyelography. The kidney showed dose-related fibrosis and hyalinization. Acute obstructive nephropathy secondary to ureteral obstruction was evident with 50 Gy, whereas subacute obstructive nephropathy was evident at 2 to 3 months of follow-up in the 40-Gy dogs and at 6 to 12 months following 30 Gy. In the 20 Gy–treated dogs, no clinical or pathologic changes in the irradiated ureter were evident. A CSU study showed that shorter (≤4 cm) segments of ureter would tolerate doses of 30 Gy.15 Typically, the ureter can be surgically mobilized and excluded from the IORT field, but segments at high risk of residual microscopic disease may be included, and prophylactic ureteral stenting may reduce the risk of narrowing/obstruction as a subacute complication.
Bladder tolerance to IOERT was studied using the foxhound model at the NCI.16,17 Following laparotomy and cystotomy, a 5-cm circular IORT portal, including the trigone and both ureteral orifices, was treated with doses of 20 to 40 Gy. Dogs were then followed for up to 5 years with clinical evaluation, kidney function tests, intravenous pyelography, and cystometry. Although no acute complications were noted, some dogs who received 25 Gy or greater developed fibrotic strictures at the ureterovesical junction within 2 years, which became more frequent at 5 years in the ≥30 Gy–treated dogs. Whereas pathologic changes of fibrosis and microvascular changes in the bladder wall were evident at 30 Gy or greater, follow-up cystometric studies showed little difference in post-IOERT bladder contractility from baseline at all IOERT doses.
Cardiothoracic Tissues
In the NCI studies of IOERT to the canine mediastinum, atrial appendages of the heart received doses up to 40 Gy.18,19 Dense fibrosis of the myocardium was found pathologically following IOERT doses of 30 Gy or greater, with early (within 1 month) medial hyaline degeneration followed by radiation vasculopathy and infarction within 12 months. However, due to the limited irradiated volume of cardiac muscle, no clinical signs of cardiac failure were evident with up to 5 years of follow-up.
Segments of the trachea and main stem bronchi also received IOERT of 20 to 40 Gy in the NCI mediastinal IOERT studies.18,19 Pathologic changes of fibrosis in the tracheobronchial wall were evident at doses of 30 Gy or greater, with infrequent development of chondronecrosis of the tracheal rings at doses of less than 40 Gy; however, most animals showed no clinical respiratory symptoms. In addition, the canine pneumonectomy study found normal healing of the bronchial stump following IOERT to 20 to 40 Gy, and no clinical sequelae were observed through 5 years of follow-up.20 However, when limited amounts of lung tissue received IOERT, acute confluent pneumonitis progressing to interstitial fibrosis and pulmonary arteriolar sclerosis developed at all doses within 12 months.18,20
IOERT tolerance of the esophagus was also evaluated in the NCI mediastinal studies, with both full-thickness and partial-thickness (<50% of circumference) treatment.18,19 Clinical examinations, barium swallows, and esophagoscopies were performed for up to 2 years following doses to 30 Gy for full-thickness irradiation and to 40 Gy for partial-thickness irradiation. With full-thickness esophageal IOERT, all animals receiving 20 Gy or more showed acute clinical toxicity with signs of dysphagia and weight loss. Acute, but transient, inflammatory changes to esophageal mucosa were found at 20 Gy, but progressive severe inflammatory changes leading to ulceration and stricture by 2 to 3 months were found following 30 Gy. With partial esophageal wall IOERT, no severe clinical or radiographic sequelae occurred at doses up to 40 Gy, with dose-related fibrosis but no mucosal ulcers or strictures.
Nervous Tissue
Whereas peripheral nerves were traditionally considered to be relatively radioresistant, clinical IORT trials at the NCI reported a significantly increased risk of lumbosacral neuropathy following IOERT + EBRT in patients with localized but technically resectable retroperitoneal sarcomas.5,21 In this clinical trial, patients were randomized to receive postoperative EBRT alone (initial 40 Gy, followed by a 14- to 16-Gy boost) or IOERT (20 Gy using multiple abutting fields based on tumor bed size/location) followed by postoperative EBRT (36 Gy). Subacute (within 1 to 3 months) and late (>6 months) clinical motor and/or sensory neuropathies to the lower trunk and/or lower extremity were found in 9 of 15 patients randomized to IOERT + EBRT and in 1 of 20 patients randomized to postoperative EBRT alone (p < .01).22
To further establish the tolerance of peripheral nerve trunks to IORT, a series of studies was conducted at the NCI and CSU.4–6,22–24 In the initial NCI study using foxhounds, the lumbosacral plexus was surgically exposed and IOERT doses of 20 to 75 Gy were delivered.5 Hind limb motor changes developed in 90% of treated dogs within 12 months, ranging in severity from slight motor strength weakness to complete motor paralysis, with an approximately inverse relationship between IOERT dose and the time to onset and severity of neurologic signs. However, no threshold dose for neuropathy was found, even with the use of nerve conduction times as a measure. Pathologically, loss of large nerve fibers and perineural fibrosis with radiation vasculopathy in perineural connective tissue were seen. A follow-up NCI trial attempted to establish a threshold dose for IORT-induced neuropathy in the lumbar-sacral plexus.4 No animal receiving IOERT doses of up to 15 Gy developed clinical neuropathy by exam and nerve conduction testing for more than 3 years following IOERT, whereas all animals receiving 20 Gy developed hind leg paresis with lower nerve conduction testing within 12 months. With up to 5 years of follow-up, dogs treated with IORT doses of up to 15 Gy showed continued lack of neurotoxicity.22
Studies assessing lumbar nerve injury following IOERT alone, EBRT alone, and combined IOERT plus EBRT were performed at CSU using a beagle dog model.6,23,24 Similar neurologic and nerve conduction testing as used in the NCI studies were performed for up to 2 years of follow-up. Although no neurologic complications were found using EBRT alone, dose- and time-related neuropathy was seen in IOERT alone– and in IOERT + EBRT–treated dogs with an IOERT dose threshold of 15 Gy. Radiation-induced changes to Schwann cells and nerve vasculature were found, similar to the NCI findings, and ultrastructural studies suggested microvascular damage causing regional hypoxia and subsequent nerve fiber loss. Thus, peripheral nerve appears to be a dose-limiting tissue for IOERT, in contrast to the relative radioresistance of peripheral nerves to EBRT.
IORT-Induced Malignancies
As mentioned previously, radiation-induced malignancies are infrequent following conventionally fractionated EBRT and are most commonly seen several to many years after the successful treatment of childhood cancers. Although IORT-induced cancers have not been reported in humans, the canine data support a potential risk in humans. Again, an IORT-induced cancer should fulfill several criteria, including occurring within the IORT field, developing after an appropriate latency period (e.g., a few years in canines), and being histologically confirmed and of a histologic type that develops infrequently in the particular dog species.
In studies of IOERT-related toxicity to bone at CSU, a 21% incidence of osteosarcoma was noted, with at least 4 years of follow-up following IOERT alone to greater than 25 Gy with or without EBRT.25 In dogs treated with EBRT alone for spontaneous soft tissue tumors, a 3% incidence of osteosarcoma within the EBRT volume was seen. IOERT-induced tumors were also seen in the NCI canine trials.7,26 Among 59 animals followed for 2 or more years, 12 tumors were pathologically confirmed at complete necropsy. Three tumors did not meet the criteria for being radiation induced, including 2 benign fibrous tumors and a mammary carcinoma. The 9 IORT-related tumors included a bladder rhabdomyosarcoma, a soft tissue malignant fibrous histiocytoma, and 7 sarcomas of bone or cartilage. In dogs receiving >25-Gy IOERT, the overall incidence of radiation-induced neoplasms was 25%, consistent with the CSU data.
In summary, the validity of the canine tissue tolerance models as described in this section and Table 18.2 as representative of the human tissue response to IORT is supported by clinical IORT data from human trials. Although the tolerance to IORT-induced acute and late toxicities can vary considerably between tissues, doses up to 20 Gy are generally tolerated. The general principle providing the rationale for IORT should always be practiced, that is, maximize the radiation dose to the tumor or, ideally, the bed of a grossly resected tumor while minimizing dose exposure to adjacent normal tissues.
PHYSICS AND TECHNICAL ASPECTS OF IORT
Definition of Techniques
IORT involves the use of a single fraction of radiation therapy delivered while the patient is under anesthesia. Three different technologies can be used to deliver IORT, including electrons (IOERT), high-dose brachytherapy with iridium-192 (192Ir; HDR-IORT), and low-kV x-rays (KV-IORT). IOERT is delivered by a linear accelerator electron beam in the 6- to 15-MeV dose range. Although the use of a conventional linear accelerator in the radiation oncology department was the standard for IOERT delivery in the 1970s and early 1980s, the subsequent development of mobile linear accelerators that are transported to the operating room (OR), or the installation of a dedicated accelerator in an OR, improved the efficiency and use of IOERT, no longer requiring the patient to be transported under anesthesia. At present, there are three commercial mobile linear accelerators designed for IOERT, including the Mobetron (4- to 12-MeV electrons; IntraOp Medical, Sunnyvale, CA), the Novac 7 (4- to 10-MeV electrons; NRT SpA, Rome, Italy), and the LIAC (4- to 10-MeV electrons; Sordina SpA, Saonara, Italy). HDR-IORT uses a high-dose-rate afterloader with 192Ir that decays via β or electron capture, and the daughter isotopes are short lived, emitting γ-rays of various energies. The β decay is absorbed by the source capsule, and the average photon energy emitted is 370 keV with a 74-day half-life. HDR-IORT is feasible only after a near gross total resection, as the maximum depth of coverage is typically 0.5 cm deep from the surface of the resected tumor bed. Several 192Ir HDR remote afterloaders are commercially marketed by Nucleotron (Veenendaal, Netherlands) and by Varian Medical Systems (Crowley, United Kingdom). A shielded OR is required for HDR-IORT. Over the last decade, mobile IORT devices using low-kV x-rays (KV-IORT) have been developed. Low-kV x-rays (20 to 50 kV) have the advantage of a steep dose gradient, not requiring specially shielded ORs, but have a major disadvantage in that the target (tumor bed) should ideally be spherical in shape with a maximum tissue treatment radius of 1 to 2 cm. Two commercial low-KV-IORT devices are available, including the Zeiss Intrabeam (Zeiss Surgical, Oberkochen, Germany) and the Xoft S700 Axxent System (Zoft, Medford, MA).
IOERT: Physics and Technical Applications
With the development of mobile linear accelerators as described earlier, no permanent OR shielding is required, unlike a fixed OR-based linear accelerator, because the maximum electron beam energy is 12 MeV or less and these mobile linear accelerators are designed without bending magnets. In addition, neutron contamination is not a problem for OR personnel or patients. The Mobetron has a fixed beam stopper, whereas the Novac 7 and LIAC accelerators are equipped with mobile beam stoppers. The 90% depth dose in water ranges from 1.1 cm for 4-MeV electrons to 3.5 cm for 12-MeV electrons. A variety of applicator shapes (e.g., circular, rectangular) and sizes are available from the manufacturers. However, to commission a machine for IORT, a minimum set of dosimetry measurements is required for each applicator and each electron energy including percentage depth dose, beam profiles in two orthogonal planes, isodose curves in two orthogonal planes, and applicator ratios. The applicator ratios are compared to a reference applicator for which the accelerator output is calibrated.
Quality assurance (QA) procedures for mobile IOERT linear accelerators are outlined in detail in the reports of the American Association of Physicists in Medicine (AAPM) Radiation Therapy Task Groups 48 and 72.27,28 Because any malfunction of these mobile accelerators could result in a patient not receiving the scheduled IOERT treatment or in having a patient’s surgery delayed, a high level of QA requires calibration of all electron energies early on the day of surgery, in addition to a rigorous process of periodic checks similar to the QA for a standard linear accelerator. Unique factors to the mobile IOERT linear accelerators are the lack of adjustable collimators and bending magnets. QA checks are necessary of the alignment of the soft docking system as used in the Mobetron and of a hard docking system for the two other mobile accelerators. Although in vivo dosimetry using TLDs or silicon diodes is not feasible for placement in a sterile surgical resection bed during IOERT, offline procedures such as the use of radiochromic films or real-time methods such as the use of metal oxide semiconductor field effect transistors are being applied to breast cancer29,30 IORT and may be applied to other tumor sites.
Mobile IOERT accelerators, however, have limited mobility, requiring the patient and OR table to be moved to the accelerator in the OR and sometimes requiring a change in the patient’s position. The use of special OR tables with fine movement capabilities superiorly, inferiorly, laterally, and pitch and roll positioning, as well as Trendelenburg and reverse Trendelenburg movements, makes IOERT patient positioning and docking much easier and faster. The soft docking process required for the Mobetron is facilitated by a set of lasers in the accelerator head. The hard docking system used with the Novac 7 and LIAC accelerators involves a two-part applicator. Once the appropriate field size and applicator shape are determined, the superior part of the applicator is fixed to the linear accelerator head and then physically mated with the inferior part, which is in contact with the target (tumor bed). Once aligned, a rigid interlocking of the two parts is made prior to IOERT delivery. Typically, the process of soft or hard docking requires less than 10 to 15 minutes.
However, prior to docking, a detailed determination of the IOERT treatment volume is required, involving close collaboration between the surgical oncologist and the radiation oncologist. Typically, surgical sutures or clips are placed by the surgeon to better visualize the tumor bed, and at least a 1-cm margin is added. Next, the size, shape, and degree of bevel for the suitable applicator are determined, as well as determining whether one or more IOERT fields are necessary to cover the tumor bed. Although the IOERT applicators can function as a normal tissue retractor, additional surgical packing is often required to further exclude certain normal tissues, for example, bowel. In the clinical situation in which sensitive normal tissues cannot be physically displaced from the IOERT field secondary shielding using sterilized lead sheets can be placed with appropriate thickness to attenuate 90% or more of the dose. Lead shielding is also essential to prevent any dose overlap, if multiple IOERT fields are required to cover the tumor bed with at least 1-cm margins. Finally, to reduce the accumulation of serous fluid in the tumor bed during IOERT (which would attenuate the dose), placement of suction adjacent to the IOERT applicator is recommended.
HDR-IORT: Physics and Technical Applications
HDR-IORT involves the use of an 192Ir HDR afterloader to deliver a large single fraction of brachytherapy to a grossly resected tumor bed with necessary retraction and physical shielding of adjacent normal tissues, similar to the guidelines for IOERT as described. Because of the high activity of 192Ir afforded by computer-controlled remoter afterloading, HDR-IORT treatment times of 30 to 60 minutes can be accomplished, based on the target (tumor bed) size. The desired dose distribution is generated by superimposing a large number of single-source radiation distributions at different locations and different dwell times. Two AAPM publications from Task Group 43 summarize in detail the dose calculations for 192Ir in air and water.31,32 A recent review of HDR-IORT is also recommended.33
Construction of an HDR-IORT dedicated facility requires a shielded OR equipped with door interlocks, room radiation monitors, and additional adjacent space for the OR team (surgeon, radiation oncologist, nurses) to remain sterile during HDR-IORT, should any emergency reentry be needed. Anesthesia and surgical monitoring via video cameras must also be available in this adjacent OR space. Ideally, construction of a HDR-IORT facility in the hospital OR complex allows for most efficient use of OR personnel and equipment. The QA program for HDR-IORT follows guidelines outlined by AAPM task groups.34 Typically, a series of QA checks is performed 24 hours prior to each HDR-IORT procedure and involves determination of source positioning and source strength, verification of proper functioning of room radiation monitors, and checking of equipment for use in the event of emergency source retraction.
For HDR-IORT, the applicator must be rigid enough to secure the afterloader catheters in a fixed and reproducible position while also conforming to the tumor bed contour. The applicator design must provide adequate thickness to maintain a 0.5-cm distance between the source plane and the treatment surface.33 Prior to HDR-IORT delivery, the radiation oncologist must confirm that the applicator is in direct contact with the tissue surface throughout the entire target area, as a separation of only 0.5 cm can reduce dose delivery by up to 30%. Treatment planning for HDR-IORT is greatly facilitated by creating a plan atlas of clinically anticipated tumor volumes.35 Plans incorporating optimized dwell times enhance efficiency and reduce risks of error. However, a plan atlas cannot anticipate the need to spare an adjacent normal tissue in certain patients, but customized lead wafers of 0.3 cm thickness can be used at the applicator–tissue interface.
KV-IORT: Physics and Technical Applications
The Zeiss Intrabeam has a miniature x-ray source at the end of a 10-cm-long, 3-mm-wide probe, where electrons are accelerated at a gold target in the probe tip, resulting in a nearly isotronic distribution of low-kV x-rays in the 30- to 50-kV range. Because these x-rays are of such low energy, no special shielding is required in a standard OR, which normally has adequate shielding for the intraoperative use of diagnostic radiology equipment. The Intrabeam is small, lightweight, designed with a floor stand, and can be easily accommodated in a standard OR. A dose rate of 2 Gy/min at 1 cm in water from the center of the target is possible with a 50-kV setting. However, the dose decreases in tissue as the inverse cube of the distance. It has an extended lifetime of over 10 years.
The Xoft S700 Axxent System is an electronic brachytherapy apparatus with a Wolfram target that operates at x-ray energies of 20 to 50 kV. As with the Intrabeam, the 50-kV energy is most commonly used. A miniature x-ray tube is located within a flexible, disposable sheath, which permits water cooling of the x-ray tube. The Xoft source is more adaptable in the OR compared to the Intrabeam source and has a higher depth–dose rate of 0.6 Gy/min at 3 cm in water. In addition, because of heavier filtration, the dose falls off less slowly in tissue than the Intrabeam source. However, the typical Xoft source lifetime is only 2.5 hours, and it has been used clinically for a much shorter time period (3 years), compared to more than a decade for Intrabeam.
A recent comprehensive review of these two KV-IORT systems is available.36 Both units are potentially suited for IORT treatment of breast cancer, either as a boost or as definitive treatment, although most of the current clinical data involve the use of the Intrabeam. Both manufacturers are expanding the clinical use of their respective low-kV IORT systems to superficial skin tumors and vaginal applications for endometrial cancers. The main applicators for the Intrabeam are spherical, with diameters ranging from 1.5 to 5 cm, and are composed of solid biodegradable material with a 2.8-mm central cavity where the source is placed. A homogeneous surface dose is generated with the addition of an aluminum flattening filter for applicators greater than 3.5 cm in diameter. The Xoft system uses inflatable balloons, similar to MammoSite (Hologic, Bedford, MA) for breast irradiation, with spherical or ellipsoidal balloon shapes. The balloon sizes vary from 3 to 6 cm for spherical balloons and 5 × 7 cm and 6 × 7 cm for ellipsoidal balloons. Both systems have a number of safety features to alert the user to the emission of x-rays and allow monitoring of the output dose. QA procedures involving pretreatment checks, monthly checks, and yearly checks were recently reviewed.36
Comparison of IORT Technical Applications
A comprehensive comparison of the technical applications of the three IORT approaches was recently published.37 The potential advantages of IOERT compared to HDR-IORT include better dose homogeneity, faster treatment times, requirement for less OR shielding, and, the ability to treat gross residual disease based on depth–dose characteristics. Potential disadvantages include surface dose of less than 90% with 6- to 9-MeV electrons (compensated with the use of bolus) and difficulty in treating anatomic areas such as the low pelvis, abdominal side walls, retropubic areas, subdiaphragmatic areas, thoracic side walls, and skull base. Low-kV IORT requires a small target (tumor) volume, where the depth in tissue at risk is 0.5 to 1.0 cm or less from the surface of the applicator.
CLINICAL RESULTS OF IORT WITH OR WITHOUT EBRT: AN OVERVIEW
A comprehensive review of the clinical experience of IORT ± EBRT (± chemotherapy) is beyond the scope of this chapter, and the reader is referred to a recently published textbook of IORT in which the clinical data by tumor site are presented in detail.38 In addition, some of the IORT clinical data are discussed in this textbook in chapters devoted to specific cancers and tumor sites. The general conclusions from these mostly nonrandomized studies in selected cancers will be discussed here, in addition to a brief overview of the limited clinical data from a few prospective, randomized trials. A general conclusion is that IORT, as a component of a multidisciplinary approach for typically locally advanced primary and recurrent cancers, is feasible and appears to improve local control, as well as overall survival in some cancers, with acceptable acute and late toxicities based on the normal tissue tolerance guidelines previously presented (Table 18.2). The clinical use of IORT in a larger number of institutions across the world over the last 10 to 15 years has been aided by improvements in IORT technology as described in the last section. The more widespread use and the promising nonrandomized, generally single-institution, clinical data, as well as data from a few prospective, randomized trials, will, it is hoped, facilitate the design and implementation of prospective, randomized trials in specific tumor sites in the next decade.
Colorectal Cancer
The clinical experience with the use of IOERT and HDR-IORT as part of a multidisciplinary approach to locally advanced colorectal cancer was recently summarized.39 More than 900 patients have been treated with IOERT and 59 patients with HDR-IORT, usually following preoperative combined-modality treatment with EBRT (45 to 54 Gy) and concomitant infusional 5-fluorouracil (5-FU). These data are derived from multiple, nonrandomized, single-institution studies in the United States and Europe. No randomized, prospective studies are available or ongoing. Most patients had clinical T4 or tethered T3 lesions in these IORT studies, making direct comparison to large prospective, randomized trials, such as the German Rectal Cancer Study, difficult, because, certainly, the clinical T4 lesions would not be included in the phase III trial. The 3- to 5-year local control rates for IORT-treated patients were, respectively, 85% to 90% for R0 patients and 50% to 75% for R1/R2 resections, with a 5% to 10% risk of grade 2 or 3 normal tissue toxicities to peripheral nerve or ureter. The 3- to 5-year disease-free survivals were in the 50% to 60% range for R0 patients but dropped to 25% to 35% for R2 patients. Thus, these data suggest some benefit to IORT in these clinically tethered T3 and T4 patients, but systemic relapse remains a major problem.
A similar general conclusion can be reached for the use of IORT in patients with recurrent colorectal cancer, based on a recent summary of the literature.40 For this patient group, many will have already received EBRT ± 5-FU-based chemotherapy at the time of presentation, making the use of further EBRT (± chemotherapy) somewhat limited. The largest experience with IOERT in this patient group is from the Mayo Clinic. In a group of 140 patients with prior EBRT, the subsequent use of 30-Gy preoperative EBRT followed by IOERT (10 to 20 Gy based on extent of resection) resulted in a 3-year local control rate of 70% and overall survival rate of 30%. Again, no randomized, prospective data are available to more accurately assess the role of IORT in this patient group, but the single-institution data are encouraging.40
Gastric Cancer
The clinical data regarding the integration of IORT as a component of combined-modality treatment of resectable gastric cancer were recently summarized in detail.41 Three randomized prospective trials of IOERT have been reported. In 1988, M. Abe from Kyoto University, Japan, reported a trial in which more than 200 patients with stage I-IV stomach cancer (using the Japanese Surgical Staging System) were randomized to surgery alone versus surgery + IOERT (28 to 35 Gy).42 A non–statistically significant trend toward improved 5-year overall survival was seen in stage II-IV patients. However, two smaller phase 3 studies did not show a survival benefit. At the National Cancer Institute, 40 stage III and IV (American Joint Committee on Cancer) patients were randomized to surgery + IORT (20 Gy) versus surgery + EBRT (50 Gy), whereas at the University of Freiburg (Freiburg, Germany), 115 patients were randomized to surgery versus surgery + IORT (28 Gy).43,44 Data from other single-institution, nonrandomized studies suggest a benefit to IORT or IORT + EBRT compared to surgery alone (summarized by Martinez-Monge et al.41). The present standard of care for locally advanced (node positive and/or margin positive) gastric cancer includes postoperative chemoradiation or perioperative chemotherapy alone, based on recent phase 3 trial experience.45,46 Thus, any future trial testing the integration of IORT in locally advanced gastric cancer must include these approaches.
Pancreatic Cancer
The results of many single-institution, nonrandomized clinical trials in patients with pancreas cancer were recently summarized.47 For patients found to have localized but technically unresectable disease, the use of IORT ± EBRT ± chemotherapy is associated with better long-term epigastric pain control but with no effect on median or overall survival. In patients with resectable pancreatic cancer, the combination of preoperative EBRT (± chemotherapy) followed by IORT appears to increase local control and overall survival modestly. Any future trials of IORT in pancreatic cancer will require the integration of more effective systemic therapy.
Sarcomas
IORT has played a role in the management of adult soft tissue sarcomas arising in the retroperitoneum, trunk, and extremities as a component of combined-modality treatment. IORT has also been used in the treatment of some primary bone sarcomas in adolescents. Both IOERT and HDR-IORT techniques have been used.
Retroperitoneal soft tissue sarcomas, although rare, are an appropriate tumor for using IORT because these tumors are typically large (>10 to 15 cm) and locally invasive, making wide excisions with negative margins very difficult to achieve. A comprehensive review of the IORT studies in retroperitoneal sarcomas was recently published.48 In the only randomized, prospective trial, a small group of 35 patients with technically resectable retroperitoneal sarcomas were randomized to receive postoperative EBRT (50 to 55 Gy; control group; 20 patients) or IOERT (20 Gy to multiple abutting fields) plus reduced-dose postoperative EBRT (30 to 35 Gy; study group; 15 patients).21 With a minimum follow-up of 5 years, in-field local recurrences occurred in 20% of the study group and in 80% of the controls (p < .001). However, there were no differences in overall survival or risk of distant metastases, which were correlated primarily with pathologic stage. Differences in the type of acute and late normal tissue complications were found with significantly increased gastrointestinal toxicities in the control group and significantly increased peripheral nerve toxicities in the study group. Multiple nonrandomized, single-institution studies support an improved local control rate with less peripheral nerve toxicities using IOERT doses of 15 Gy or less.48 Two institutions have published their experience using combinations of EBRT and HDR-IORT for primary or locally recurrent retroperitoneal sarcomas. A prospective, nonrandomized trial at Memorial Sloan-Kettering Cancer Center (MSKCC) included 32 patients (12 with primary disease and 20 with recurrent disease) who each received 14 Gy at 0.5 cm deep to the resected tumor bed using HDR-IORT. The MSKCC group reported a 5-year local control rate of 74% and 54% and a 5-year overall survival rate of 55% and 30%, respectively, in the primary and recurrent tumor patient groups.49 A second series of 46 patients with primary or recurrent retroperitoneal sarcomas from the Curie Cancer Center in France received 20-Gy HDR-IORT following a gross total resection (30 patients with R0 resection and 16 patients with R1 resection). These patients experienced actuarial 5-year overall survival and local recurrence survival rates of 55% and 51%, respectively.50
The IORT literature for extremity and truncal soft tissue sarcomas was recently summarized.51 Although local recurrence risks using surgery and EBRT (either preoperative or postoperative) are low (5% to 8%) with R0 resections, patients with positive margins or gross residual disease require higher EBRT doses, with an increased risk of acute and late normal tissue toxicities. For these patients, IOERT or HDR-IORT treatments have been used as a treatment boost while maintaining the EBRT dose at 45 to 50 Gy. In general, local control is excellent (≥85%), and normal tissue complications and functional outcomes are acceptable.52
The more limited experience with IORT in bone sarcomas was also recently reviewed.53 Typically, IOERT has been used for marginally resected Ewing’s sarcomas and osteosarcomas in adolescents at centers in Germany, Spain, and Japan. Although local control is excellent in these high-risk patients, the risks of late normal tissue complications, including IORT-induced second cancers, are of concern, and long-term follow-up is needed before an endorsement of the role of IORT in these patient groups can be made.
Breast Cancer
IORT is playing a major role in the curative treatment of early-stage breast cancer throughout the world, as recently reviewed.54 Based on several prior single-institution, nonrandomized studies,54 two large phase III studies have been designed for patients with early-stage disease to test the concept of IORT as full-dose, single-dose partial breast irradiation treatment compared to standard, conventionally fractionated whole-breast radiation therapy (WBRT). In addition, the concept of using IORT as a boost followed by WBRT for patients with adverse pathologic features is being evaluated by the European Group of the International Society of Intraoperative Radiotherapy (ISIORT Europe). A brief description of the two large phase III studies of definitive IORT and the ongoing single arm IORT boost study is given later. A more detailed discussion of these trials is provided in the chapter of this book on breast cancer.
The Targeted Intraoperative Radiotherapy Trial enrolled and randomized more than 2,200 early-stage (T1, T2; N0) breast cancer patients with invasive ductal carcinoma without evidence of lobular carcinoma and older than 45 years of age to single-fraction, orthovoltage IORT to 20 Gy (surface dose) or conventionally fractionated WBRT to 50 Gy.55 Only in the case of specific risk factors was the IORT complemented with WBRT. The first interim analysis, published in June 2010, with a median follow-up of 2 years, reported a similar local recurrence risk at 4 years (1.2% in IORT arm vs. 0.95% in the WBRT arm) and similar normal tissue complication rates (3.3% with IORT vs. 3.9% with WBRT).55 Although encouraging, longer follow-up is needed before definitive conclusions may be drawn.
The Electron Intraoperative Treatment Trial compared IOERT to 24 Gy to the 90% isodose line to the use of WBRT. The Novac 7 and LIAC mobile electron machines were used.56 This trial has reached its anticipated accrual of approximately 650 patients on each arm, and the first interim analysis will soon be published. However, a single-arm study of IORT to 21 Gy was reported by Veronesi et al.57 from Milan, Italy, on 574 early-stage breast cancer patients. In this study, a 1.05% rate of in-breast recurrence was found, with a median follow-up of 20 months. Again, these preliminary data are encouraging for the use of IORT as a single-dose, partial-breast irradiation treatment.
Since 2005, the ISIORT Europe Group of six institutions has enrolled more than 1,200 patients to a single-arm study of an IOERT boost to 10 Gy to the 90% isodose line prior to WBRT to 50 Gy.58 At least 60% of patients had at least one adverse prognostic factor for developing a local recurrence, including a tumor size of greater than 2 cm, high-grade histology, young age (<45 years), and/or positive lymph nodes. With a median follow-up of 6 years, a 1.2% local recurrence rate was found. Thus, IORT may also be used as an effective boost treatment combined with WBRT for higher-risk patients.
Other Cancer Types
The clinical data regarding the use of IORT for lung cancers, head and neck cancers, CNS malignancies, and pediatric cancers were recently reviewed in detail,38 and the reader is referred to chapters in this textbook on specific tumor sites for additional information. No prospective, randomized trials of IORT for these cancers are available or in development.
REFERENCES
1. Hall EJ, Marchese M, Hei TK, et al. Radiation response characteristics of human cells in vitro. Radiat Res 1988;114(3):415–424.
2. Thames HD, Suit HD. Tumor radioresponsiveness versus fractionation sensitivity. Int J Radiat Oncol Biol Phys 1986;12(4):687–691.
3. Hu K, Yom S, Kaplan M, et al. Head and neck cancer. In: Gunderson L, Willett C, Calvo F, et al., eds. Intraoperative irradiation: techniques and results, 2nd ed. Totowa, NJ: Humana Press; 2011:163–168.
4. Kinsella TJ, DeLuca AM, Barnes M, et al. Threshold dose for peripheral neuropathy following intraoperative radiotherapy (IORT) in a large animal model. Int J Radiat Oncol Biol Phys 1991;20(4):697–701.
5. Kinsella TJ, Sindelar WF, DeLuca AM, et al. Tolerance of peripheral nerve to intraoperative radiotherapy (IORT): clinical and experimental studies. Int J Radiat Oncol Biol Phys 1985;11(9):1579–1585.
6. LeCouteur RA, Gillette EL, Powers BE, et al. Peripheral neuropathies following experimental intraoperative radiation therapy (IORT). Int J Radiat Oncol Biol Phys 1989;17(3):583–590.
7. Barnes M, Duray P, DeLuca A, et al. Tumor induction following intraoperative radiotherapy: late results of the National Cancer Institute canine trials. Int J Radiat Oncol Biol Phys 1990;19(3):651–660.
8. Sindelar WF, Kinsella TJ. Normal tissue tolerance to intraoperative radiotherapy. Surg Oncol Clin N Am 2003;12(4):925–942.
9. Vujaskovic Z, Willett CG, Tepper J, et al. Normal tissue tolerance to IOERT, EBRT, or both: animal and clinical studies. In: Gunderson L, Willett CG, Calvo F, et al., eds. Intraoperative irradiation: techniques and results, 2nd ed. Totowa, NJ: Humana Press, 2011:119–138.
10. Sindelar WF, Tepper JE, Kinsella TJ, et al. Late effects of intraoperative radiation therapy on retroperitoneal tissues, intestine, and bile duct in a large animal model. Int J Radiat Oncol Biol Phys 1994;29(4):781–788.
11. Gillette EL, Powers BE, McChesney SL, et al.. Response of aorta and branch arteries to experimental intraoperative irradiation. Int J Radiat Oncol Biol Phys 1989;17(6):1247–1255.
12. Johnstone PA, Sprague M, DeLuca AM, et al. Effects of intraoperative radiotherapy on vascular grafts in a canine model. Int J Radiat Oncol Biol Phys 1994; 29(5):1015–1025.
13. Tepper JE, Sindelar W, Travis EL, et al.. Tolerance of canine anastomoses to intraoperative radiation therapy. Int J Radiat Oncol Biol Phys 1983;9(7):987–992.
14. Sindelar WF, Tepper J, Travis EL. Tolerance of bile duct to intraoperative irradiation. Surgery 1982;92(3):533–540.
15. Gillette EL, Gillette S, Powers BE. Studies at Colorado State University of normal tissue tolerance of beagles to IOERT, EBRT or a combination. In: Gunderson L, Willett CG, Calvo F, et al., eds. Intraoperative irradiation: techniques and results, 1st ed. Totowa, NJ: Humana Press; 1999:147–163.
16. DeLuca AM, Johnstone PA, Ollayos CW, et al. Tolerance of the bladder to intraoperative radiation in a canine model: a five-year follow-up. Int J Radiat Oncol Biol Phys 1994;30(2):339–345.
17. Kinsella TJ, Sindelar WF, DeLuca AM, et al. Tolerance of the canine bladder to intraoperative radiation therapy: an experimental study. Int J Radiat Oncol Biol Phys 1988;14(5):939–946.
18. Barnes M, Pass H, DeLuca A, et al. Response of the mediastinal and thoracic viscera of the dog to intraoperative radiation therapy (IORT). Int J Radiat Oncol Biol Phys 1987;13(3):371–378.
19. Tochner ZA, Pass HI, Sindelar WF, et al. Long term tolerance of thoracic organs to intraoperative radiotherapy. Int J Radiat Oncol Biol Phys 1992;22(1):65–69.
20. Pass HI, Sindelar WF, Kinsella TJ, et al. Delivery of intraoperative radiation therapy after pneumonectomy: experimental observations and early clinical results. Ann Thorac Surg 1987;44(1):14–20.
21. Sindelar WF, Kinsella TJ, Chen PW, et al. Intraoperative radiotherapy in retroperitoneal sarcomas. Final results of a prospective, randomized, clinical trial. Arch Surg 1993;128(4):402–410.
22. Johnstone PA, DeLuca AM, Bacher JD, et al. Clinical toxicity of peripheral nerve to intraoperative radiotherapy in a canine model. Int J Radiat Oncol Biol Phys 1995;32(4):1031–1034.
23. Vujaskovic Z, Gillette SM, Powers BE, et al. Intraoperative radiation (IORT) injury to sciatic nerve in a large animal model. Radiother Oncol 1994;30(2):133–139.
24. Vujaskovic Z, Gillette SM, Powers BE, et al. Ultrastructural morphometric analysis of peripheral nerves after intraoperative irradiation. Int J Radiat Biol 1995;68(1):71–76.
25. Gillette SM, Gillette EL, Powers BE, et al. Radiation-induced osteosarcoma in dogs after external beam or intraoperative radiation therapy. Cancer Res 1990;50(1):54–57.
26. Johnstone PA, Laskin WB, DeLuca AM, et al. Tumors in dogs exposed to experimental intraoperative radiotherapy. Int J Radiat Oncol Biol Phys 1996;34(4):853–857.
27. Beddar AS, Biggs PJ, Chang S, et al. Intraoperative radiation therapy using mobile electron linear accelerators: report of AAPM Radiation Therapy Committee Task Group No. 72. Med Phys 2006;33(5):1476–1489.
28. Palta JR, Biggs PJ, Hazle JD, et al. Intraoperative electron beam radiation therapy: technique, dosimetry, and dose specification: report of task force 48 of the Radiation Therapy Committee, American Association of Physicists in Medicine. Int J Radiat Oncol Biol Phys 1995;33(3):725–746.
29. Ciocca M, Orecchia R, Garibaldi C, et al. In vivo dosimetry using radiochromic films during intraoperative electron beam radiation therapy in early-stage breast cancer. Radiother Oncol 2003;69(3):285–289.
30. Consorti R, Petrucci A, Fortunato F, et al. In vivo dosimetry with MOSFETs: dosimetric characterization and first clinical results in intraoperative radiotherapy. Int J Radiat Oncol Biol Phys 2005;63(3):952–960.
31. Nath R, Anderson LL, Luxton G, et al. Dosimetry of interstitial brachytherapy sources: recommendations of the AAPM Radiation Therapy Committee Task Group No. 43. American Association of Physicists in Medicine. Med Phys 1995;22(2):209–234.
32. Rivard MJ, Butler WM, DeWerd LA, et al. Supplement to the 2004 update of the AAPM Task Group No. 43 Report. Med Phys 2007;34(6):2187–2205.
33. Furhang EE, Sillanpaa JK, Hu KS, et al. HDR-IORT: physics and techniques. In: Gunderson L, Willett CG, Calvo F, et al., eds. Intraoperative irradiation: techniques and results, 2nd ed. Totowa, NJ: Humana Press, 2011:73–84.
34. Kutcher GJ, Coia L, Gillin M, et al. Comprehensive QA for radiation oncology: report of AAPM Radiation Therapy Committee Task Group 40. Med Phys 1994;21(4):581–618.
35. Anderson LL, Hoffman MR, Harrington PJ, et al. Atlas generation for intraoperative high dose rate brachytherapy. J Brachyther Int 1997;13:333–340.
36. Kraus-Tiefenbacher U, Biggs PJ, Vaidya J, et al. Electronic brachytherapy/low KV-IORT: physics and techniques. In: Gunderson L, Willett CG, Calvo F, et al., eds. Intraoperative irradiation: techniques and results, 2nd ed. Totowa, NJ: Humana Press, 2011:85–98.
37. Nag S, Willett CG, Gunderson L, et al. IORT with electron-beam, high-dose-rate brachytherapy or low-KV/electronic brachytherapy: methodological comparisons. In: Gunderson L, Willett CG, Calvo F, et al., eds. Intraoperative irradiation: techniques and results, 2nd ed. Totowa, NJ: Humana Press, 2011:99–115.
38. Gunderson L, Willett CG, Calvo F, et al., eds. Intraoperative irradiation: techniques and results, 2nd ed. Totowa, NJ: Humana Press, 2011: Part IV, 139–518.
39. Nils D, Arvold ND, Hong TS, et al. Primary colorectal cancer. In: Gunderson L, Willett CG, Calvo F, et al., eds. Intraoperative irradiation: techniques and results, 2nd ed. Totowa, NJ: Humana Press, 2011:297–322.
40. Haddock MG, Nelson H, Valentini V, et al. Recurrent colorectal cancer. In: Gunderson L, Willett CG, Calvo F, et al., eds. Intraoperative irradiation: techniques and results, 2nd ed. Totowa, NJ: Humana Press, 2011:323–351.
41. Martinez-Monge R, Gaztanaga M, Alvarez-Cienfuegos J, et al. Gastric cancer. In: Gunderson L, Willett CG, Calvo F, et al., eds. Intraoperative irradiation: techniques and results, 2nd ed. Totowa, NJ: Humana Press, 2011:223–248.
42. Abe M, Takahashi M, Ono K, et al. Japan gastric trials in intraoperative radiation therapy. Int J Radiat Oncol Biol Phys 1988;15(6):1431–1433.
43. Kramling HJ, Willich N, Cramer C, et al. Early results of IORT in the treatment of gastric cancer. Front Radiat Ther Oncol 1997;31:157–160.
44. Sindelar WF, Kinsella TJ, Tepper JE, et al. Randomized trial of intraoperative radiotherapy in carcinoma of the stomach. Am J Surg 1993;165(1):178–186; discussion 186–177.
45. Cunningham D, Allum WH, Stenning SP, et al. Perioperative chemotherapy versus surgery alone for resectable gastroesophageal cancer. N Engl J Med 2006; 355(1):11–20.
46. Macdonald JS, Smalley SR, Benedetti J, et al. Chemoradiotherapy after surgery compared with surgery alone for adenocarcinoma of the stomach or gastroesophageal junction. N Engl J Med 2001;345(10):725–730.
47. Miller RC, Valentini V, Moss A, et al. Pancreas cancer. In: Gunderson L, Willett CG, Calvo F, et al., eds. Intraoperative irradiation: techniques and results, 2nd ed. Totowa, NJ: Humana Press, 2011:249–271.
48. Czito B, Donohue J, Willett CG, et al. Retroperitoneal sarcomas. In: Gunderson LL, Willett CG, Calvo F, et al., eds. Intraoperative irradiation: techniques and results, 2nd ed. Totowa, NJ: Humana Press, 2011:353–386.
49. Alektiar KM, Hu K, Anderson L, et al. High-dose-rate intraoperative radiation therapy (HDR-IORT) for retroperitoneal sarcomas. Int J Radiat Oncol Biol Phys 1 2000;47(1):157–163.
50. Dziewirski W, Rutkowski P, Nowecki ZI, et al. Surgery combined with intraoperative brachytherapy in the treatment of retroperitoneal sarcomas. Ann Surg Oncol 2006;13(2):245–252.
51. Petersen IA, Krempien R, Beauchamp C, et al. Extremity and trunk soft-tissue sarcomas. In: Gunderson LL, Willett CG, Calvo F, et al., eds. Intraoperative irradiation: techniques and results, 2nd ed. Totowa, NJ: Humana Press, 2011:387–405.
52. Kunos C, Colussi V, Getty P, et al. Intraoperative electron radiotherapy for extremity sarcomas does not increase acute or late morbidity. Clin Orthop Relat Res 2006:247–252.
53. Calvo F, Sierrasesumaga L, Patino A, et al. Bone sarcomas. In: Gunderson LL, Willett CG, Calvo F, et al., eds. Intraoperative irradiation: techniques and results, 2nd ed. Totowa, NJ: Humana Press, 2011:407–429.
54. Sedlmayer F, DuBois J-B, Reitsamer R, et al. Breast cancer. In: Gunderson LL, Willett CG, Calvo F, et al., eds. Intraoperative irradiation: techniques and results, 2nd ed. Totowa, NJ: Humana Press, 2011:189–200.
55. Vaidya JS, Joseph DJ, Tobias JS, et al. Targeted intraoperative radiotherapy versus whole breast radiotherapy for breast cancer (TARGIT-A trial): an international, prospective, randomised, non-inferiority phase 3 trial. Lancet 2010;376(9735):91–102.
56. Intra M, Leonardi C, Luini A, et al. Full-dose intraoperative radiotherapy with electrons in breast surgery: broadening the indications. Arch Surg 2005;140(10): 936–939.
57. Veronesi U, Orecchia R, Luini A, et al. Full-dose intraoperative radiotherapy with electrons during breast-conserving surgery: experience with 590 cases. Ann Surg 2005;242(1):101–106.
58. Sedlmayer F, Fastner G, Merz F, et al. IORT with electrons as boost strategy during breast conserving therapy in limited stage breast cancer: results of an ISIORT pooled analysis. Strahlenther Onkol 2007;183(Spec No 2):32–34.
< div class='tao-gold-member'>
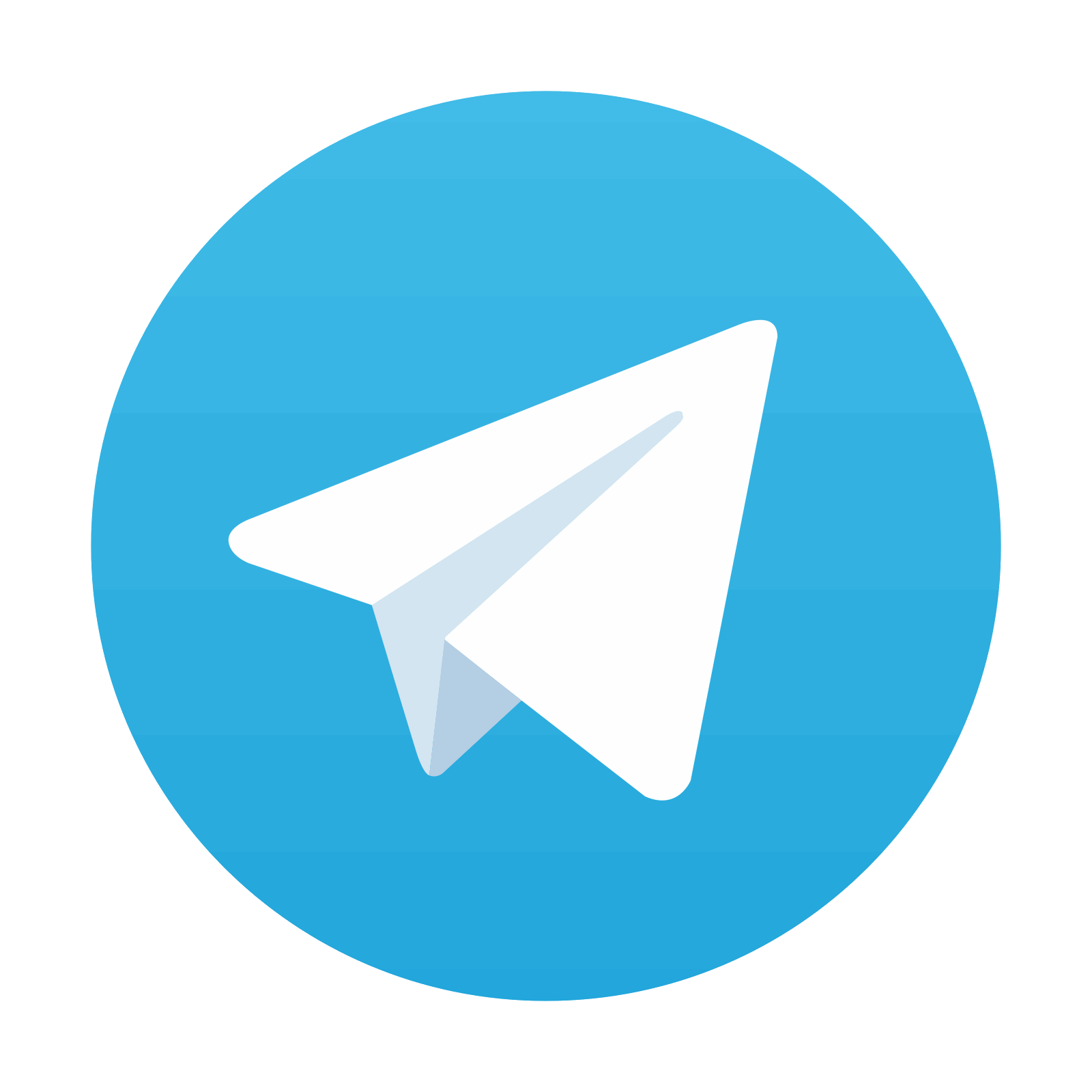