Abstract
The relationship between a living organism and its environment is based on a tightly regulated balance between symbiosis and competition. The complexity of an organism is directly correlated to the sheer volume of challenges presented to its continued fertility. The systems in higher organisms that function to protect against biological insults are collectively referred to as the immune system. The immune system may act as friend or foe. Immune systems of higher vertebrates can be roughly subdivided into two major components: a phylogenetically older innate immune system, and the more recently evolved adaptive immune system. The diversity of environments that humans occupy is staggering; thus the human body has remarkably elaborate mechanisms to protect the individual from foreign pathogens.
During development, the innate and adaptive arms of the immune system are programmed and learn to distinguish self from nonself. Despite the safeguards of the immune system, some self-reacting lymphocytes are not inactivated or removed and can cause one of several illnesses we know as autoimmune diseases. Immunologists are well aware that if they understood all the mechanisms for tolerance induction, they might be able to prevent autoimmune diseases, and conversely, focus the potent tool-kit of immunity against foreign pathogens and the “altered self” of cancer.
The failure of tolerance-induction mechanisms to prevent the recognition of “self” antigens as foreign by the immune system may result in an autoimmune response. Thus autoimmunity refers to an immune reaction of the body against substances that are normally present in the body. To understand autoimmunity, it is important to first understand tolerance. Tolerance can best be defined as a state of antigen-induced immunologic unresponsiveness. (Autoimmunity can be considered a failure of tolerance.) Tolerance is not synonymous with generalized immunosuppression; it is antigen-specific and causes no impairment of the immune response to antigens other than the ones used to induce tolerance.
Implantation is one of the most important aspects of pregnancy that immunologic studies have targeted. Implantation represents a critical developmental process in that it requires the interaction of immunologically and genetically distinct tissues. The immune system may influence pregnancy success or failure during any of the critical steps of implantation. Embryo attachment occurs only during the “implantation window,” the time period when the endometrial epithelium is receptive and the embryo is hatching and competent for attachment. Failure of this synchronization precludes success, as demonstrated in human studies of implantation.
Studies of the karyotype on products of conception from early recurrent pregnancy loss have shown that many of these losses are chromosomally normal human embryos. Human preimplantation embryos express major histocompatibility antigens theoretically capable of inducing an immune response, but the role of these antigens in pregnancy has not been delineated. It is possible that maternal immune responses play a role in the failure of implantation.
Keywords
Reproductive immunology, maternal-fetal allograft, mucosal immune system, alloimmunity, autoimmunity
Basic Immune Principles
- ◆
Responses of the innate immune system are generally rapid, do not exhibit antigen specificity, and lack memory; those of the adaptive immune system are antigen specific and include primary responses and secondary, memory-driven responses.
- ◆
Immune specificity relies on a lymphocyte education process that distinguishes self from nonself. T lymphocyte education is dependent on recognition of self–major histocompatibility complex (MHC) restriction; B lymphocyte education is not.
- ◆
As a part of the mucosal immune system, the first line of defense against many pathogens, the immunologic interactions in the female reproductive tract are biased toward innate immunity.
Innate and Adaptive Immune Defense Systems
The importance of effective defenses against foreign invaders has led to development of an innate (or natural) and an adaptive (acquired, or specific) immune defense system. While these immune systems often cooperate in their defense against foreign invaders, their responses differ in intensity, timing, and specificity ( Table 13.1 ).
Characteristic | Innate Immunity | Acquired Immunity |
---|---|---|
Specificity | Nonspecific | Antigen-specific |
Physicochemical barriers | Skin, mucous membranes | Peripheral and mucosal immune systems |
Effector cells | NK cells, macrophages | B and T lymphocytes |
Circulating molecules | Complement | Antibodies |
Soluble mediators | Macrophage-derived cytokines (IFN-α, IFN-β, TNF-α) | Lymphocyte-derived cytokines (IFN-γ) |
Response time | Rapid | Primary and secondary |
Memory | None | Long-lived |
Innate Immunity
The innate or natural defenses against pathogens consist of tissue barriers, phagocytic and cytotoxic cells, and a variety of effector molecules. These defenses at the skin and mucous membranes may be perfectly adequate to clear potentially harmful environmental antigens. The initial entry of foreign organisms or cells is met by an initial inflammatory response which may be amplified by the activation of serum complement components resulting in the generation of enzymes and the deposition of their products on the surface of foreign antigens. The innate system can differentiate between a harmful invader, such as bacteria or viruses, by promoting an offensive environment. Other invaders, such as sperm or blastocyst, result in an inflammatory response that is accommodating to the guest. However, this first exposure to an antigen, despite intensity elicited, will not alter the type and magnitude of innate immune response upon reexposure. In this sense, innate response to reexposure to same or unrelated antigen(s) will not exhibit “memory” and is generally unaltered from initial response.
Acquired Immunity
The acquired immune response is characterized by an adaptation to the first exposure to the foreign antigen, known as the primary response . A subsequent exposure to the same antigen results in a secondary response that is quantitatively and qualitatively different than the primary response. The acquired immune response consists of antigen-specific cells and molecules that often interface with components of the innate immune defense systems. During opsonization, antibodies bind to bacterial surface antigens facilitating their phagocytosis by macrophages. In addition, macrophages may process and present protein antigens to specific T cells during a primary immune response.
Antigen-specific lymphocyte responses are characterized by their activation and proliferation of previously antigen-naïve lymphocytes, followed by functional differentiation towards production of soluble mediators including antibodies and cytokines and the development of antigen-specific memory. A typical primary response to antigen has a lag phase of about 5 days and has a slow rate of increase to a low plateau with a low sensitivity to the antigen. The low-affinity antibody is predominantly pentameric immunoglobulin (Ig)M. Over the course of approximately 4 to 8 weeks, B cells specific for antigen proliferate and undergo affinity maturation (selection for B cells producing higher-affinity antibodies) and class-switching (from IgM to IgG, IgA or IgE). The secondary response (and all subsequent memory responses ) to the same antigen are quantitatively and qualitatively different. The response occurs in 1 to 2 days, rises rapidly to a high plateau, and has a high degree of sensitivity to the antigen. The antibody affinity is high and may be predominated by IgG, IgA, or IgE depending on the location in which the immune response occurs.
The major features of the specific immune response are consistent with Burnett’s clonal selection theory. This hypothesis states that (1) the antigen specificity of a single lymphocyte is established in the absence of antigen during its initial maturation, (2) each lymphocyte has a unique antigen specificity manifested by multiple copies of the same receptor, and (3) the proliferation and differentiation of the lymphocyte is induced by antigen binding to the specific receptors. The interaction of antigens and lymphocyte receptors is governed by the relative affinity of the receptor for the antigen. Clonal selection explains subsequent responses to the same antigen are more rapid, robust, and long lasting. The proliferation of specific lymphocytes in response to antigen results in the generation of antigen-responsive clones of cells.
As a consequence of antigen-induced proliferation, a small proportion of the proliferating lymphocytes commits to long-term memory cell fate that persists for many years. Activated B cells may undergo isotype switching by changing from the production of IgM to IgG, IgE, or IgA, thus allowing different physiologic and biologic properties. By these mechanisms, a maturing immune response maintains antigen specificity but establishes memory and functional diversity.
The development of adaptive immunity based on the clonal selection of lymphocytes bearing specific receptors was critical in allowing all pathogens, no matter how novel, to be recognized and in allowing the development of immunologic memory.
Basic Effectors of Immune Responses
Cellular Effectors
Leukocytes are the cellular effectors of immunity and include the lymphocytes, monocytes, macrophage, dendritic cells, neutrophils, basophils, and eosinophils ( Table 13.2 ). Lymphocytes can be further divided into subclasses based on function and upon cell surface markers, called cluster of differentiation (CD) markers. Lymphocyte subclasses include T cells, B cells, and natural killer (NK) cells. Both B and T lymphocytes originate in the bone marrow and participate in antigen specific immune responses.
Characteristic | T Cytotoxic T Regulatory | T Helper T Inducer | B Cells | NK Cells |
---|---|---|---|---|
Symbol | Tc/reg | Th | B | NK |
Surface antigen | CD8+ | CD4+ | CD19+ | CD56+ |
MHC restricted | Class I | Class II | Class I and II | None |
Target cells | Tumors, virally infected cells, allografts | B cells, Tc cells and precursors, macrophages | — | Tumor cells, virally infected autologous cells |
Function | Kill foreign cells, downregulate cells | Interleukin secretion | Immunoglobulin production | Immune surveillance, cytotoxic |
Differentiation | Thymus | Thymus | Bone marrow | Bone marrow |
Specificity | Antigen peptides | Antigen peptides | Native antigen epitopes | Unknown |
Antigen receptor | T-cell receptor | T-cell receptor | Cell surface immunoglobulin | Fc portion of immunoglobulin |
T (Thymus Derived) Cells
In humans, T cells circulate through the thymus, where they gain specific CD markers, antigen specificity, and tolerance to self (see subsequent text). T lymphocytes maturing in the thymus express both CD4 and CD8 cell surface receptors during early development, but those exiting the thymus express only one of these cell surface markers. CD4 positive T cells typically develop into helper T cells when they reach peripheral lymphoid tissues. Helper T cell effector functions include the secretion of soluble mediators of immune responses such as cytokines. These cytokines, in turn, modulate B cell, T cell, and macrophage responses. A subset of CD4 T cells differentiates into regulatory T cells, key suppressors of autoimmunity. CD8 positive T cells leaving the thymus are destined to mature into either cytolytic T cells (CTLs), which function to lyse infected or otherwise altered target cells, or into suppressor T cells, which are currently called regulatory T (Treg) cells.
B (Bone Marrow Derived) Cells
B cells appear to be educated in the bone marrow prior to exit into the peripheral immune system. Similar to T lymphocytes, B lymphocytes are antigen specific. Unlike T lymphocytes, B cells function to secrete antibodies, which characterize humoral immune responses (see below).
Innate Lymphoid Cells
Innate lymphoid cells (ILCs) are the third general class of lymphocytes, sharing transcriptional and cytokine effector profiles with adaptive T cells but lacking specific antigen receptors. NK cells were previously thought to be the only innate lymphocytes but are now recognized to comprise only a subset of a much more diverse and complex system collectively known as ILCs. A classification system for ILCs has been recently agreed upon and categorizes ILCs into three groups: (1) group 1—ILC1s and NK cells in intraepithelial (intestinal and other epithelial, inflamed mucosal, and tonsillar) locations; (2) group 2—ILC2s in human lung and intestinal tissue (also in spleen and liver in mice); and (3) group 3—ILC3s, which are predominantly in gut-associated mucosal and lymphoid tissues.
NK cells have characteristic cell surface markers and receptors, and their activities are typically not antigen specific, although a form of surface receptor repertoire tuning is reflected in their long-term cellular identity. NK cells function in the recognition of cells lacking MHC class I products (see below) and in first-line defense against virally infected or oncogenically transformed target cells. NK cells also display cell surface receptors, allowing recognition of antibody-coated target cells. Through these receptors, NK cells function as the major effector of antibody-dependent cellular cytotoxicity (ADCC).
Monocytes, Macrophage, and Dendritic Cells
Similar to other leukocytes, monocytes are derived from bone marrow stem cells. Monocytes circulate in the peripheral blood and populate nearly every tissue. Within specific tissues, monocytes mature into macrophages. In some tissues, these macrophages have been given specific names (e.g., alveolar macrophages in the lung and Kupffer cells in the liver). Macrophages are phagocytic cells that participate in the development of innate as well as antigen-specific immune responses. They can signal T and B cells via secretion of cytokines and via delivery of antigen in forms recognized by these cells (antigen presentation). They may also stimulate direct lysis of altered target cells. Dendritic cells are closely related to macrophages, and most derive from a similar cellular lineage in the bone marrow. Similar to macrophages, dendritic cells are phagocytic. Unlike macrophages, dendritic cells are distinguished by their pivotal role in antigen presentation, a process necessary for naïve T-cell priming and subsequent development of antigen-specific B-cell responses.
Other Effector Cells
Neutrophils, eosinophils, and basophils are effector cells with specific importance in innate defense against pathogens. Each has also been associated with specific immune-mediated disease states. Eosinophils, for instance, are classically associated with defense against parasites and have pathophysiologic importance in asthma.
Soluble Components of Immune Responses
Immunoglobulins and Humoral Immunity
Ig molecules are composed of dimerized subunits of heavy and light chains ( Fig. 13.1 ). The N-terminal portions of each of the heavy and light chains are highly polymorphic and are termed variable regions . The variable region of one heavy chain combines with the variable region of one light chain to give the Ig its antigen specificity. The C-terminal segments of the Ig heavy and light chains are called constant segments and have minimal polymorphism. The constant portion of the Ig heavy chain interacts with other immune response components. Since these interactions are governed by heavy chain constant region isotypes, the Ig effector functions are also governed by the isotypes. Ig molecules isotypes include IgA, IgD, IgE, IgG, and IgM. IgG, IgE, and IgD molecules are typically present as single Ig molecules (monomers). IgA molecules usually circulate as dimers and IgM molecules as pentamers ( Fig. 13.2 ).
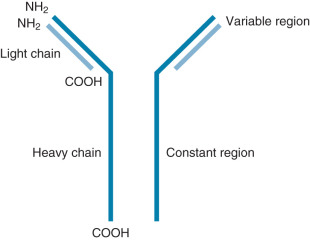
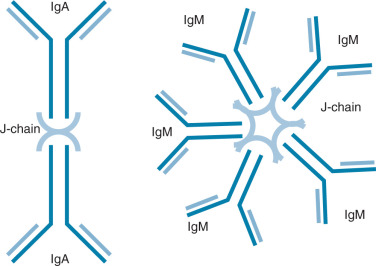
Each Ig isotype has distinctive functions. IgA dimers, connected by a joining or J chain, have been best described in the mucosa of the intestine and the female reproductive tract. Here, they are actively transported into the mucosal lumen via interaction of the J chain with mucosally derived secretory components. This allows exposure of IgA to antigens at the mucosal surface. IgE molecules interact with eosinophils in parasitic infections, allowing eosinophil-mediated target lysis. IgE molecules are also implicated in delayed-type hypersensitivity (DTH) via mast cell interactions. Membrane-bound IgD and IgM monomers are components of the antigen-recognizing B-cell receptor on naïve B cells. Pentavalent IgM molecules activate the complement cascade. Secreted IgM multimers are also characteristic of the early antigen specific responses of naïve B cells.
Primary and Secondary Immune Responses
Collaboration between helper T cells and B cells is essential during the generation of primary immune responses. B cells expressing an antibody surface receptor bind to and internalize an antigenic particle (e.g., bacterium, virus). The particle is digested within B cells and peptide fragments presented to circulating helper T cells by B cell-expressed MHC molecules. If a circulating, activated helper T cell recognizes the peptide antigen on the B cell, it will in turn provide an activating signal (through direct cell-cell receptor/ligand and soluble cytokines), allowing this B cell to proliferate and produce antibody molecules—a process termed licensing . Continued helper T cell activation in local lymph nodes is necessary to trigger affinity maturation, antibody class switching appropriate to tissue locale, and the formation of immunologic memory. Without strong and sustained T-cell help, B cells will produce mostly IgM and will not form immunological memory. As described earlier, the initial or primary immune response requires fairly significant antigenic stimulus and typically peaks approximately 5 to 10 days after antigen exposure. Primary responses usually involve more IgM secretion than IgG and the magnitude of the response is often lower than that after a second exposure to peptide antigen. Upon reexposure to the same antigen, a secondary response ensues. Secondary responses require less antigenic exposure than primary responses and they peak more rapidly (2 to 5 days postexposure). Secondary responses are typically more robust than primary responses and often do not require T-cell help. As a result of isotype switching, IgG rather than IgM molecules represent the predominant Ig subtype in secondary immune responses.
These IgG molecules have multiple immune effector functions. They can cross the placenta, allowing immune transfer from mother to fetus. IgG can directly bind antigen via its variable region. This allows the free constant portion of the IgG heavy chain (Fc portion) to be recognized and internalized by phagocytic cells in a process termed opsonization. Similarly, IgG bound to cell-associated antigen can signal lytic attack by cytotoxic T cells, NK cells, or NKT cells, promoting a process called ADCC. Finally, IgG molecules can activate the complement cascade.
Complement
The complement cascade is a vital part of innate immunity. It may be useful to compare the complement cascade to a more familiar clinical entity, the coagulation cascade. Both have components that circulate in inactive forms. Activation of each pathway can occur via two mechanisms. Reminiscent of the intrinsic and extrinsic coagulation pathways, the complement cascade can be activated via classical and alternative pathways ( Fig. 13.3 ). The classical pathway is activated when a complement component C1 binds to antigen-antibody complexes (IgG or IgM). The alternative pathway is activated when complement component C3b binds to activating surfaces such as the cell wall of a bacterial pathogen. Each pathway sets off a series of activating enzymatic digestions of subsequent complement cascade components. Similar to the coagulation cascade, both of the activation pathways in the complement cascade intersect, joining as a final common effector pathway. Late components of the complement cascade can interact with specific complement receptors, resulting in pathogen phagocytosis and/or activation of the humoral immune system. Alternatively, activation of the complement cascade can result in the formation of a membrane attack complex (MAC) that results in indirect osmotic lysis of a target cell via the formation of an ion permeable transmembrane pore in the target cell.
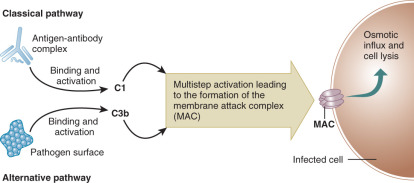
Cytokines
The rapidly enlarging and pleiomorphic family of cytokines joins the immunoglobulins and the components of the complement cascade to complete a list of important soluble mediators of immune responses. Cytokines are a family of secreted proteins, all of which are generated by immune cells. They include the interleukins, the interferons, tumor necrosis factors, transforming growth factors, and the chemokines. The actions of cytokines may be autocrine, paracrine, or endocrine, and effects are mediated by specific cytokine receptors. Most cytokines have short half-lives, so direct actions are typically of short duration. However, cytokines often stimulate additional immune cell activity with cascading cytokine secretion and effects. Members of this large family of soluble immune mediators often have complementary and/or redundant activities.
In addition to myriad other roles in immunobiology, cytokines help to direct T helper cell differentiation. CD4+ T helper cells appear to circulate from the thymus to peripheral tissues in a functionally immature state (Th0). The T helper cell phenotype/polarization model holds that Th0 cells differentiate into their mature phenotypes based largely upon the cytokine microenvironment in which they reside at the time when they first recognize antigen and on the cell surface coreceptors expressed on the Th0 cell ( Fig. 13.4 ). Certain cytokines appear to be of particular importance in the polarization of T helper cell responses. If antigen is recognized by Th0 cells in an environment dominated by the cytokines interleukin 12 (IL-12), IL-18, and interferon gamma (IFN-γ), the resulting T helper effector phenotype is characterized by the secretion of inflammatory-type cytokines, including IFN-γ, interleukin 2 (IL-2), and tumor necrosis factor alpha (TNFα). This type of response is called a T helper 1 (Th1) response. Alternatively, if Th0 cells recognize antigen in a microenvironment dominated by IL-4, the predominant T helper response is of the Th2 type and is characterized by secretion of IL-4, IL-5, IL-9, and IL-13. These Th2 cytokines, in turn, stimulate allergic-type responses, including mast cell and eosinophil activation and antibody production. Th1 responses enhance Th1 responses while inhibiting Th2 responses. Th2 responses initiate a positive feedback loop, which promotes further Th2 activity while inhibiting Th1 effects. Several other T helper responses have also been distinguished. Th3 and Treg1 responses are characterized by the secretion of transforming growth factor beta (TGF-β); Treg responses are defined by the secretion of IL-10, and Th17 cells are defined by the secretion of IL-17.
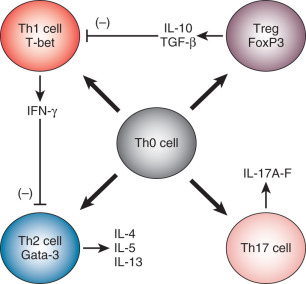
Altered T helper cell polarization may lead to immune-mediated diseases. Although the etiologies are often incompletely described, these disorders are characterized by a dysregulation of cytokine secretion. For instance, patients with some forms of asthma appear to have inappropriate polarization of immune responses in their lungs towards the Th2 and Th17 phenotypes. Those with Crohn disease polarize intestinal mucosal immune responses toward the Th1 phenotype. It has been hypothesized that some cases of spontaneous isolated and/or recurrent pregnancy loss (RPL) may be the result of an insufficient Th2 polarization of maternal lymphocytes in the presence of placental antigens.
Basis of Immune Specificity and Immune Cell Education
While many of the salient attributes of the immune system can be addressed in a description of the cellular and soluble components of immune responses, there are two additional defining features of immune responses that require specific discussion: the specificity of immunologic antigen recognition and T lymphocyte education.
Antigen Presentation
Most of the antigens recognized by the effector cells involved in acquired immune responses cannot be detected in isolation. Rather, antigens must be specifically presented to effector cells. This presentation process places antigens into a context in which the cell presenting the antigen can be recognized by the effector cell as “self-derived,” and the antigen can be seen as foreign. This process is called antigen presentation and it is essential to antigen specific development of both cellular and humoral immune responses.
Major Histocompatibility Complex
The MHC region on the short arm of human chromosome 6 is a 4-million base-pair DNA segment that encodes many of the molecules involved in innate and acquired immune responses ( Fig. 13.5 ). This highly polymorphic DNA region contains nearly 130 genes and approximately 100 pseudogenes. Not all of these genes are linked to immunity, but two sets of genes within the region play central roles in antigen presentation. These genetic loci encode the MHC class I and MHC class II products ( Fig. 13.6 ) and several other molecules involved in antigen presentation. MHC class I molecules (e.g., human leukocyte antigen [HLA]-A, -B, and-C) are present on the surface of nearly every cell in the human body. They aid in the defense against intracellular pathogens such as viruses by presenting virus-derived antigens at the surface of infected cells and in the detection of oncogenically transformed cells by presenting abnormal intracellular cellular proteins that occur as a result of oncogenic transformation. Class I products also play a central role in transplant recognition and rejection. In each case, MHC class I molecules act as important ligands for the T-cell receptor (TCR) on CD8+ cytotoxic T cells. They may also act as important ligands for a variety of receptors on NK cells. MHC class I-mediated intercellular interactions generally activate the cellular portion of the immune response and result in killing of the antigen-presenting (i.e., virally infected, cancerous, or transplanted) cell expressing the class I molecule.
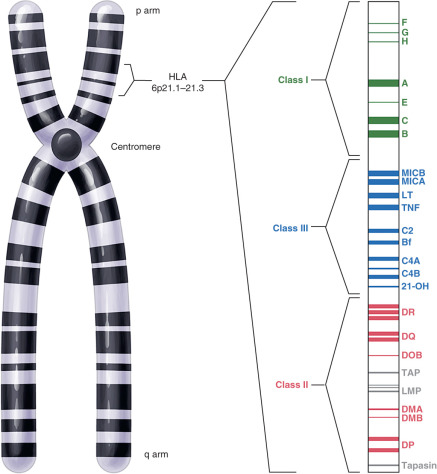
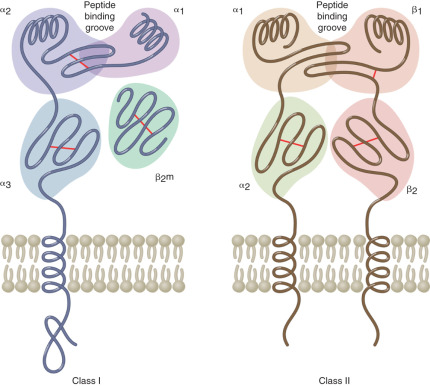
Unlike the globally expressed MHC class I molecules, MHC class II molecules (HLA-DR, HLA-DP, and HLA-DQ) are present on the surface of a limited number of “professional” antigen-presenting cells. These include dendritic cells, macrophages, monocytes, B cells, and tissue specific antigen presenters (e.g., Langerhans cells in the skin). MHC class II molecules are important in the defense against extracellular pathogens, typically bacterial in origin. They present peptides derived from phagocytosed organisms via the endsome/lysosome pathway. The TCR on CD4+ Th cells recognizes MHC class II products loaded with antigenic peptides. Accordingly, MHC class II-mediated interactions with these Th cells are required for licensing B-cell antibody production, as described previously.
The remarkable polymorphism of the classical MHC class I and class II genes allows for the recognition and presentation of an enormous diversity of peptides. In fact, the MHC genes are typically the most polymorphic of all loci in each species. Between the MHC class I (intracellular pathogens/proteins) and class II (extracellular pathogens/proteins) antigen presentation pathways, nearly every protein in the human body can be sampled. Those derived from foreign (nonself-antigens) or altered self are recognized as such, especially when accompanied by costimulation by activated antigen presenting cells. This triggers the development of a cellular immune response. In the absence of an autoimmune disorder, peptides derived from unaltered self do not trigger responses.
A large number of other immune-related genes are located within the HLA locus. These include many of the complement component and cytokine genes (see Fig. 13.5 ). Other MHC molecules are also encoded within the HLA region of chromosome 6. These include nonclassical MHC class I products (HLA-E, -F, and -G) and MHC class I-like products such as MHC class I polypeptide-related sequence A and –B (MICA and MICB). These molecules tend to be less polymorphic than their classical counterparts and are thus predicted to have less important roles in antigen presentation. Nonclassical and MHC class I–related products have been implicated as central players in immune responses at mucosal surfaces, including those within the reproductive tract (see subsequent text).
Lymphocyte Education
It is essential that cells in the immune system can differentiate self from nonself. The education of B lymphocytes in this regard may involve only a single step process—one of negative selection. Those immature B cells that express receptors that recognize self-antigen with high affinity do not survive to exit the bone marrow. This is negative selection against self-antigen and promotes B-cell tolerance to recognition of self-antigens. Self-tolerance by B cells is independent of MHC molecules.
In contrast, T lymphocytes are educated in the thymus via a two-step process. Immature T cells entering the thymus express neither the TCR nor the CD4 or CD8 coreceptors. Upon entering the thymus, all three cell surface molecules come to be expressed. During a process called thymic education, CD4/CD8 double positive cells undergo both positive and negative selection events in which T cells “mature” from CD4/CD8 double positive cells to single positive (CD4+ or CD8+) cells. This two-step maturation positively selects for those T cells with TCRs that recognize self MHC products, thus establishing self-MHC restriction. Those recognizing self MHC class I molecules are destined to become CD8 single positive T cytotoxic/suppressor cells. Those recognizing self MHC class II molecules are destined to mature into CD4 single positive T helper cells. The immature CD4/CD8 double positive thymocytes that do not recognize self MHC (neglect) will die by apoptotic mechanisms. The second portion of the two-step intrathymic T-cell maturation process is one of negative selection. Negative selection of T cells within the thymus appears to depend on the strength of the recognition of MHC/peptide antigen complexes by the TCR of the developing T lymphocyte. If recognition is particularly strong, the cells undergo apoptosis. This functions to eliminate cells reactive against self-peptide antigens, which are likely to be fairly abundant within the antigen-binding clefts of the thymic antigen-presenting cells. T lymphocytes exiting the thymus should therefore recognize self MHC but ignore self-antigen. Growing evidence indicates that NK cells undergo education by receptor “tuning,” possibly at the effector sites.
Mucosal Immune System
Common Mucosal Immune System
The large area of the body’s mucosal surfaces is protected by innate humoral factors and cells and by specific antibodies that are selectively transported into external secretions. These antibodies are predominantly of the IgA isotype. Environmental antigens from ingested food, inhaled particles in air, and resident microorganisms at mucosal surfaces represent the most important sources of exogenous stimulation of the entire immune system. The surface area of the mucosal membranes includes several hundred square meters versus only approximately 1.8 square meters for skin. Large numbers of cells that process antigens, produce antibodies, and produce innate humoral factors such as lysozyme, lactoferrin, peroxidase, and digestive enzymes are present in mucosal tissues and contribute to the protection of mucosal surfaces.
The existence of a common mucosal immune system and the determination of the origin of mucosal IgA plasma cells in humans are based on a number of indirect studies. External secretions of glands anatomically remote from the site of antigen stimulation contain secretory-IgA antibodies that are specific for microbial and food antigens. Ingestion of bacteria and viruses induces a parallel appearance of specific secretory-IgA in saliva, milk, and tears but not in serum. The best known example is that of specific immunity passed on from mother to infant through secretory-IgA contained in breast milk. Specific antibody-forming cells can be transiently detected among peripheral blood mononuclear cells collected 7 to 12 days after oral immunization, and their presence precedes the appearance of specific secretory-IgA antibodies in saliva and tears. Analyses of the properties of IgA from stimulated human peripheral blood mononuclear cells reveal that such cells produce predominately polymeric IgA and display an IgA subclass distribution reminiscent of IgA cells in mucosal tissues.
The common mucosal immune system can be summarized as follows ( Fig. 13.7 ). Various environmental antigens are taken up by specialized epithelial cells (M cells) covering Peyer patches and enter the Peyer patches that contain populations of antigen-processing and antigen-presenting cells. Immature surface IgM+ B cells, presumably of bone marrow origin, become surface IgA+ under the influence of special T cells, Peyer patch dendritic cells, or T-cell–derived cytokines. Such isotype- and antigen-committed cells exit the Peyer patches and, after maturation in mesenteric lymph nodes, enter the blood circulation through the thoracic duct and lodge in the mucosal tissues and glands (primarily the intestine) where they terminally differentiate into IgA-producing plasma cells.
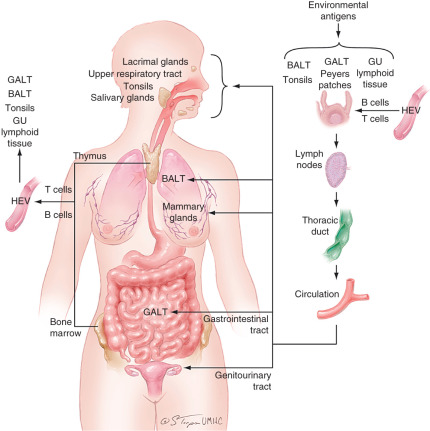
Mucosal Immune System of the Female Reproductive Tract
Although the female reproductive tract is considered an integral component of the mucosal immune system, the genital immune system displays characteristic features that are distinct from those of other typical mucosal sites. Most importantly, the mucosal immune system of the female reproductive tract is under a strong hormonal control that regulates the transport of immunoglobulins, the levels of cytokines, the distribution of various cell populations, and antigen presentation in the genital tissues during the reproductive cycle. In addition to protecting against potentially dangerous infectious agents, it must adapt to a spectrum of physiologic events that include fertilization, implantation, pregnancy, and parturition. Throughout the menstrual cycle, a balance is maintained to respond to the challenges of bacteria, yeast, and viruses without interfering with events that surround conception. During pregnancy, in addition to protecting against infection, the mucosal immune system undergoes further adaption to support a fetal placental unit that is immunologically distinct. To meet these challenges, the reproductive tract has separate compartments consisting of the ovaries, the fallopian tubes, uterus, cervix, and vagina that are precisely regulated by the endocrine system. Each of the sites displays aspects of the mucosal immune system that are designed to contribute to maternal and fetal survival. Unique to the genital tract and unlike other mucosal secretions in which secretory-IgA is the dominant isotype, IgG levels in the lower genital tract secretions equal or exceed the levels of secretory-IgA. Finally, local antigenic stimulation of the lower female genital tract appears to be less effective in the induction of immune responses because of the absence of classical mucosal lymphoepithelial inductive sites such as intestinal Peyer patches ( Fig. 13.8 ).
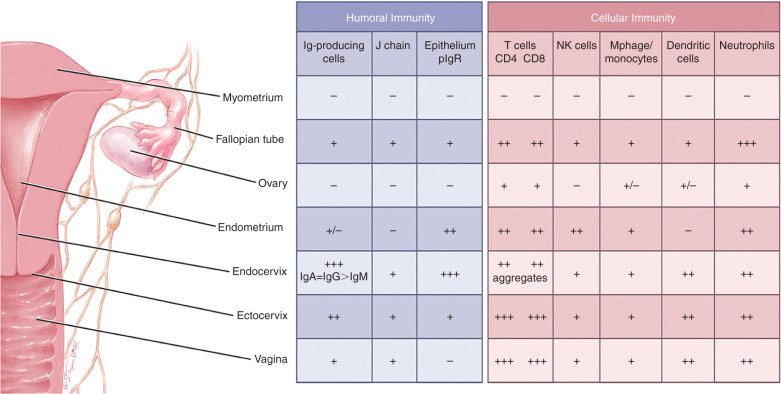
Immunoglobulins in Genital Tract Secretions
Ig concentrations in the genital tract are dependent on hormonal and local factors such as the presence of inflammation. Several studies concerning the origin of antigen specific IgG in the female genital tract secretions suggest that locally produced, as well as plasma-derived, IgG contribute to the IgG pool in genital tract secretions. Regardless of the source, the route of passage of IgG into the lumen of the genital tract—passive, paracellular diffusion or active, receptor-mediated transport—has not been elucidated.
The IgA of cervical mucus is primarily secretory-IgA, with a small component of monomeric IgA. The IgA subclass distribution resembles that of the lower intestinal tract with IgA1 and IgA2 present in roughly equal proportions. The increased proportions of IgA2 may provide a functional advantage to certain specific antibodies, because they are resistant to the IgA1 proteases of many pathogenic bacteria.
Cytokines in Genital Tract Secretions
Unlike other mucosal tissues, the reproductive tract is characterized by progressive tissue growth and remodeling during each menstrual cycle. Cell proliferation, apoptosis, and cell migration are essential for reproductive tract renewal to occur. Growth in preparation for fertilization, implantation, and successful pregnancy is mediated by a changing pattern of cytokine and adhesion molecule expression that is regulated by the sex steroid hormones. Multifunctional cytokines such as Il-1β, IL-6, and IL-10 that are produced throughout the reproductive tract have important roles in the maturation of B lymphocytes to Ig-producing plasma cells. Estrogen increases the expression of polymeric IgA receptor, thereby increasing the transport of IgA into the lumen, when cultured human endometrial cells are incubated with IL-4 and IFN-γ. Regulatory cytokines, such as IL-10, IL-1β, and IL-6 are present in human cervical mucus, and their levels correlate with the cyclic reproductive hormones estradiol and progesterone. Cytokines and chemokines are differentially secreted from immortalized human endocervix–derived epithelial cell lines depending on the hormone milieu in which the cells are exposed.
Cells of the Female Reproductive Tract
Estradiol and progesterone secreted during the menstrual cycle act both directly and indirectly on epithelial cells and other immune cells to modify immune function in a way that is unique to specific sites throughout the female reproductive tract. The female reproductive tract contains a full spectrum of leukocytes with the potential to function in ways similar to those present in other mucosal tissues and in the peripheral blood. Macrophages, distributed primarily between the epithelial and myometrial layer, are found both scattered throughout the fallopian tubes, cervix, vagina, and in small aggregates in the uterus. A tissue-specific population of uterine/decidual CD56 bright CD16 − natural killer (uNK) cells, distinct from peripheral blood CD56 low CD16 + NK cells, are present in uterine endometrium and increase in number during the secretory phase of the menstrual cycle and during the first trimester of pregnancy. They are present at the maternal-fetal interface and have been implicated in cytotrophoblast (cytoT) guidance into the decidua and vascular remodeling necessary for proper decidualization. Similar to other mucosal tissues, it is also now apparent that uNK cells are merely a part of a more complex milieu of ILCs newly discovered in the uterine tissue. Immune cells make up 6 to 20% of the total nonmyometrial cells in the fallopian tube, uterus, endocervix, ectocervix, and vagina and are not significantly influenced by the stage of the menstrual cycle. Approximately 50% of the leukocytes in the reproductive tract are CD3+T cells, with the remainder consisting of neutrophils (CD66b+), B cells (CD19+), and monocytes (CD14+). In particular, NK cells and CD3+ T lymphocytes, including the CD4+ T helper/regulatory cells and CD8+ T cytotoxic cells, are the two major leukocyte subsets present of the endometrial leukocytes. Studies have demonstrated that cervical class I MHC-restricted CD8+ and class II MHC-restricted CD4+ cytotoxic T cells can lyse autologous target cells made to express HIV-1 and other viral proteins.
Discrete lymphoid aggregate (follicles) in the endometrium are composed primarily of a B-cell core (CD19+) surrounded by T cells (CD3+, CD8+, CD4−) and an outer halo of macrophages (CD14+). Antigen-presenting cells in the reproductive tract, which are responsible for initiating the immune response by presenting antigens to T lymphocytes, are derived proportionally from reproductive tract cells and peripheral blood mononuclear cells.
Independence of the Secretory and Systemic Immune Systems
Comparison of the molecular properties of IgA in serum and secretions and of the cells in the secretory tissues and bone marrow suggests that human serum and secretory IgA systems display a great degree of independence ( Table 13.3 ). The molecular properties of IgA produced by the bone marrow (the primary source of serum IgA) and the secretory tissues and glands (the source of secretory IgA) are different in that serum IgA is predominantly monomeric, whereas secretory IgA consists of J-chain-containing polymers. Furthermore, the parallel distribution of IgA subclasses in serum and bone marrow cells, on the one hand, and external secretions and cells in the secretory tissues and glands, on the other, strongly suggest that the two systems are represented by populations of IgA molecules and cells with distinct properties. The ontogenies of the serum and secretory IgA systems display characteristic independent patterns of maturation. Adult levels of IgA are reached in external secretions as early as 1 month after birth but before 2 years of age, while adult levels of serum IgA are not achieved until adolescence.
Characteristic | Serum/Systemic | Secretory/Mucosal |
---|---|---|
Molecular form of IgA | Monomeric IgA | Polymeric IgA |
Subclass of IgA | IgA1 > > IgA2 | IgA1 ≥ IgA2 |
Transport into secretions | No | Yes |
Ontogeny | Matures late | Matures early |
J-chain expression | Mostly negative | Mostly positive |
Secretory component binding | Mostly negative | Mostly positive |
Origin of precursors | Bone marrow | Peyer patches |
Comparison of the levels and molecular properties of the immunoglobulins in blood and in the external secretions of the female genital tract indicate that in humans the systemic and mucosal compartments of the immune system display a considerable degree of independence. In comparison to other components of the common mucosal immune system, the mucosal immune system of the female reproductive tract is characterized by a significant contribution of the systemic compartment with respect to the Ig isotype distribution, unique distribution and phenotypes of B and T cells, strong hormonal dependency, lack of typical lymphoepithelial inductive sites, and relative weakness of immune responses to local infections or immunizations with certain antigens.
Alloimmunity and Reproduction
- ◆
Mechanisms underlying tolerance of the fetal allograft in humans are complex. Maternal immunity is not generally suppressed during pregnancy. In contrast, it is tightly modulated with some responses to the fetus considered inflammatory and others more classically tolerogenic.
- ◆
The uterine endometrium and decidua is populated by a variety of specialized immune cells during pregnancy. While many of these populate other mucosal immune sites and drive innate immune interactions, some, such as uNK cells, are quite unique.
- ◆
Classical immune theory cannot fully explain all of the changes in local immune interactions that occur at the maternal-fetal interface. Other immune approaches that invoke a need for coreceptors or the presence of “danger” signals for appropriate immune responsivity may better explain some aspects of pregnancy immunology.
Fetal Allograft
Maintenance of human pregnancy requires a tightly balanced and cooperative effort between the mother and fetus. Although the concept of the fetal allograft, first posited by Billingham and Medawar nearly 6 decades ago, remains important, the immune interactions between mother and fetus are two-sided and even more complex than can be modeled by a simple allograft. The fetus, which carries maternal and paternal antigens, is more specifically called a semi-allograft but must still circumvent potentially catastrophic alloimmunity. It does this through alterations in antigen presentation and robust secretion of immunoregulatory pregnancy hormones. The mother, in turn, must balance tolerance to paternal alloantigens against a continued need for defense against pathogen invasion. She does this via local alterations in decidual immune cell subpopulations combined with alterations in local and systemic immunoregulatory hormone and chemokine/cytokine secretion.
Cellular and Soluble Mediators of Immune Responses at the Maternal-Fetal Interface
Immune Cell Subtypes
As mentioned previously, the immune cell repertoire of the human endometrium differs dramatically from that of the peripheral blood and is characterized by very few B lymphocytes, a larger complement of T cells, macrophages, ILCs/uNKs, and a number of more specialized immune effector cell subtypes. The relative proportions of these resident cells demonstrate remarkable menstrual cyclicity. A few of these cellular populations deserve specific description, as their presence in both the female reproductive tract and in the periimplantation decidua implicate a particularly important role for innate immune interactions during implantation and early pregnancy.
Peripheral immune cells have been described that have characteristics of both NK cells and T cells. These NKT cells and their ligands (e.g., CD1) have been detected in the endometrium and decidua of humans. NKT cell interactions with the MHC-like antigen presenting molecule, CD1d, can result in proinflammatory or in tolerogenic effector functions, suggesting that these interactions may be important in maintaining the delicate immune balance at the maternal-fetal interface. Other NK-like cells also populate the late luteal phase endometrium and decidua of women. These unusual cells have been variably called decidual granular lymphocytes, large granular lymphocytes (LGLs), and decidual natural killer cells (dNKs), and they exhibit phenotypic differences in their cell surface CD expression from typical NK cells found in the periphery. Still, most investigators consider them to be NK cell variants. The proportion of uNK cells in the decidua of early pregnancy rises to nearly 70% to 90% of the total endometrial lymphocyte population. Recent studies reveal that this lymphocyte mix is even more complex than previously understood as all three ILC groups, in addition to uNKs, were recently discovered at this site. While NK cells can display activating and inhibitory receptors on their cell surfaces, the predominant interactions of those in the decidua involve killer inhibitory receptor (KIR) ligation with trophoblast MHC class I products (see previous text) and the outcome of these interactions is unlikely to be cytotoxic. Rather, the cytokine and chemokine products of the decidual NK cell tend to be tolerogenic and to support trophoblast invasion and decidual spiral artery remodeling. Very elegant investigations have shown that decidual NK cells are derived from a small population of endometrial NK cells that await pregnancy for robust expansion. These cells do not appear to be recruited from the peripheral blood, as previously hypothesized. Thus the measurement of peripheral NK cell number and/or activity as a predictor of pregnancy outcome is unlikely to reflect aberrancy in the function of decidual NK cells in pregnancy maintenance.
Animal and human data have also suggested roles for “suppressor” macrophages and TCR γδ+ T lymphocytes in the immunology of early pregnancy. Mimicking the subcategorization of Th cells, macrophages have been classified based on their cytokine secretion patterns and the abundant macrophages of the uterine decidua typically exhibit an immunosuppressive phenotype or M2 polarization. The M1 phenotype is proinflammatory. In contrast, M2 cells exert robust IL-10 secretion and indoleamine 2,3-dioxygenase (IDO) activity. As mentioned earlier, TCRγδ+ T cells have presumed importance in innate immunity. Their presence within the human endometrium increases dramatically in early pregnancy, they preferentially secrete IL-10 and TGF-β, and they support trophoblast invasion and inhibit trophoblast apoptosis. All should promote pregnancy maintenance.
Soluble Immune Effectors
The human endometrium and decidua are replete with immune and inflammatory cells capable of cytokine and chemokine secretion, but the role of individual factors in pregnancy maintenance may be fairly minimal. Most cytokine genes have been individually deleted in animal models and few, if any, of these factors appear to be absolutely required in pregnancy maintenance. Still, two are frequently discussed as particularly integral. Leukemia inhibitory factor (LIF), and IL-11, both IL-6 family cytokines, have been demonstrated to be required for blastocyst implantation, but not for subsequent embryogenesis in mice, indicating that the defect induced by their deletion is in the maternal compartment. Further delineation of the mechanisms for these implantation abnormalities indicate that LIF functions at the blastocyst attachment/adhesion phase and IL-11 in the response to the implanted blastocyst. The fact that essential roles for individual cytokines in pregnancy maintenance are so limited speaks to the likely redundancy in this system and spurs future studies addressing the integrated effects of multiple cytokines.
Application of the Th1/Th2 paradigm to pregnancy maintenance has led to the hypothesis that the intrauterine environment surrounding normal human pregnancies is Th2-dominant and in vitro data have shown that peripheral blood cells from women with normal pregnancy histories secrete Th2 type cytokines when exposed to placental antigens; some women with histories of RPLs have Th1 responses in the same in vitro assay systems. Inflammatory cytokines characteristic of Th1 responses (e.g., IFN-γ) have been shown to be harmful to the implanting embryo. While the Th1/Th2 paradigm has been useful as a stepping stone in our understanding of the immunology of pregnancy maintenance, rapid expansion in descriptions of cytokine/chemokine activities and in T helper cell subpopulations suggests that the Th1/Th2 paradigm must also be expanded to include Th17, Th3, Treg, and possibly even more cytokine-secreting immune cells at the maternal-fetal interface. Furthermore, the antigen recognized at the site of implantation that might cause dysregulation of the local T helper response phenotype has yet to be identified in vivo. In vitro data have been based on the supposition that the antigen(s) is placental in origin, which is a reasonable, but unverified hypothesis. Cytokine profiles in the microenvironment of an implanting fetus are also difficult to document, and dysregulation in the presence of a failing pregnancy will always be obfuscated by the question of cause versus effect. The possibility that dysregulation of T helper response causes pregnancy loss remains intriguing but unproven.
Antigen Presentation in the Placenta
There is little controversy that the characteristics of antigen presentation in the placenta are unique. For many years, Medawar’s paradox of the fetal allograft appeared consistent with the assumption that the placenta lacked MHC class I and class II transplantation antigens. This assumption, however, was only partially correct. It is true that MHC class II molecules are not expressed on the surface of placental trophoblast cells, nor are the classical MHC class I transplantation antigens HLA-A and -B. However, the classical MHC class I product, HLA-C, and at least two nonclassical products, HLA-G and HLA-E, are found on particular subpopulations of trophoblast cells.
The human placenta, which is of the hemochorial type, is a complex structure that establishes intimate association with maternal tissues primarily through two types of cells: (1) syncytiotrophoblast (SynT) cells, the fetally derived trophoblast cell subset that coats the surface of the placenta villi and is directly bathed in maternal blood, and (2) extravillous trophoblast (EvT), which invade deeply into the maternal decidua, interact directly with maternal decidual immune cells, and remodel the uterine spiral arteries. Both of these trophoblast subsets are derived from an ill-defined, stem-cell like, cytotrophoblast (CytoT) subpopulation ( Fig. 13.9 ). At the surface of placental villi, within the decidua and within decidual spiral arteries, fetally derived trophoblast cells intimately contact maternal immune effector cells, thereby exposing tissues expressing fetal antigens to potential MHC-restricted recognition as nonself.
