Chapter 16 Intraoperative Irradiation
History
The use of IORT was first employed almost 100 years ago.1 The contemporary approach to IORT was initiated in the 1960s by Abe and colleagues in Japan. These investigators advocated resection (where possible) with large single-dose radiation therapy (25 to 40 Gy using cobalt-60).2 By the mid-to-late 1970s, many institutions in the United States (e.g., Howard University, Massachusetts General Hospital, the Mayo Clinic, and the National Cancer Institute [NCI]) had adopted IORT as a treatment approach using linear accelerator–based electron treatment in the operating room. Presently there are about 90 centers in at least 16 countries worldwide with active IORT programs.
Biology
When EBRT is fractionated there is a preferential therapeutic advantage for normal tissues relative to tumor as defined by the “4Rs” of classical radiobiology (normal tissue repair, tumor reoxygenation, tumor redistribution, and normal tissue repopulation). With a single large fraction of radiation therapy, these advantages are lost. In addition, large doses per fraction may result in increased risk of late effects. There is evidence that small vessel injury caused by large doses per fraction may contribute to late effects, and ischemic complications are dose dependent.3 Furthermore, tumor response to single and fractionated radiation therapy depends on the percentage of hypoxic cells within a tumor. This differential sensitivity between hypoxic and well-oxygenated cells increases with increasing dose.
Using alpha/beta (α/β) calculations, biologically equivalent doses to a fractionated EBRT course using 2 Gy per fraction for varying IORT doses have been estimated (Table 16-1). There are disadvantages from a late effects standpoint with IORT, but many of these disadvantages are mitigated by exclusion of nontarget tissues from the radiation field by direct inspection, mobilization, and shielding.4 When combined with EBRT and resection, IORT doses of 10 to 20 Gy provide local control for most solid tumors, especially in the setting of microscopic residual tumor tissue. When combined with EBRT and surgery there is little reason to exceed IORT doses of 10 to 20 Gy. Late normal tissue complications are often the limiting sequelae of IORT administration, and careful planning and administration with techniques designed to reduce the dose to nontarget tissues are of paramount importance.
Local Control: An Important Endpoint
Pancreatic Cancer
EBRT with 5-FU–based chemotherapy employed in the treatment of unresectable pancreatic cancer results in a doubling of median survival compared with surgical bypass/stenting alone (3 to 6 months vs. 9 to 13 months) and an increase in 2-year survival from 0% to 5% to 10% to 20%.5 Unfortunately, these techniques result in poor local control rates. Series from the Mayo Clinic, Thomas Jefferson University Hospital, and other institutions report local failure rates exceeding 70% to 80%6,7,8,9,10 (Table 16-2).
Retroperitoneal Sarcoma
When surgery is used as the primary treatment modality for retroperitoneal sarcomas, local failure has been reported to range from 40% to 90%. Despite the addition of EBRT to surgery, local failure rates are 40% to 80%. This is in contrast to extremity sarcomas in which local control rates approach 90%. Because of the limited tolerance of surrounding normal tissue (small intestine, stomach, liver, kidney, spinal cord), EBRT doses are limited. A randomized NCI trial evaluating IORT in retroperitoneal sarcomas demonstrated that patients receiving IORT + EBRT experienced significantly improved local control and less small bowel toxicity versus patients treated with EBRT alone (80% in-field relapse; see later discussion).11
Colon and Rectal Cancer
In patients with locally advanced (T4) or locally recurrent colon and rectal cancer, local control is difficult to achieve, despite multimodality therapy. Studies from Princess Margaret Hospital and the Mayo Clinic report local failure rates of 90% or greater in evaluable patients treated with EBRT with or without systemic therapy.12,13 In radiation-naive patients, the optimal approach in patients with locally advanced disease is preoperative EBRT combined with 5-FU–based chemotherapy followed by resection. Despite this, local recurrence occurs in 30% to 70% of patients.14
Cervical Cancer
For patients with cervical cancer, para-aortic nodal metastases are common. Despite the presence of these “distant” metastases, 15% to 20% of patients are cured by radical radiotherapy techniques, employing EBRT doses of 55 to 60 Gy. However, high complication rates have been reported with these doses and techniques.15,16 As in rectal cancer, patients with recurrent cervical cancer in the pelvis or para-aortic region have a poor long-term prognosis with 5-year overall survival (OS) rates ranging from 5% to 30%. These patients have often been previously irradiated, and re-treatment with meaningful doses of EBRT is usually not feasible given normal tissue tolerance. When patients have para-aortic or locally recurrent disease, administration of IORT is a feasible method to escalate dose and enhance local control.
Local Control: Radiation Dose, Complications, Shrinking-Field Techniques, Distant Metastases
Influence of Dose
In both animal and human models the probability of local control of a tumor by radiation is proportional to the total dose administered. The dose of radiation to control a tumor locally depends on several factors, including tumor type, clonogen number, and tumor microenvironment. Thus a given radiation dose may be able to control a small tumor with high probability and acceptable morbidity but that same dose may be insufficient against disease of larger volume. Clinical experience has generated a body of data correlating local control by tumor type and radiation dose. In vivo data for a variety of irradiated human tumors of varying sizes and types are summarized in Figure 16-1.
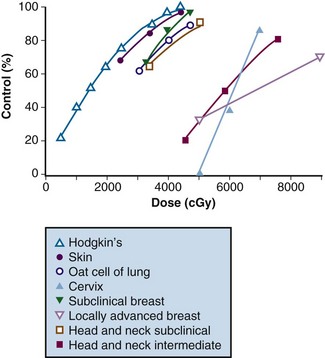
Figure 16-1 Local control versus dose of irradiation.
From Gunderson LL, Tepper JE, Biggs DJ, et al: Intraoperative ± external beam irradiation. Curr Probl Cancer 7:8, 1983.
The studies of Fletcher examined local control probability as a function of radiation dose for patients undergoing treatment for breast carcinoma and squamous cell carcinoma of the upper aerodigestive tract (see web-only Table 16-1 on the Expert Consult website). For breast cancer patients, control of subclinical disease was 60% to 70% with 30 to 35 Gy, 85% with 40 Gy, and 95% with 45 to 50 Gy. For larger/palpable tumors, EBRT doses of 46 Gy, 59 Gy, and 76 to 90 Gy result in a local control probability of 20%, 35% to 50%, and 70% to 80%, respectively.17,18,19,20 These data suggest that marked improvements in local control can be achieved by escalating radiation doses.
WEB-ONLY TABLE 16-1 Tumor Control Probability Correlated with Irradiation Dose and Volume of Cancer
Dose (Gy) | Tumor Control Probability |
---|---|
Squamous Cell Carcinoma: Upper Aerodigestive Tract | |
50* | >90% subclinical |
~60% T1 lesions of nasopharynx | |
~50% 1- to 3-cm neck nodes | |
60* | ~90% T1 lesions of pharynx and larynx |
~50% T3 and T4 lesions of tonsillar fossa | |
~90% 1- to 3-cm neck nodes | |
~70% 3- to 5-cm neck nodes | |
70* | ~90% T2 lesions of tonsillar fossa and supraglottic larynx |
~80% T3 and T4 lesions of tonsillar fossa | |
Adenocarcinoma of the Breast | |
50* | >90% subclinical |
60* | 90% clinically positive axillary nodes, 2.5-3.0 cm |
70* | 65% 2-3 cm primary |
70-80 (8-9 wk) | 30% >5 cm primary |
80-90 (8-10 wk) | 56% >5 cm primary |
80-100 (10-12 wk) | 75% 5-15 cm primary |
* 10 Gy in five fractions each week.
Modified from Fletcher GH, Shukovsky LJ: The interplay of radiocurability and tolerance in the irradiation of human cancers. J Radiol Electrol 56:383-400 1975.
Dose Versus Complications
The chief limitation of EBRT to control macroscopic disease in the abdomen and pelvis is normal tissue tolerance. Normal organs such as stomach, small bowel, and kidney have tolerance levels well below the radiation doses required to eradicate most abdominal and pelvic malignancies. Exceeding these EBRT doses results in a prohibitive risk of late normal tissue damage (see web-only Table 16-2 on the Expert Consult website). Because of this, “conventional” tolerable doses of EBRT from 45 to 55 Gy using 1.8 to 2.0 Gy per fraction are not curative in most abdominal and pelvic malignancies, with resultant local persistence/local recurrence of disease common in patients treated with radiation therapy alone. This often results in tumor-related morbidity and mortality such as bowel obstruction and perforation, ureteral obstruction, and neuropathy.
Although local control is enhanced with increasing doses of radiation, tumor dose-response curves with EBRT closely resemble normal tissue complication curves. Therefore, efforts to improve local control through escalating EBRT doses may also result in treatment-related complications (Fig. 16-2). In an R1 (microscopic residual) resection, EBRT doses of 60 Gy or higher using conventional fractionation schemes are necessary to achieve a high probability of local control. In an R2 (gross residual) resection, even higher doses are usually required. Such doses exceed normal tissue tolerance (see web-only Table 16-2 on the Expert Consult website).
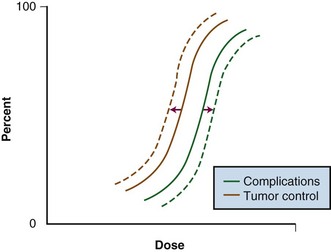
Figure 16-2 Radiation dose versus incidence of tumor control or complications.
From Gunderson LL, Tepper JE, Biggs DJ, et al: Intraoperative ± external beam irradiation. Curr Probl Cancer 7:8, 1983.
Because of the risks associated with dose escalation beyond normal tissue tolerance, an attractive alternative in patients with locally advanced malignancies is to deliver moderate doses of EBRT (i.e., at or below accepted tolerance of surrounding normal tissue). A typical course would range from 45 to 50 Gy at 1.8 to 2.0 Gy per fraction, followed by surgical exploration. After resection, IORT would be performed, avoiding or minimizing irradiation of surrounding organs by shielding or mobilization. With this approach, an increase in local control with decreased risk of normal tissue complications (relative to an EBRT-only approach) can be achieved (see Fig. 16-2: local control curve shifts to left with IORT; complication curve shifts to right with increasing EBRT doses).
Development of Distant Metastases
Preclinical data suggest that the incidence of distant metastases is related to both tumor size as well as the development of locally recurrent disease in multiple spontaneous tumor systems.21–23 In fibrosarcoma and squamous cell carcinoma cell lines in rodent models, Ramsay and associates21 reported increased rates of distant metastases in tumors measuring 6 versus 12 mm, as well as primary versus recurrent tumors. Additionally, Suit and colleagues23 showed that in mouse mammary tumors treated with single-dose irradiation, increasing rates of local failure were associated with increasing rates of distant metastases. Specifically, the incidence of metastatic disease was 31% (16 of 52) of mice with local control, 50% (9 of 18) in those with local relapse salvaged by resection, and 80% (12 of 15) in mice with local relapse in whom salvage was not attempted. Similar high rates of metastases associated with local failure have been observed in human malignancies, including cervical,24 prostate,25 head and neck,26 and breast27 cancers. These and other data suggest that metastases may arise from locally recurrent disease.
Patient Selection and Evaluation
Sequencing and Dose: EBRT, IORT
Sequencing with Surgery
The role of preoperative versus postoperative therapy has been evaluated in rectal cancer. A large German randomized trial demonstrated that patients undergoing neoadjuvant irradiation and chemotherapy experienced significantly improved local control and less toxicity than patients receiving postoperative irradiation and chemotherapy.30
Radiation Dose and Technique
The biologic effectiveness of single-dose IORT in early responding tissues (tumor) relative to an equivalent total dose of fractionated EBRT has been estimated to be 1.5 to 2.5 times the IORT dose delivered4,31,32 (see Table 16-1). Therefore the effective tumor doses (when “normalized” to fractionated EBRT doses) of IORT treatment added to 45 to 50 Gy given by EBRT are as follows: 10-Gy IORT dose, 60 to 80 Gy; 15-Gy IORT dose, 75 to 87.5 Gy; and 20 Gy IORT dose, 85 to 100 Gy. These figures are not intended to be exact but represent educated estimates.
A discussion of the technical aspects of IORT administration is beyond the scope of this chapter. In brief, the implementation of IORT-based treatment is a multidisciplinary effort including one or multiple surgeons, radiation oncologist, anesthesiologist, operating room nurse, radiation physicist/dosimetrist, and therapist. In its broadest sense, IORT may be administered with either electrons (IOERT) or high-dose-rate photon afterloading techniques (HDR-IORT). Each method has potential advantages and disadvantages (see later and web-only Tables 16-6 and 16-7).
Dose-Limiting Structures and Tolerance
The development of normal tissue late effects increases with increasing radiation dose as well as dose delivered per fraction. Therefore, the incidence of late normal tissue effects in patients receiving IORT plus EBRT would be higher than those receiving EBRT alone. However, in this context the severe morbidity and mortality associated with locally recurrent tumor is often overlooked. As an example, when EBRT alone is used as the primary treatment modality for locally advanced rectal cancer, more than 90% of patients experience local persistence or local recurrence of disease with associated symptomatology. These symptoms include severe pelvic pain and neuropathy, which are difficult to manage clinically; the vast majority of patients experience disease-related death within 2 to 3 years. An argument can be made that the tumor-related morbidity/mortality approaches 100% in these patients.33
IORT tolerance for intact or surgically manipulated organs or structures in animals (primarily canines) is seen in Table 16-3. Much of this information has been derived from studies from the NCI34,35,36–38,39 and Colorado State University.40–42
Ureter
Clinical studies of the effect of IOERT on the ureters of human cancer patients have been undertaken at the Mayo Clinic.43 Doses of 10 Gy administered intraoperatively resulted in a 50% incidence of ureteral obstruction, increasing to 70% with doses from 15 to 25 Gy. This high complication rate relative to canine models may be due to age-related factors, surgical manipulation, EBRT, and/or tumor bed effects.
In an update of this experience, Mayo Clinic investigators reported on 146 patients with locally advanced malignancies receiving IORT to one or both ureters with doses between 7.5 and 30 Gy.44 They reported the risk of obstruction after IOERT is significant and increases with time and IORT dose. However, obstruction risk for ureters not receiving IOERT was also high, which suggests an underlying risk of ureteral injury from other causes (EBRT, surgical manipulation of ureters). (A more detailed discussion of ureteral tolerance with IORT can be found on the Expert Consult website.)
Peripheral Nerve
Peripheral nerve tissue is the principal dose-limiting normal tissue for IORT in the pelvis and retroperitoneum. Data regarding peripheral nerve tolerance and neuropathy comes from canine models as well as clinical analyses from patients treated intraoperatively.* Peripheral nerve tissue is often situated adjacent to or directly involved by tumor in the abdomen and pelvis. Because of this, the relative surgical “immobility” of the peripheral nerve, and the inability to shield the nerve from the IORT field, nerve tissue will often receive full-dose EBRT and IORT.
The mechanism of neuropathy after IORT is poorly understood. Peripheral nerve tolerance depends on the volume of nerve irradiated and total dose delivered. In animal models, histomorphologic findings after IORT have demonstrated decreased central nerve fiber density, particularly in large nerve fibers receiving more than 20 Gy. Electron microscopy analysis has demonstrated increased microtubule density and neurofilament accumulation within axons without associated myelin changes, suggesting possible hypoxic injury related to vascular changes.54
A Spanish study evaluated 45 patients with primary or locally recurrent extremity soft tissue sarcoma undergoing resection with IOERT (10 to 20 Gy).55 Nine patients received IOERT alone secondary to prior EBRT or patient refusal. Five patients developed neurotoxicity at a median of 13 months; 4 of the 5 showed objective weakness and/or sensory loss. Most patients developing neuropathy received IOERT doses greater than 15 Gy.
An analysis from the Mayo Clinic evaluated peripheral nerve tolerance in 51 patients undergoing IOERT for primary or recurrent pelvic malignancies.43 Patients received EBRT (median dose 50.4 Gy), maximal resection where possible, and IOERT boost from 10 to 25 Gy using 9- to 18-MeV electrons. Sixteen (32%) of patients experienced grade I to III peripheral neuropathy as manifested by pelvic/extremity pain, leg weakness, numbness, or tingling. Pain was severe (grade III) in 3 of 51 patients (6%) (see web-only Table 16-3 on the Expert Consult website).
WEB-ONLY TABLE 16-3 Mayo Clinic Series: Clinical Peripheral Neuropathy Characteristics with Pelvic IOERT
In a follow-up study, Mayo Clinic investigators evaluated 178 patients with locally advanced colorectal cancer receiving IOERT. This study suggested a relationship between increasing doses of IOERT and the incidence of clinically significant neuropathy (Table 16-4). In primary and locally recurrent colorectal patients the incidence of severe (grade III) neuropathy was approximately 5% and the incidence of any neuropathy was approximately one third. This is consistent with canine studies, suggesting that increasing IOERT doses are related to the incidence of clinical and electrophysiologic neuropathy.47,48
A more recent Mayo Clinic analysis of 607 patients with locally recurrent colorectal cancer receiving IORT reported an incidence of grade 1 to 3 neuropathy of 15% (grade 1, 5%; grade 2, 7%, grade 3, 3%). A dose-related increase in grade 2 and 3 neuropathy was seen in patients receiving 15 Gy or more versus 12.5 Gy or less.50
Results for Selected Diseases
A summary of IORT results and a discussion of future possibilities in selected disease site (pancreas, breast, colorectal, gynecologic cancers, retroperitoneal/pelvic sarcomas) are now presented. For a more detailed discussion of results of treatment for selected diseases the reader is referred to the Expert Consult website and to dedicated chapters on each site in an IORT text.56,57
Pancreatic Cancer
Because of poor local control results achieved with conventional EBRT and chemotherapy, the use of IORT in the setting of pancreatic cancer is rational. The combination of EBRT plus IOERT in the setting of locally advanced/unresectable pancreatic adenocarcinoma has resulted in improvement in local control in separate series from Massachusetts General Hospital, the Mayo Clinic, and Thomas Jefferson University Hospital* (see Table 16-2
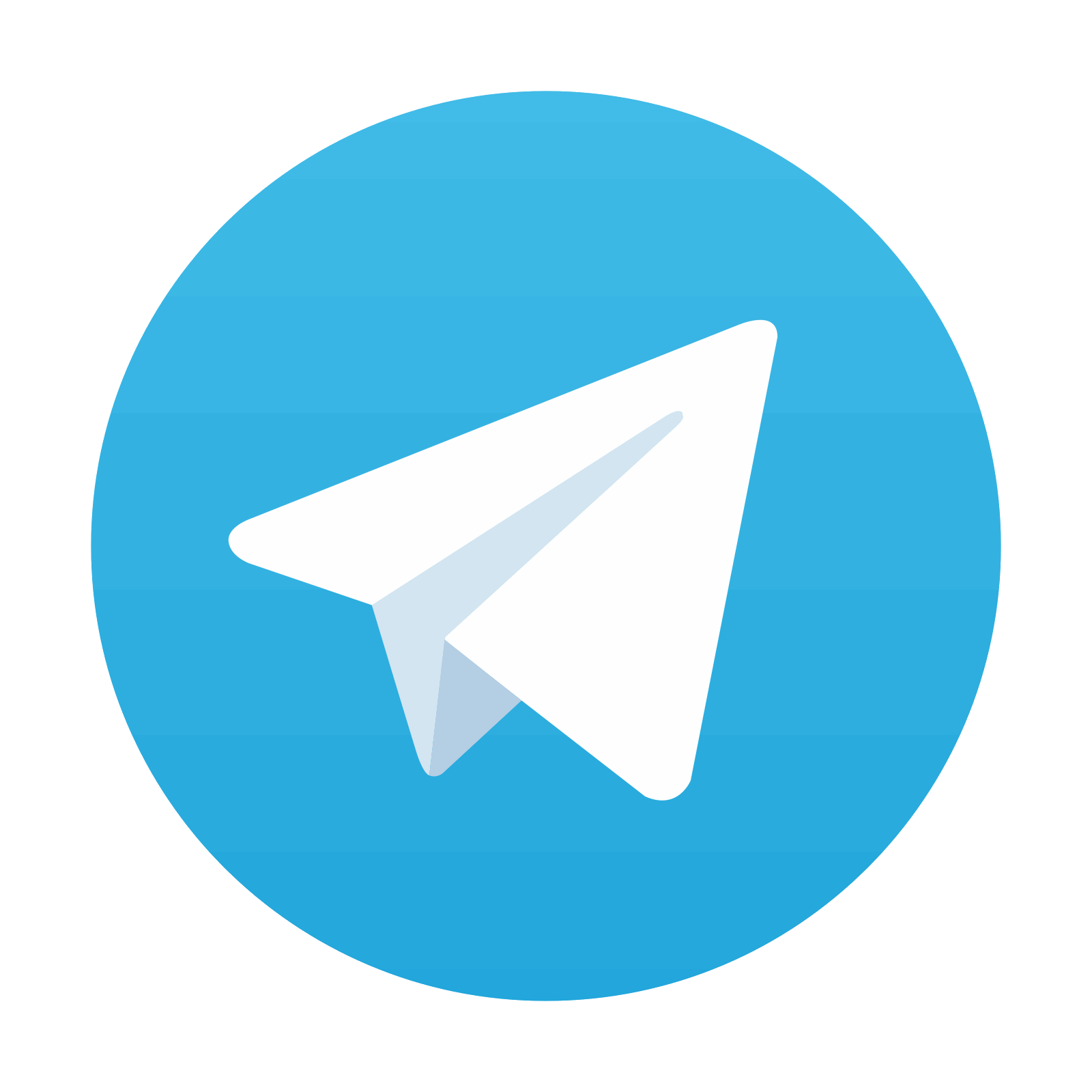
Stay updated, free articles. Join our Telegram channel

Full access? Get Clinical Tree
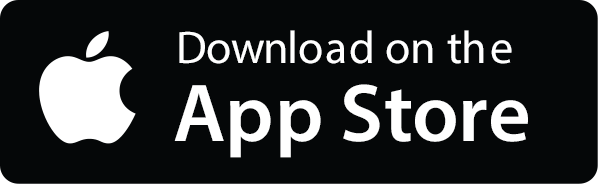
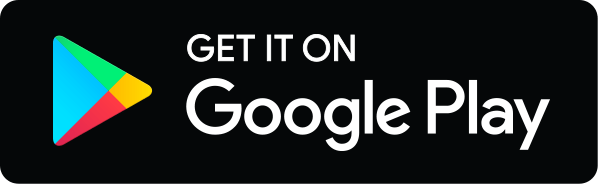