Anemia
Other autoimmune disorder (celiac disease, adrenal insufficiency, pernicious anemia, atrophic gastritis, primary biliary cirrhosis, multiple sclerosis)
Lyme or tick-borne disease infections
Sleep apnea
Impaired sleep (shift work, bad habits or sleep hygiene, menopause, partner with sleep issue)
Previous chronic illness and perception of being worse after thyroid or other cancer diagnosis
Multiple hormone deficiencies (patients with pituitary issues or traumatic brain injury)
Estrogen deficiency (menopause) androgen deficiency (andropause)
Inflammation (infection, diabetes, rheumatoid arthritis, inflammatory bowel disease, poor diet ± abnormal microbiome, food sensitivities, allergies, estrogen deficiency, obesity)
Work-related and/or home-related financial and/or caretaker stress
Endogenous or situational depression and/or anxiety
Chronic fatigue or myalgic encephalomyelitis of uncertain etiologies
PTSD from prior traumatic event including childhood trauma
Malignancy – synchronous or prior to current thyroid cancer diagnosis
Drug and alcohol abuse
Anxiety specific to not understanding illness/treatment or what will happen to self or family
Postoperative hypo- or eucalcemic hypoparathyroidism
T3 deficiency and/or relative hypothyroidism at various tissue levels
There are no current recommendations that therapeutically address cancer-related complaints in thyroid cancer patients. Therefore we can be guided by evidence-based data from complementary therapy trials effective at improving cancer-related complaints (low quality of life, cancer-related fatigue, anxiety, and depression) in patients with non-thyroid cancers. Table 23.2 summarizes modalities discussed in this section. The Society for Integrative Oncology (http://www.integrativeonc.org/) has issued guidelines for complementary therapies in breast cancer [66]. In this population, behavioral therapies (mindfulness/meditation, relaxation, and yoga) have strong evidence (Grade A) for improvement of mood in the context of depression during cancer treatment and meditation for improvement of quality of life and physical functioning. Moderate evidence (Grade B) exists for music therapy, mindfulness meditation, and stress management for reduction of long-term anxiety during and after treatment; massage, acupuncture/pressure, and music therapy for reducing depression; energy conservation for fatigue; and acupressure and electroacupuncture in addition to antiemetics to help control nausea during chemotherapy [66]. Acupuncture at three points (ST36, SP6, LI4 with alternatives at GB34 and SP9) for 20 min for 6 weeks is also significantly effective for improving cancer-related fatigue, on physical and psychological measures including quality of life in a randomized control trial of 302 breast cancer patients [67].
Table 23.2
Complementary mind-body modalities effective in cancer-related symptoms
Symptom | Modality |
---|---|
Anxiety | Music therapy, meditation, MBSR, yoga, CBT, exercise |
Depression | MBSR, relaxation, yoga, massage, acupuncture, acupressure, music therapy |
Quality of life | Meditation, acupuncture, yoga, exercisea |
Cancer-related fatigue | Energy conservation, yoga, acupuncture, CBT + H (radiotherapy), exercise (resistance training) |
Physical function | Meditation, CBT, yoga, exercise |
Nausea | Acupressure, electroacupuncture |
Meta-analysis of randomized control trials of yoga and cancer patients has documented a large effect (d = −69 to −0.75) in reductions in distress, anxiety, and depression and moderate effect in reduction of fatigue (d = 0.49) and improvement quality of life (d = 0.33) and functional well-being (d = 0.31) [68].
Fatigue and muscle weakness were significantly lower at 4 weeks and 6 months after radiotherapy in cancer patients randomized to weekly cognitive behavioral therapy (CBT) sessions and hypnosis during the radiotherapy treatment, in comparison to those that just received compassionate listening [69]. In CBT, the patients identify negative unhelpful beliefs, and the negative consequence these cause for them, and are taught behavioral strategies counteract negative thought patters and to manage fatigue. During the 5–15 min sessions of hypnosis, the suggestion was given for increased well-being and reduced fatigue. Multivitamins are ineffective in reducing cancer-related fatigue [70]. Given these data, it appears that trials of mind-body therapies that include mindfulness meditation, yoga, acupuncture/acupressure, and CBT + hypnosis (H) can be safely recommended to thyroid cancer patients without causing harm and with the expectation of improvement in well-being. In this regard CBT + H may be particularly useful in preparing patients who need to receive radioiodine therapies.
Meta-analysis of the effect of exercise trials in breast and prostate cancer survivors shows a moderate reduction of cancer-related fatigue with effect size of 0.31 (95 % CI: 0.22–0.40) [71]. These investigators noted that moderate intensity resistance training of 3–6 Mets is more effective than lower intensity or aerobic exercise, and older patients benefited more from this than younger patients. Exercise may improve biobehavioral variables that impact quality of life for patients with cancer such as improving quality of sleep, decreasing psychological stress, increasing muscle mass, and functional capacity [72]. Studies that use trans-theoretical models, where patients progressively analyze pros and cons of their own behavioral change in exercise, are more effective at maintaining behaviors [73] and at lowering cancer-related fatigue [71]. A second meta-analysis of trials evaluating the effect of exercise on quality of life and/or quality of life domains (fatigue, anxiety, emotional health) included 40 trials and 3,694 patients. The modes of the exercise intervention included strength training, resistance training, walking, cycling, yoga, Qigong, or Tai Chi. A positive effect of exercise was seen on the global quality of life, breast cancer concerns, emotional well-being, sleep disturbances, anxiety, fatigue, and pain [74]. Quality of life improvements have also been documented in 16 thyroid cancer patients on TSH-suppressive therapy (TSH = 0.2–0.02) who participated in twice weekly exercise for 3 months compared to 16 who remained sedentary [75]. Based on these data, it is sound to recommend exercise with a goal of twice weekly sessions that include resistance training for 45–60 min for thyroid cancer survivors.
Thyroid Hormone Replacement with Levothyroxine Monotherapy and Monitoring Adequacy of Replacement with Thyroid-Stimulating Hormone (TSH)
Thyroid hormone replacement is necessary for all patients undergoing total thyroidectomy, patients with lobectomies who have underlying Hashimoto’s thyroiditis and positive TPO-AB, and for those rendered hypothyroid after radioactive iodine treatment for Graves’ disease or thyrotoxicosis. Levothyroxine is taken orally for thyroid hormone replacement therapy and serum thyrotropin. TSH is measured to determine adequacy of therapy using a normative TSH range derived from individuals without thyroidectomies. This section delineates data on nuances of thyroid hormone replacement and its management and provides a data-based rationale for maintaining TSH ≤ 2.5 mIU/L, after thyroidectomy, in most healthy patients who do not have cancer.
The replacement of thyroid hormone is complex [6–8] due to the multiple biological steps required for the active form of thyroid hormone, T3, to reach target tissues, and the patient-specific factors involved in achieving tissue euthyroidism. All endocrine societies currently recommend that only levothyroxine monotherapy be given for thyroid hormone replacement [8, 76]. This is because it is possible to normalize TSH to the normal range and achieve peripheral conversion of T4 to the active hormone T3 with levothyroxine monotherapy [77, 78], and there are no definitive data to support that combination levothyroxine/T3 therapy is better [8, 79]. A functional guide to dosing of levothyroxine monotherapy is offered in this section. For an in-depth evidence-based discussion of the literature on how levothyroxine therapy is derived and described below, the reader is referred to the 2014 American Thyroid Association (ATA) guidelines for treatment of hypothyroidism [76] Generally, the dose calculation is based on weight, age, and state of health of the patient and indications for therapy. Patients who have had thyroidectomy need full replacement doses. For levothyroxine monotherapy in patients without thyroid cancer, the average replacement dose required is approximately 1.6–1.8 mcg/kg/day for most patients. Higher doses of 2.1–2.7 mcg/kg/day are recommended for patients with thyroid cancer, particularly those with moderate and high risk of recurrence who need TSH suppression as therapy with TSH goals 0.1–0.5 mIU/L and <0.1 mIU/L, respectively [80] (see also Chap. 22).
Patient-related factors influence effectiveness of levothyroxine therapy [76]. We rely on (1) patients to be compliant to taking the medicine daily, or as prescribed, on an empty stomach and in the absence of competing medicines for best absorption, (2) a healthy digestive tract without malabsorption, (3) consistency of drug preparation taken whether generic or brand, and (4) normal thyroid hormone metabolism resulting in adequate T3 conversion from levothyroxine at the tissue level (see below). Elderly patients need lower doses of thyroid hormone, but tend to absorb thyroid hormone less, so particular caution is needed in these patients. Higher doses may be needed in patients with malabsorption syndromes and women who are pregnant or on birth control pills. Different formulations of levothyroxine, such as gel or liquid preparations, may be needed for patients with sensitivity to gluten and lactose intolerances, allergy to color dyes, using proton pump inhibitors, or diagnosed with atrophic gastritis.
In euthyroid individuals, prior to thyroidectomy, 20 % of their bioavailable T3 is secreted directly from the thyroid gland; the rest, or 80 %, is derived from peripheral conversion of T4 by peripheral tissue by enzymes called deiodinases and 5′deiodination of T4 [81]. Patients without thyroid glands cannot produce the 20 % of T3 that their thyroids used to make and secrete and have to rely entirely on the deiodinases (D1 and D2) for the peripheral conversion of T4 to its active form T3. Some individuals have variations or polymorphisms on how their deiodinases convert active hormone T3 from T4, and this may affect their thyroid hormone replacement. Some polymorphisms of the iodothyronine deiodinase 1 (D1) (found in the liver, kidney, and thyroid) gene lead to more peripheral-free T3 from T4 [82], whereas polymorphism of D2 deiodinase activity, normally found in the brain, pituitary, muscular and cardiovascular tissue, and fat (D2 Thr92ala polymorphism, present in 16 % of the population), results in decreased peripheral conversion of T4 to T3 [83]. These latter patients need higher levothyroxine doses to normalize TSH after thyroidectomy [84]. They also feel better with combination levothyroxine/T3 replacement therapy (discussed in section “Combination Thyroid Therapy Trials Levothyroxine and Liothyronine (T3) and Alternative Therapy Trials with Levothyroxine and Desiccated Thyroid for Patients with Persistent Hypothyroid Symptoms and Lack of Wellness” of this chapter) [85]. In addition there are polymorphisms of genes that regulate thyroid pathways and contribute to an individual’s unique thyroid set point by defining how much TSH is needed to create the perfect T4/T3 environment for the individual [86]. Currently we do not have routine ways to detect which individuals carry such polymorphism.
While each individual has its unique set point for the regulation of their thyroid axis and intraindividual variability is narrow [87] after thyroidectomy, the adequacy of thyroid hormone replacement is determined by measuring serum TSH and ascertaining that this TSH value falls within a “normative TSH range,” based on population data of euthyroid people who have not had thyroidectomies. While TSH goal for low-risk thyroid cancer patients is 0.5–2.0 mIU/L, based on recurrence risk assessment (see Chap. 22), for most patients without thyroid cancer, the accepted normal TSH range is broader, 0.45–4.12 mIU/L. This range is based on National Health and Nutrition Survey III population study, which excluded those with personal or family history of thyroid disease or positive antibodies [88]. However, data from national academy of clinical biochemists in the United States suggests that 95 % of individuals without evidence of thyroid disease have TSH below 2.5 mU/L [89]. There are population-based data showing that the upper limit of normal or 97.5 percentile of TSH appears to be age, gender, and ethnicity specific [90, 91]. In fact, there is not just a TSH reference range, but rather a series of shifting curves of TSH distribution defining ranges specific to gender, racial ethnicity, age [92], and iodine exposure history [93]. Given these nuances and patient-specific set points of TSH regulation, it is possible to understand how, after thyroidectomy, even if an individual’s TSH falls within the “normal range,” TSH may not represent euthyroidism at the tissue level for that individual. Most relevant for the patient who has had thyroidectomy, and a major point of this chapter’s section, is that in the cross-sectional assessment of 22,116 patients without thyroid disease used to define normative data for ranges and reference limits for TSH by Bocai et al. [92], 80 % of patients age 59 years or less had TSH < 2.5 mU/L and that 90 % of patients age 70 and above had TSH < 4.5 mIU/l and at all ages analyzed less than 6 % of the population had TSH > than 4.5 mIU/L [92]. This latter point is important because higher TSH values are associated with higher cardiovascular risks [94, 95] and T3 levels cannot be normalized with levothyroxine monotherapy at TSH > 4.5 mU/L [88]. Thus for individuals who have undergone thyroidectomy and do not have thyroid cancer, unless the baseline characteristics of TSH and free T4 relationship are understood prior to surgery, efforts should be made to keep TSH ≤ 2.5 mIU/L in most patients age less than 60 years and the healthy older ones raised in iodine-sufficient countries, and no higher than 4.5 mIU/L for elderly patients.
TSH range in pregnancy after thyroidectomy is similar to that of patients with thyroid disease who receive thyroid hormone therapy during pregnancy to prevent adverse effects of maternal hypothyroidism or hyperthyroidism on maternal and fetal outcomes [96]. All these patients cannot compensate for increased thyroid hormone demands in normal pregnancy [97]. It is necessary to increase thyroid hormone replacement doses by about 30 % more than nonpregnant values [98]. This can be accomplished by increasing the prepregnancy dose by adding an extra thyroid pill 2 days of the week during the first trimester and closely monitoring patients thereafter [98]. The TSH ranges recommended are 0.1–2.5 mIU/L in the first trimester, 0.2–3.0 mIU/L in the second trimester, and 0.3–3.0 mIU/L in the third trimester and a total T4 that is 1.5 times the normal range [99–101].
Combination Thyroid Therapy Trials Levothyroxine and Liothyronine (T3) and Alternative Therapy Trials with Levothyroxine and Desiccated Thyroid for Patients with Persistent Hypothyroid Symptoms and Lack of Wellness
Levothyroxine monotherapy may not be the ideal replacement after thyroidectomy for some individuals [102, 103]. Studies suggest that 12 % of hypothyroid patients do not feel well on levothyroxine alone and have symptoms that are suggestive of hypothyroidism despite having TSH in the normal range [104]. This is a particular group of patients that may benefit from combination therapy trials [77, 105]. While it has been reported that combination therapy with levothyroxine and slow release liothyronine (T3) at a dose of 6 mcg resulted in more physiologic thyroid parameters and more normal free T4/T3 molar ratios [106], to date, there are no reliable slow release preparations of liothyronine available (or FDA approved). The concern for adverse effects due liothyronine overdosage, the rapid onset and short half-life of currently available liothyronine preparations, and the high content of T3 in commercially available porcine-derived desiccated thyroid preparations have all become hurdles to the routine use of liothyronine therapy alone or in combination therapy for patients after thyroidectomy.
The literature available on combination levothyroxine + liothyronine therapy is difficult to interpret due to the heterogeneity of patient populations treated, different dosing protocols, short duration, and different endpoints which may or may not reflect hypothyroid symptoms, or the tissue response at a biological meaningful level [6, 8, 107]. One study found benefit of combined therapy after thyroidectomy [108] but others have not [109]. Even though meta-analyses of randomized controlled trials have not found benefit of combined levothyroxine/liothyronine replacement versus levothyroxine monotherapy, combined therapy is preferred by hypothyroid patients in these trials [8, 110–113].
Both the ATA and European Thyroid Association (ETA) recognize that it is reasonable to give an experimental trial of combined levothyroxine and liothyronine to patients who continue to not feel well on levothyroxine monotherapy [8, 76]. Because liothyronine is rapidly absorbed and results in a peak 4 h after ingestion, it is prudent to use the lowest amount possible per dose, and to avoid use in patients with arrhythmia, cardiovascular compromise, osteoporosis, or pregnant women. Table 23.3 offers suggestions for the use of combination therapies for patients who desire such trials with combination therapy or alternative therapy (see below) with levothyroxine and/or desiccated thyroid (like Armour or Nature-Throid). These combinations are based on bioequivalence data of the hormones [50, 51], knowledge that the molar ratio of T4 to T3 secretion in humans is 14:1 [78], and supported by the suggested calculations of physiologic ratios with T3 and T4 hormones from the ETA [8]. They suggest methods of accounting for potential individual differences in levothyroxine and liothyronine absorption and for calculating levothyroxine and liothyronine doses, in such patients, that maintain the physiologic T4/T3 molar ratio using molar ratios of 13:1–20:1. It is suggested that the liothyronine dose is split, if possible, with the largest dose given at bedtime [8] to attempt to simulate the circadian rise in T3 that naturally happens in normal patients around 3 AM following the TSH surge [114]. This calculation (see T4:T3 ratios in Table 23.3) allows for liothyronine doses much lower than doses used in randomized controlled trials. The higher T4:T3 ratios could be used in patients with moderate to higher adverse risks to thyroid hormone. Another simple approach, used by this author, to do combination trials in healthy individuals, solely based on bioequivalence data [51, 52], is to replace 35 mcg of levothyroxine monotherapy with 10 mcg of liothyronine per day, the latter split in two doses.
Table 23.3
Dose approximations for alternative thyroid replacement therapy trials using combinations of levothyroxine (LT4 mcg) with liothyronine (T3 mcg) or desiccated thyroid (DT, grain) equivalent that maintain T4:T3 daily dosing ratiosa between 14:1 and 19:1
Baseline LT4 mcg dose | LT4:T3 ratios 14:1 16:1 19:1 | LT4 × tablets/week + T3 dose × tablets/week | LT4 tablets/week + DT × tablets/week |
---|---|---|---|
25 | 20:1.6 20:1.47 21:1.25 | 0 mcg × 7 + 0.25 g × 7 | |
50 | 40:3.3 41:2.9 43:2.3 | 50 mcg × 5.5 +2.5 mcg × 7 | 25 mcg × 7 +0.25 g × 7 |
75 | 60:5 61.7:4.4 63.7:3.75 | 50 mcg × 8 +5 mcg × 7 | 50 mcg × 5.5 +0.50 g × 7 |
88 | 70:5.6 72:5.17 74.8:4.4 | 75 mcg × 5 +5 mcg × 7 | 50 mcg × 7 + 0.50 g × 7 |
100 | 80:6.6 82:5.88 85:5 | 75 mcg × 7 + 5 mcg × 7 | 50 mcg × 7.5 + 0.50 g × 7 |
112 | 89:7.4 92:6.5 95:5.6 | 88 mcg × 7 + 7.5 mcg × 7 | 75 mcg × 7.5 + 0.50 g × 7 |
125 | 100:8.3 103:7.35 106:6.25 | 100 mcg × 7 + 7.5 mcg × 7 | 75 mcg × 7 + 0.75 g × 7 |
137 | 109:9.1 113:8.0 116:6.85 | 112 mcg × 7 + 7.5 mcg × 7 | 88 mcg × 7 +0.75 g × 7 |
150 | 120:10 123:8.8 127:7.5 | 125 × 7 +10 mcg × 7 | 88 mcg × 7 +1.0 grain |
175 | 140:11.6 144:10.2 148:8.75 | 137 mcg × 7 + 10 mcg × 7 | 112 mcg × 7 + 1.0 grain |
200 | 160:13.3 164:11.7 170:10 | 175 mcg × 7 + 10 mcg × 7 | 125 mcg × 7 + 1.25 grain |
300 | 240:19.8 246:17.6 255:15 | 200 mcg × 9 + 15–20 mcg × 7 | 200 mcg × 7 + 1.5 grain |
An advantage of levothyroxine and liothyronine combinations over desiccated thyroid preparations is the fact that each hormone can be adjusted individually and that one can avoid the undesirable high content of T3 in available fixed doses desiccated thyroid preparations, such as Armour or Nature-Throid, where the molar ratio is 4.3:1 (60 mg having 38 mcg T4 and 9 mcg T3, 15 mg 9.5 mcg T4 and 2.37 T3) [115] or that of European preparations with T4:T3 combinations of 10:1 in Prothyroid, 5:1 in Novothyral, and 4:1 in Thyreotom, and deal with the fact that the bio-availability of T4 and T3 is similar after ingestion from all the oral preparations [116].
Individual adjustments of liothyronine or T3 are usually necessary to increase serum free T3 and lower free T4 (fT4). Giving more levothyroxine only increases fT4 dose but does not increase endogenous free T3, as the deiodinases are suppressed at higher fT4 and suppressed TSH levels. This author aims for serum free T3/free T4 ratio of about 3, which is found in healthy individuals [117].
This author likes 3-month cycles for combination and alternative therapy (see below) trials in order to bypass a potential placebo effect, which can last up to 6 weeks, and allow patients to have an honest assessment of their well-being. Some patients return to standard levothyroxine monotherapy, while others have a clear preference for combination therapies. Some patients find it a nuisance to take thyroid more than once a day, or to cut liothyronine in small doses, or pay multiple co-pays using two thyroid preparations.
An example for trial of combination levothyroxine + liothyronine therapy based on ETA recommendations, from Table 23.3, is a patient who was on 50 mcg levothyroxine daily and in a trial could take the equivalent of 40 mcg daily by taking 50 mcg tab per day for 5 days and ½ tab the 6th day, and none on the 7th day + 1/2 tablet of 5 mcg liothyronine daily at night to achieve the 14:1 ratio dosing.
In addition to trials of combination therapy with standard levothyroxine and liothyronine, patients want to try compounded thyroid preparations or desiccated “natural” thyroid. The Endocrine Society, ATA, and ETA do not recommend that thyroid combinations be compounded by pharmacies, because of potential for errors leading to iatrogenic hypo- and hyperthyroidism, and this author strongly supports this recommendation. Similarly desiccated thyroid hormone preparation is not recommended for thyroid replacement due to its high T3 content. Therefore the use of desiccated thyroid is considered nonstandard therapy or alternative therapy. For physicians, this is an ethical dilemma, as we are bound to help with evidence-based treatments and to cause no harm. Education and flexibility in negotiation is recommended so that patients, with their preferences considered, are appropriately treated while protecting them from harm. It is important to acknowledge patients’ interest in self-regulation and to work toward a long-term healing partnership with the right therapy. This may discourage patients from pursuing alternative care by nonmedically trained practitioners, who may administer even more unregulated “thyroid” preparations. Physicians always have the choice of opting out of participation in these trials and refering patients to another practice. From an integrative medicine perspective, it would be safer for our patients and more rewarding for us as physicians to oversee our patients’ individual trials of thyroid preparations. This can be achieved by having mutual agreements on the goals for treatment and potential risks of the therapy (which symptom(s) will be addressed by the trial and a signed consent), making sure there are no contraindications and that potential causes of lack of wellness, as noted in Table 23.1, have been addressed.
This author finds that small doses of desiccated thyroid may be used judiciously and safely, alone or in combination therapy with levothyroxine for patients who want to try it and are deemed to be reliable and healthy. Desiccated thyroid offers dried entire thyroid contents minus connective tissue, including other thyronines, T1 and T2, which may have physiologic metabolic functions [118]. 3,5-T2 is also made by peripheral tissue conversion, it is measurable in athyreotic patients, and it is not regulated as T3 and T4 are by TSH [119]. An example of such alternative trial from Table 23.3 is a patient whose initial dose is 75 mcg/day of levothyroxine monotherapy. He or she can take the equivalent of 40 mcg daily of levothyroxine by taking 50 mcg tab per day for 5 days and ½ tab the 6th day, and none on the 7th day and adding 0.5 grain desiccated Armour daily: The Armour dose should be split to 0.25 g for twice daily dosing. Most patients are not compliant with the ideal thrice daily dosing for any T3-containing preparations. It is best to avoid doses of desiccated thyroid above the 1–1.5 grain (60–90 mg) range, and to add levothyroxine as needed to achieve treatment goals, while avoiding adverse risk or use in at-risk populations. This author uses the 0.25 grain or 15 mg tablet dose of desiccated thyroid to supplement levothyroxine and 5 mcg tablets of liothyronine to supplement T3 for combination therapy trials.
Patients receiving combination therapies should aim for goal TSH recommended for their diagnosis (section “Thyroid Hormone Replacement with Levothyroxine Monotherapy and Monitoring Adequacy of Replacement with Thyroid-Stimulating Hormone (TSH)”) and have free T3 and T4 levels measured 6–8 weeks after each dose change. Note that measurements of serum total or free T3 are currently discouraged and not considered or recommended as outcome measure in the treatment of hypothyroidism. Neither the levothyroxine, liothyronine, nor desiccated thyroid should be taken the morning of blood testing to avoid transient peaks in hormone levels (17 % for T4 and up to 42 % for T3) which occur after ingestion [120].
Dealing Well with Radioactive Iodine: Addressing Mind-Body Concerns and Complementary Approaches to Patients Receiving Radioiodine Therapy
The goal of this section is to address emotional issues experienced by patients when they undergo radioiodine ablations or adjuvant therapy and create awareness of potential confounders with iodine-containing supplements in patients receiving I131.
Patients experience considerable stress over the process of undergoing radioactive iodine scans, ablations, and adjuvant treatments [34]. After agreeing to receive radioiodine, dietary changes need to render the patient iodine deficiency for optimization of tissue ablation. During the exposure to radioiodine, patients are concerned about contaminating their homes and their families, particularly children. Patients do not like the isolation necessary for the process and much less the potential side effects of nausea, fatigue, acute and chronic sialadenitis, xerostomia, dysgeusia, and small (1 %) but significant increased risks for future secondary malignancy, particularly leukemia [121–124]. The ATA issued guidelines to limit the public’s risk of radiation exposure after a patient receives radioiodine treatment, provided useful outlines to identify patient’s capability to manage the process, and help identify patients who need more guidance or are best served by hospitalization during the treatment process [125]. Education of patients using patient decision aids helps them be more confident about the decision to receive radioiodine [126]. Meeting with radiation safety staff and a nuclear physicist can further assist with safety concerns regarding exposures to family and home.
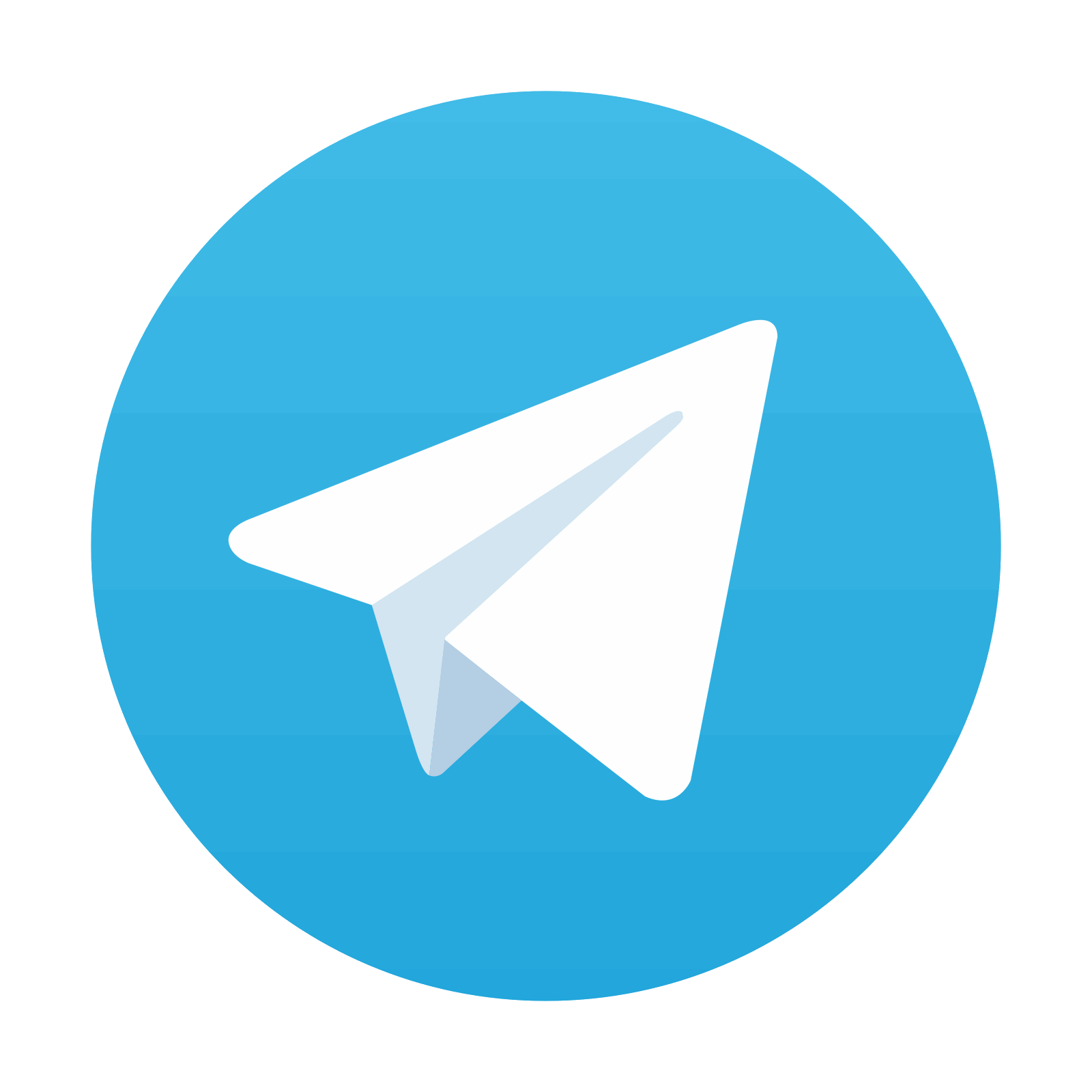
Stay updated, free articles. Join our Telegram channel

Full access? Get Clinical Tree
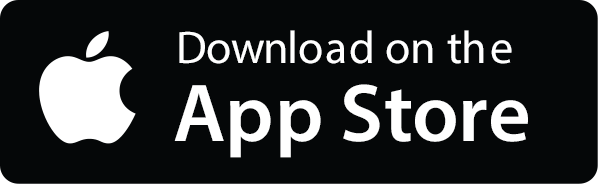
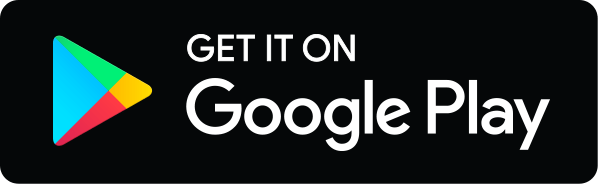