Visit the Endocrine Hypertension: From Basic Science to Clinical Practice , First Edition companion web site at: https://www.elsevier.com/books-and-journals/book-companion/9780323961202 .
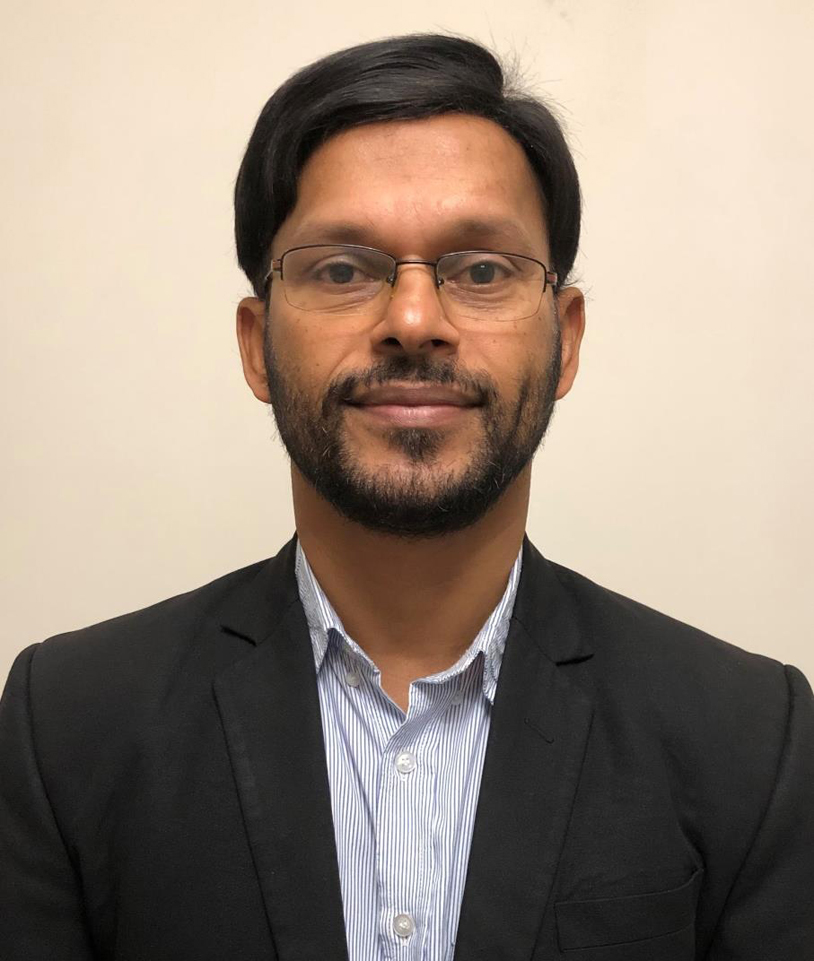
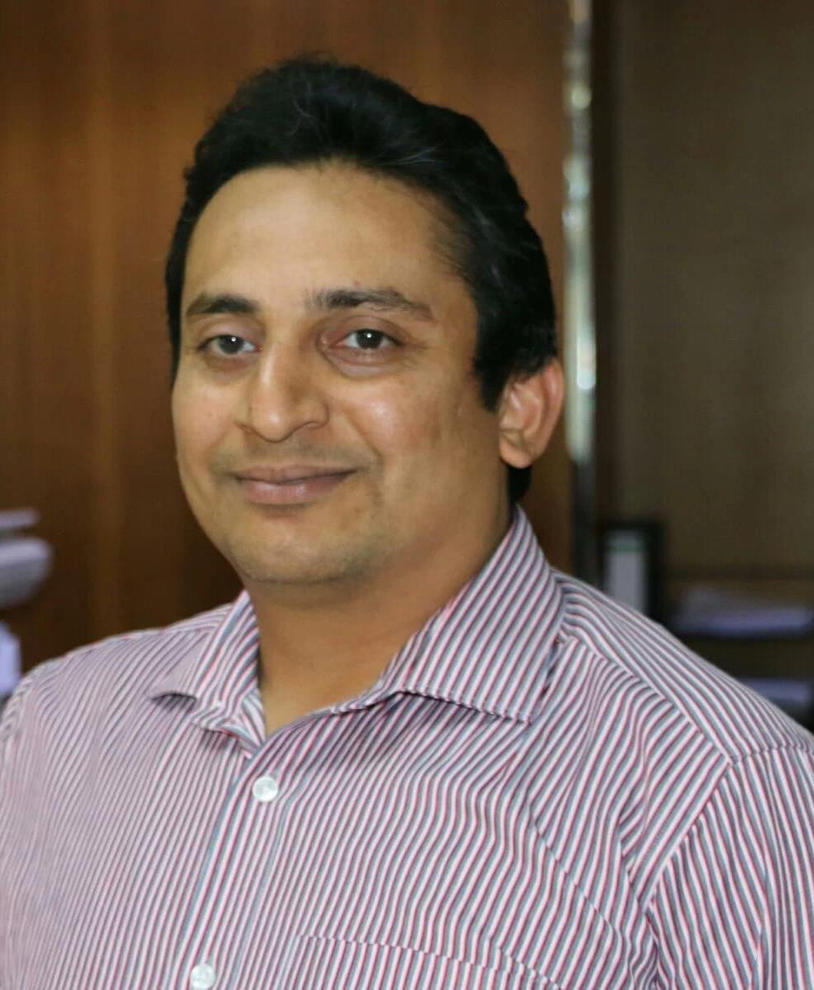
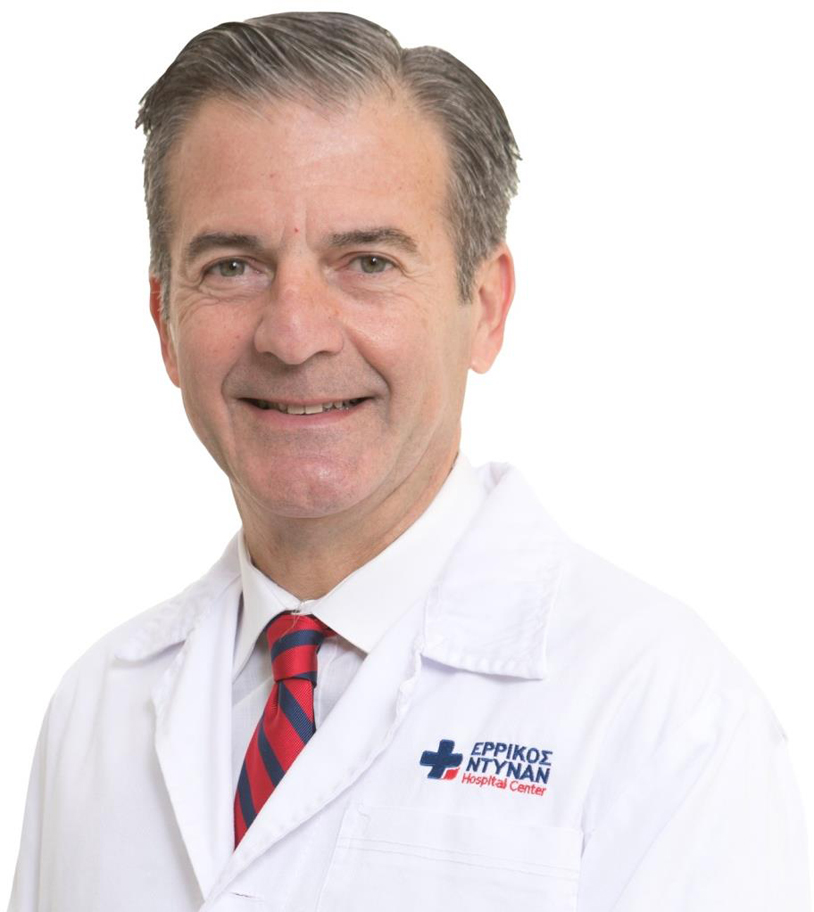
Introduction
The hypothalamic–pituitary–adrenal (HPA) axis is an important neuroendocrine circuit that controls the adaptive stress response and metabolic homeostasis of the human body. The paraventricular nucleus (PVN) of hypothalamus has neurons that express angiotensin Type 1a receptors (PVN Agtr1a ) and is implicated in many neuroendocrine, as well as autonomic adaptive responses [ ]. Arterial blood pressure regulation depends on many of the neuroendocrine and autonomic functions of the HPA axis. The blood pressure response to physiological and pathological stress and its relationship to the output of HPA axis neural circuitry had been an area of immense scientific research in the past few decades [ ]. This chapter evaluates the relationship between HPA axis and blood pressure regulation in health and disease states, and presents the relevant up-to-date evidence.
Physiology of blood pressure regulation and HPA axis
POMC (pro-opiomelanocortin): its role in metabolism and energy balance
Pro-opiomelanocortin is a polypeptide prohormone synthesized by the corticotrophs and melanotrophs of the pituitary gland and from the neurons of the arcuate nucleus of hypothalamus [ ]. POMC neurons play pivotal roles in the control of several physiological functions including feeding behavior and the regulation of energy balance to maintain metabolic homeostasis [ ]. By fine-tuning the divergent metabolic pathways and behaviors necessary for survival, the POMC neuronal circuitry is thought to integrate appetite regulation and whole-body metabolic physiology, and thereby modulate
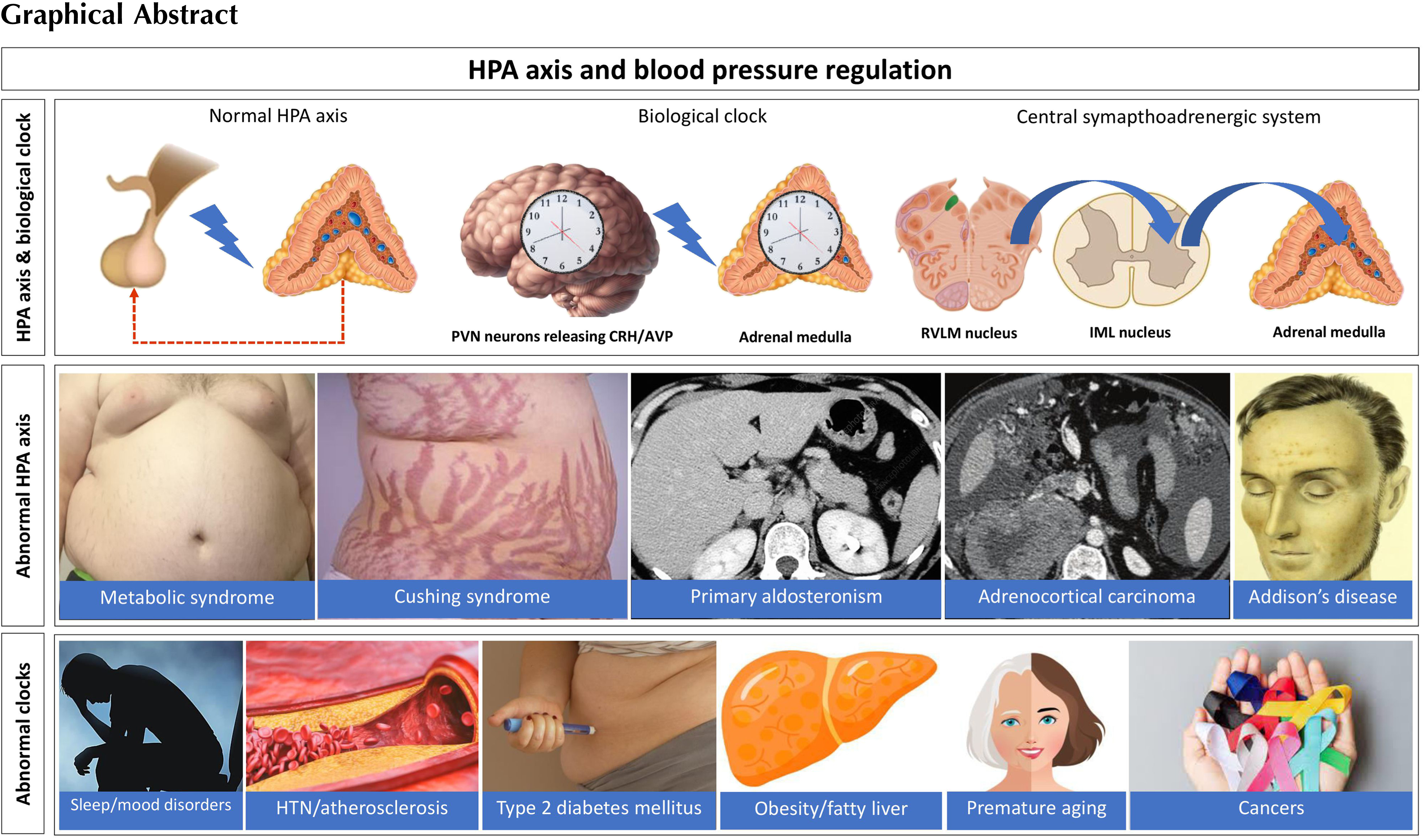
CRH (corticotropin-releasing hormone) and AVP (arginine vasopressin) control the expression of POMC (pro-opiomelanocortin)
Human CRH is a peptide hormone encoded by the CRH gene and secreted by the hypothalamus in response to stressful stimuli [ ]. Several molecules such as interleukin-1 (IL-1), IL-6, tumor necrosis factor-α (TNF-α), serotonin, acetylcholine, histamine, norepinephrine, epinephrine, arginine vasopressin (AVP), angiotensin II, neuropeptide Y (NPY), cholecystokinin, activin, encephalin, estrogens, γ-amino butyric acid, dynorphin, substance P (SP), somatostatin, galanin, and last but not least, glucocorticoids induce expression of CRH gene and therefore the CRH release [ ]. Several pharmacological agents including psychotropic drugs cause CRH secretion. Most of these molecules are formed in the body as part of physical, physiologic, metabolic, and adaptive stress response to prepare the individual in combating such vulnerable situations.
CRH binds to the CRH receptors in various tissues including the pituitary gland and activates various biochemical pathways for additional hormone/neurotransmitter signaling [ ]. Hypothalamic neurons produce CRH and AVP, which are transported by local vascular network to the anterior pituitary. Both these neuropeptides increase the POMC gene transcription and, thus, synthesis of the prohormone that gives rise to ACTH, MSH molecules, and β-lipotropin [ , ]. The neural signals mediated through CRH and AVP during normal physiological state and in various pathophysiological conditions as part of adaptive response modulate the HPA axis function in health and disease.
Pathophysiology of hypertension and HPA axis
Blood pressure (BP) control in the human body depends on a multitude of factors. Significant diurnal variations occur in the BP values in any individual during physical activity and rest to ensure normal perfusion of vital organs and tissues in the body. This inherently programmed vital function is maintained through rigorous fine-tuning during evolution to preserve homeostasis essential for normal life and well-being [ ]. There is a circadian pattern of BP in healthy individuals with a morning surge upon waking that plateaus during the day, and a 10%–20% decrease (‘dip’) in BP during sleep such that average nocturnal BP is lower than the BP in the awake period during the day [ , ]. Around 30% of individuals show a nocturnal dip in BP that is less than 10%, and are considered nondippers. Although the main reason for circadian variations in BP is thought to be the activity of sympathetic nervous system, several other neurohormonal systems including dietary sodium intake, salt sensitivity, impaired sodium excretion capacity during daytime, and HPA axis function might play their roles [ ].
Normal HPA axis function is highly influenced by the physical, environmental, neurohumoral, and emotional milieu of an individual, and any form of stress in these factors can have major impacts in its neurohormonal output. Stress, a state of disharmony in the physiological normality, is counteracted by various physiologic and behavioral responses to reestablish homeostasis termed as adaptive stress response mediated through complex neuroendocrine, cellular, and molecular mechanisms in the central and peripheral nervous systems [ , ]. The key components of the central neurochemical circuitry responsible for the stress system activation are hypothalamic CRH and AVP neurons in combination with the catecholaminergic locus coeruleus [LC] and norepinephrine [NE] neurons, whereas the peripheral limb of this complex circuit is formed by the sympathetic adrenomedullary system controlled by the HPA axis [ ]. There are also multiple negative and positive feedback loops to maintain homeostasis in health. For example, NPY stimulates CRH neurons and inhibits the central sympathetic nervous system [ , ], and thereby influences the central stress system activity in opposite ways.
HPA axis is a key component of the central and peripheral limbs of the stress system, the integrity and precise regulation of the function of which are crucial for successful adaptive response to any form of stress [ , ]. Under normal conditions, the circadian control of HPA axis is maintained by pulsatile release of CRH and AVP [ ]. This orchestrates the HPA axis function according to the sleep-wake cycle in tuning the biologic clock rhythm of a healthy individual. The HPA axis gets activated within minutes of stressful stimuli with the release of CRH from the hypothalamus that stimulates pituitary to release ACTH into the circulation [ ]. ACTH stimulates adrenal cortex to secrete cortisol that elicits negative feedback on the pituitary to stop ACTH release. However, chronic stress can inhibit this negative feedback and contribute to poor health due to diseases and states that are associated with higher cortisol values [ ]. Sometimes medications, addiction, trauma, or chronic stress can cause activation of HPA axis and high cortisol levels, leading to a condition that was previously termed “pseudo-Cushing’s” syndrome, and now is better known as nonneoplastic Cushing’s syndrome (NCS) [ ]. Elevated blood pressure is almost always a feature of NCS, regardless of the degree of activation of the HPA axis or the peripheral cortisol levels. In classical Cushing’s syndrome (CS), hypertension is one of the most common symptoms, typically more severe with higher cortisol levels, and with long-lasting consequences in cardiovascular morbidity even long after the surgical or medical cure of the underlying condition; in patients, with active CS, typically there is an abnormal circadian rhythm of blood pressure with lack of nocturnal dipping [ ].
The stress system is closely connected to and communicates with the biological clock system that controls the circadian rhythm of the body and regulates multiple physiologic bodily functions [ , ]. There is a central master clock situated in the hypothalamus that orchestrates the functions of the peripheral clocks in multiple organs such as adrenal glands. The central clock system is closely linked to the HPA axis [ ]. The circadian cortisol rhythm with morning surge and late evening nadir is maintained by the light-activated central clock system and the HPA axis activity via its central connections mediated through CRH/AVP-neurons [ , ]. Through the connections of the central autonomic nervous system to the adrenal medulla, the local adrenal clock is reset modulating the adrenal sensitivity to ACTH through epinephrine and NPY which also contributes to the circadian glucocorticoid rhythm [ , ]. Through these complex neurohormonal circuitries, HPA axis directly and indirectly contributes to the maintenance of blood pressure especially during stress.
HPA axis and autonomic nervous system (in relation to BP)
The PVN of the hypothalamus possesses several neuroendocrine and autonomic functions and contributes to the control and maintenance of blood pressure through its coordinated neural output [ ]. Within the PVN, angiotensin-II (AT-II) is implicated in orchestrating the neuroendocrine and autonomic responses to stressful stimuli through activation of the AT-II type 1a receptors [ ]. This in turn results in activation of the HPA axis and sympathetic outflow to augment cardiovascular reactivity as a part of the fight-or-flight response during any form of stress [ , ]. The coordinated activity and the novel cross-talks between the HPA axis (along with its neuroendocrine output) with the central autonomic nervous system and its peripheral limb in the splanchnic nervous system through its sympathoadrenal effector organs regulate the blood pressure to a great extent during rest and stressful stimuli. The catecholamines secreted from the peripheral autonomic nervous system controlled by the central autonomic pathways finally modulate the cardiovascular responses to maintain BP in health and during illness. The role of local factors in the vasculature and the effects of catecholamines on blood pressure regulation are detailed in Chapter 2 .
A graphical representation of HPA axis and the central to peripheral sympathoadrenal axes are shown in Fig. 4.1 .
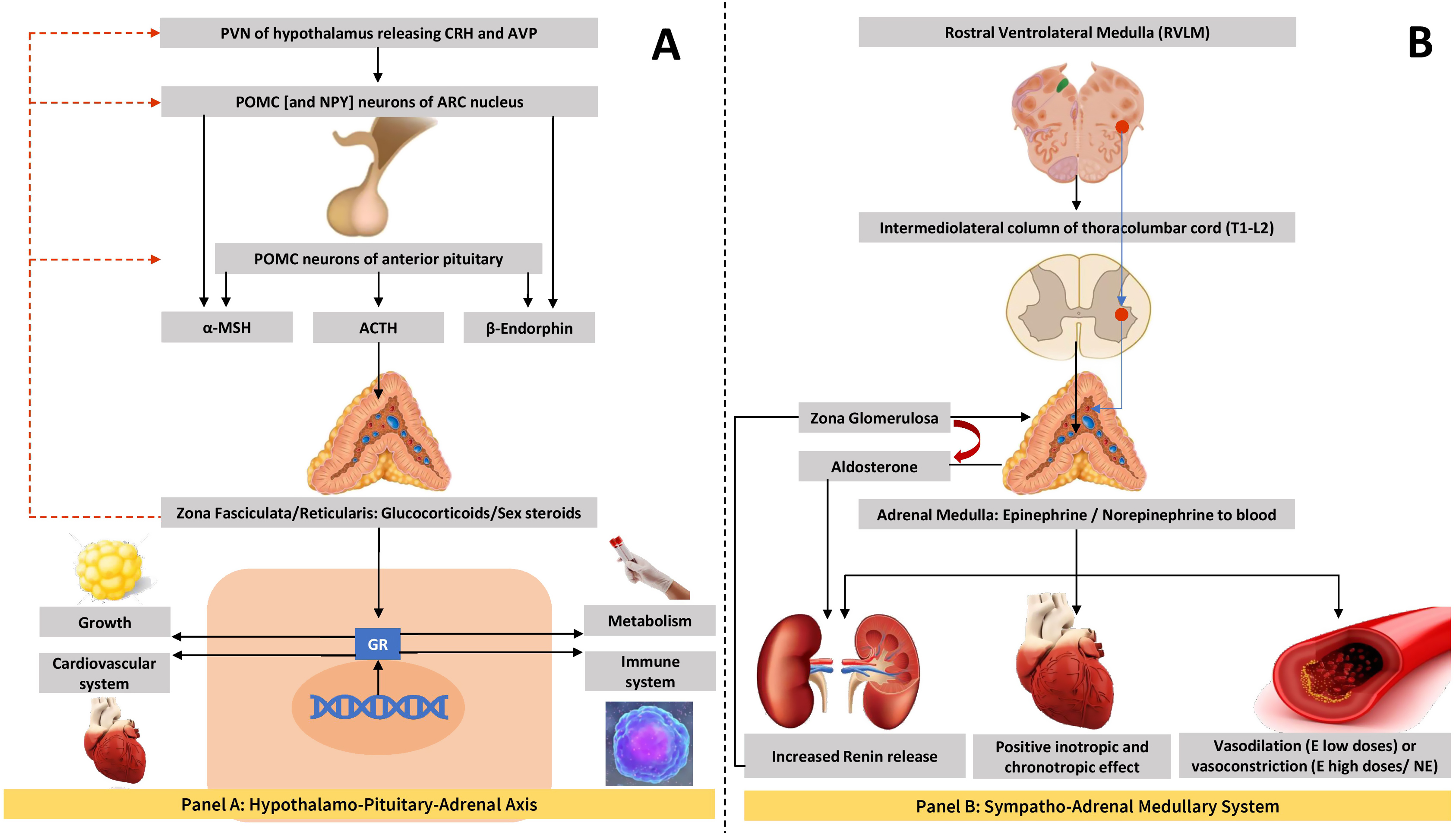
HPA axis, adrenal hormones, and blood pressure
Adrenocortical hormones such as glucocorticoids and androgens are under the direct control of the HPA axis, unlike mineralocorticoids which are largely under the control of the renin–angiotensin–aldosterone system (RAAS) and plasma potassium levels. The principal mineralocorticoid in circulation is aldosterone, the main actions of which are salt and water balance and maintenance of blood pressure in normal levels. The details of blood pressure regulation by mineralocorticoids are given in the Chapter 3, Chapter 6, Chapter 7 and therefore not elaborated here.
Cortisol is the main glucocorticoid in circulation that possesses protean effects in the body especially in the metabolic homeostasis. Cortisol mainly exerts its pressor effect in blood pressure control through the mineralocorticoid receptors (MRs) in the renal tubules, though at physiological plasma levels there is only negligible pressor effect, as this steroid hormone is inactivated by the enzyme 11β-hydroxysteroid-dehydrogenase type 2 (11β-HSD2) [ , ]. In pathological hypercortisolism states such as in CS, this enzyme is overwhelmed which results in overactivation of the MR and consequently the development of hypertension. Other mechanisms of BP abnormalities and hypertension in patients with CS are detailed in Chapter 13, Chapter 14 .
As sex steroids are mainly produced by the gonads, adrenal contribution to the circulating sex hormone pool is minimal. Adrenal androgens are vasoactive substances with testosterone possessing some vasoconstrictive effect [ ] and estrogen causing vasodilatation [ ]. Except in adrenal disorders of androgen overproduction, blood pressure is not affected by the adrenocortical androgens.
HPA axis and metabolic syndrome
As described in the previous sections, HPA axis and the central biological clock have influence on the feeding and emotional behavior of human beings, and therefore, abnormalities in this central neural circuitry can be associated with development of obesity and metabolic syndrome (MetS). Energy metabolism can be altered as a physiological response to stress and conversely the changes in energy availability can affect the physiological response to the stressor by activation of HPA axis and the sympathetic nervous system [ ]. Stress during early life and the related activation of the HPA axis and central autonomic system has been shown to be associated with future risk of development of MetS and other cardiometabolic disorders in adult life [ , ]. Obesity and poorly controlled type 2 diabetes mellitus as consequences of MetS may result in further activation of the HPA axis as discussed earlier and re-establish the vicious circle of further worsening of the problems in the affected individual.
HPA axis, various hormonal disorders, and hypertension
Various hormonal disorders are associated with marked alterations in the physiological and neurohormonal output of the HPA axis. The most profound effects on HPA axis are from CS, either from pituitary pathology or from extrapituitary disease. Cushing’s disease (CD) results from an autonomously functioning corticotroph adenoma that causes hypercortisolemia from chronic adrenal stimulation. CD accounts for ≈80% cases of endogenous CS, while adrenal CS accounts for ≈15–20% of cases, whereas ectopic ACTH production causes up to 1–2% of all cases of CS [ ]. Suppression of pituitary production of ACTH with the disruption of HPA axis is classical of adrenal CS and exogenous hypercortisolism. Prolonged suppression of HPA axis for several months is usual following successful management of adrenal CS (see Chapter 14 ). Regardless of the etiology of CS, hypertension is common among patients and the pathophysiology is detailed in the Chapter 13, Chapter 14, Chapter 3 .
Primary aldosteronism (PA), the most common cause of endocrine hypertension, results from overproduction of mineralocorticoids from the adrenal glands and the common causes are adrenal hyperplasia and aldosterone-producing adenoma. Aldosterone production is partially controlled by ACTH, and therefore, HPA axis plays an important role in PA along with RAAS. ACTH stimulation test is useful to differentiate between some forms of PA. It is also useful during adrenal venous sampling to identify unilateral from bilateral aldosterone excess [ , ].
A subset of incidentally detected adrenal adenomas may turn out to become functional on follow-up with mild autonomous cortisol secretion (ACS; subclinical CS) with variable effects on the HPA axis function. The prevalence estimates of ACS vary widely (5–30%) because of the reporting bias [ ]. An overnight dexamethasone (1 mg) suppression test is the preferred diagnostic screening test to check the integrity of HPA axis in patients with suspected ACS in such situations [ ]. Metabolic complications of chronic steroid excess such as type 2 diabetes mellitus, hypertension, dyslipidemia, osteoporosis, and MetS are the anticipated long-term consequences of ACS, and therefore, excision of the adrenal adenoma may be considered although there is still no clear international consensus on this approach [ , ]. Improvement of hypertension occurred in 66.7% of patients with ACS after surgery, while worsening of hypertension was observed in 80% of those followed up conservatively [ ].
Sex steroid-producing adrenal tumors are often malignant and co-secrete glucocorticoids and therefore present with marked alterations in the HPA axis function and virilization or feminization [ ]. They are rare and often present diagnostic and therapeutic challenges to the clinicians. Hypertension and metabolic complications such as diabetes mellitus are usual in these patients, but the classical features of CS are less common owing to the rapidity with which the clinical progression occurs [ , ]. Prognosis depends on the tumor invasiveness, operability, comorbidities including hypertension and cardiovascular disease, and the Ki67 index [ ]. Because of the rarity of these tumors and marked variations in the clinicopathological features, the statistical figures of prognostic outcomes are not very reliable.
A pictorial representation of CS from different causes is shown in Fig. 4.2 .
