Gastrointestinal factors:
Insufficient oral intake
Malabsorption resulting from chronic pancreatitis, inflammatory bowel disease, celiac disease, cystic fibrosis, Whipple’s disease, radiation enteritis, bariatric surgery
Metabolic factors:
Chronic renal insufficiency (from decreased 1alpha-hydroxylase activity)
Hypoalbuminemia (nephrotic syndrome, protein loosing enteropathy) due to decreased vitamin D binding protein
Hepatic failure
Medication Interactions: Antiepileptic medications, glucocorticoids, HAART, rifampin, ketoconazole, cholestyramine, St John’s wart
Granulomatous diseases and malignancies: sarcoidosis, tuberculosis, lymphomas, histoplasmosis
Obesity (vitamin D is sequestered in fat)
Environmental factors:
decreased sun exposure due to increased distance from the equator, prolonged winter, covering of whole body with clothing, living mostly indoors, occupation or lifestyle
sun avoidance due to concern of skin cancer or risk for skin cancer, e.g., prolonged hospitalization, nursing home, advanced age
Physiology of Vitamin D Synthesis and Metabolism
Vitamin D is a secosteroid hormone which may be produced in the human body from direct exposure of the skin to sunlight (Solar UV-B). This causes photochemical conversion of 7-dehydrocholesterol present in the skin to form previtamin D3, which, in turn, converts rapidly to vitamin D3. The oily fishes such as salmon, sardines and mackerel, egg yolks, and fish oils such as cod liver oil contain vitamin D naturally. Fortified milk, cereal, juice, and yogurt are common dietary sources in North America. The cutaneous synthesis of vitamin D may vary widely in different populations depending on latitude, season, and time of day. Skin pigmentation by melanin is a limiting factor in the cutaneous synthesis of vitamin D. Melanin acts as an effective natural sunscreen and, therefore, increased skin pigment can greatly reduce the solar UV-B mediated cutaneous synthesis of vitamin D3 by as much as 99% [11].
Vitamin D from the skin and diet is metabolized in the liver to form 25(OH)D. The circulating 25(OH)D is metabolized in the kidneys by the enzyme 25 (OH) vitamin D-1α-hydroxylase (1α-hydroxylase) to its active or hormonal form, 1,25-dihydroxyvitamin D [1,25(OH)2D]. The renal production of 1,25(OH)2D is closely regulated by serum parathyroid hormone (PTH) as well as serum calcium and phosphorus [12]. The absorption of renal calcium [13] as well as intestinal calcium and phosphorus is increased in the presence of 1,25(OH)2D [14]. It was previously believed that the 1α-hydroxylase was present only in kidney cells; however, this enzyme has been found in variety of tissues which has led to investigation of the role of vitamin D in all of these extra-renal tissues [15].
Several vitamin D-dependent proteins in the enterocyte are regulated by 1,25(OH)2D to increase intestinal absorption of both calcium and phosphorus. Vitamin D is also involved in bone formation, resorption, and mineralization as well as in maintaining neuromuscular function [12, 14]. Circulating 1,25(OH)2D reduces the serum PTH level directly by negative feedback of parathyroid gland activity and indirectly by increasing serum calcium. It also regulates bone metabolism in part by interacting with the vitamin D receptor (VDR) in osteoblasts to release biochemical signals, leading to formation of mature osteoclasts. The osteoclasts release collagenase and hydrochloric acid to dissolve the matrix and mineral, releasing calcium into the blood [16].
Deficient vitamin D status impairs calcium and phosphorus homeostasis. Although the bone, small intestine, and kidneys are the classic organs responsive to vitamin D, the effects of vitamin D appear to involve several other organ systems. The VDR has been identified in numerous tissues and organs, including those not typically associated with calcium homeostasis and bone metabolism. This suggests that vitamin D may have a role in important biological processes beyond calcium homeostasis [17]. Some of the organs containing the VDR include the heart, vascular smooth muscle, endothelium, stomach, pancreas, brain, skin, gonads, as well as activated T and B lymphocytes, monocytes, and macrophages [18].
Vitamin D Receptor
The VDR is a steroid hormone nuclear receptor that binds to 1,25(OH)2D with high affinity and mediates transcriptional gene regulation. It acts as a ligand-regulated “gene-switch” which regulates the transcription of specific genes, leading to a cascade of events in the cell [19]. This may play a vital role in disease prevention and maintenance of extra-skeletal health. A number of recent studies involving cell culture and VDR knockout mice have suggested that vitamin D plays a critical role in regulating the renin–angiotensin system (RAS) and thus, influencing the regulation of blood pressure [20, 21].
Consequences of Vitamin D Deficiency [24]
Biochemical consequences:
Low serum 25(OH)D
Normal or low serum calcium and phosphorus
High normal or elevated PTH (secondary hyperparathyroidism (HPT))
Normal or elevated serum alkaline phosphatase
Decrease in 24 h urinary calcium excretion
Decrease in calcium and phosphorus absorption from the intestine
Clinical consequences: Exacerbation or increased susceptibility to the following diseases
Infections: Upper respiratory tract infections, tuberculosis
Respiratory: Asthma, reactive airway disease
Metabolic syndrome, increased insulin resistance and type 2 diabetes
Cardiovascular: Systemic hypertension, pregnancy-induced hypertension, pre-eclampsia and eclampsia, increased mortality related to cardiovascular events
Autoimmune diseases: Type 1 diabetes, multiple sclerosis, psoriasis, Crohn’s disease, rheumatoid arthritis
Malignancy: Breast, colon, prostate, pancreas
Musculoskeletal: Muscle pain and weakness (leading to increased risk of fall and fracture), osteoarthritis, osteoporosis, osteomalacia and bone pain, rickets
Racial and Geographical Factors Influencing Vitamin D Nutritional Status
There are various cultural, social, racial, and geographical factors that may influence the vitamin D status. The amount of sunlight exposure is one of the most important factors in determining one’s vitamin D status. A change in season or latitude may have a dramatic effect on the cutaneous production of cholecalciferol. Both systolic and diastolic blood pressures have been shown to be significantly and positively associated with distance from the equator [25, 26]. Above the latitude of approximately 33 °N, vitamin D3 synthesis in the skin becomes very low or nearly absent during most of the Winter. In Edmonton, Canada (52 °N), the photosynthesis of pre-cholecalciferol essentially ceases by mid-October and does not resume until mid-April, thus influencing the serum vitamin D concentration in that population [27]. Covering the entire body with clothing (as customary in women in some religions), use of sunscreen and glass shielding may significantly reduce or completely eliminate the production of vitamin D3 in the skin [28]. Biological factors that inhibit cutaneous vitamin D synthesis and bioavailability include skin pigmentation, medication use, body fat content, fat malabsorption, and age [29]. Increased skin pigmentation can reduce cutaneous vitamin D3 production as much as 99.9%, which may explain, at least in part, the higher prevalence of vitamin D deficiency in the African American population [11]. In addition, ethnicity-specific polymorphisms of the VDR gene and its promoter may influence the end-organ response to vitamin D, including individual calcium absorption.
Epidemiological Evidence of the Relationship Between Vitamin D and Hypertension
Several clinical and epidemiological studies have shown that there may be an association between hypertension and vitamin D status as well as calcium metabolism.
The abnormal calcium metabolism including HPT has been related to the development of hypertension [30]. In the early 1990s, Cooper et al. observed that individuals of African origin residing in the northern regions had higher blood pressure than those residing closer to the equator [31]. Langford and Watson [32] noted that children from rural Mississippi had higher blood pressure and low calcium intake compared to urban children. It has been also found that the intake of calcium in salt-sensitive blacks can reduce blood pressure and cause partial regression of left ventricular hypertrophy [33, 34]. Other investigators have found similar results [35, 36].
Several recent studies conducted by different investigators examining the data from the third National Health and Nutrition Examination Survey (NHANES III) showed an association between the serum 25 (OH)D concentration and blood pressure. In this study population, the serum 25(OH)D concentration was found to be inversely associated with blood pressure, even after adjustment for variables including age, gender, ethnicity, and physical activity. The 25(OH)D levels were considerably lower in women, elderly, racial/ethnic minorities, and persons with obesity, hypertension, and diabetes mellitus [37]. The adjusted prevalence of hypertension in this population was 30% higher in the lowest quartile compared to the highest quartile of serum 25(OH)D.
A recent prospective study investigating the independent association between serum 25(OH)D levels and risk of incident hypertension revealed similar findings [38]. In this study, the multivariable relative risk (RR) of incident hypertension among those with low vitamin D level (serum 25(OH)D levels were <15 ng/mL) was 6.13 in men and 2.67 in women (compared with those whose levels were >30 ng/mL). These findings suggest an association between vitamin D deficiency and the increased risk of hypertension. However, recent meta-analyses of different studies demonstrated mixed results. One meta-analysis of ten studies showed minimal improvement in blood pressure with vitamin D supplementation, whereas another meta-analysis showed 80% increase in the risk of incident hypertension with vitamin D deficiency [39].
Clinical Studies Showing Effect of Vitamin D Supplementation on Blood Pressure
Increasing the serum 25(OH)D concentration in the blood, directly or indirectly, has been shown to reduce blood pressure. Krause et al. demonstrated that exposure of persons to vitamin D producing UV-A three times a week for 6 weeks increased the circulating levels of 25(OH)D by 162% and reduced both systolic and diastolic blood pressure by 6 mm Hg, whereas hypertensive patients exposed to UV-A did not experience any increase in the blood level of 25(OH)D and had no change in their blood pressure (UV-A does not produce vitamin D) [40]. Another randomized, placebo-controlled study showed that 800 IU of vitamin D3 plus 1,200 mg of calcium significantly reduced blood pressure by 9.3% after 8 week, whereas treatment with 1,200 mg of calcium alone reduced blood pressure by only 4.0% (P = 0.02) [41]. Adults with significant vitamin D deficiency have been found to have a 50% higher risk of developing myocardial infarction and they are also more likely to die from these cardiac events [42, 43]. A very recent randomized placebo control pilot study showed a 9% reduction in systolic blood pressure (p < 0.001) after treatment with calcitriol 0.5 μg twice daily for only 2 weeks. The blood pressure returned to its pretreatment level after one week of concluding the calcitriol therapy [44].
Vitamin D Deficiency and Hypertension: Potential Biological Mechanisms
(a)
Regulation of the RAS
The RAS is a regulatory cascade that plays a critical role in the regulation of blood pressure, electrolyte, and plasma volume homeostasis. Inappropriate stimulation of the RAS has been associated with hypertension. Vitamin D is a potent endocrine suppressor of renin biosynthesis to regulate the RAS [21]. Vascular smooth muscle and cardiac myocytes contain VDR and it has been estimated that there may be up to 200 genes regulating cardiovascular physiology influenced by vitamin D [45, 46]. Mice lacking the VDR have elevated the production of renin and angiotensin II, leading to hypertension, cardiac hypertrophy, and increased water intake [20]. These abnormalities can be prevented by treatment with an ACE inhibitor or AT1 receptor antagonist. Vitamin D suppression of renin expression is independent of calcium metabolism, the volume and salt-sensing mechanisms, and the angiotensin II feedback regulation. In normal mice, vitamin D deficiency stimulates renin expression, whereas injection of 1,25-dihydroxyvitamin D3 [1,25(OH)2D3] reduces renin synthesis. In cell cultures, 1,25(OH)2D directly suppresses renin gene transcription by a VDR-dependent mechanism. In a transgenic mouse model with mice over-expressing the human VDR in renin-producing cells, suppression of renin expression by 1,25(OH)2 D in vivo was found to be independent of PTH and calcium [47]. Vitamin D also has anti-inflammatory activity, reducing the production of IL-10 and CRP, and suppression of foam cell formation [45, 46, 48]. In the Framingham Offspring Cohort Study, the risk of developing cardiovascular events was found to be doubled with a low vitamin D level of <15 ng/mL (compared to those with vitamin D level >15 ng/mL) [42]. Thus, vitamin D deficiency may increase the risk of hypertension and CVD by up-regulating the renin–angiotensin–aldosterone system and correcting the deficiency status may be beneficial to cardiovascular health (Fig. 10.1).
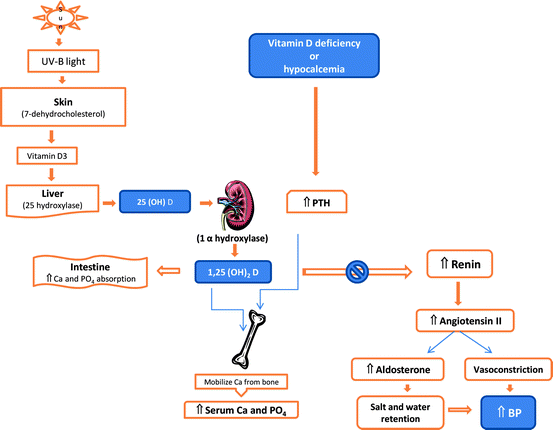
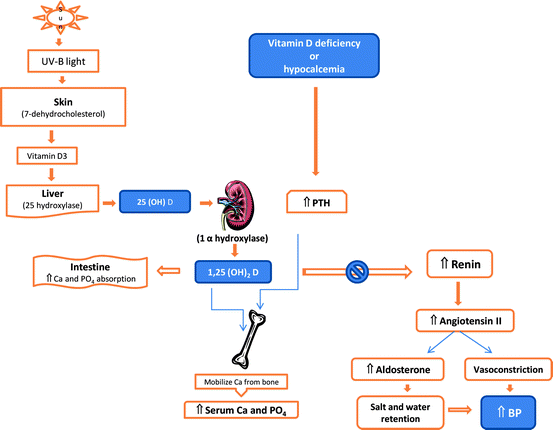
Fig. 10.1
Pathway of vitamin D metabolism and its relationship with PTH and renin–angiotensin–aldosterone system (modified from Ullah et al., Int J Endocrinol, 2010) [98]
(b)
Hypertension, hyperparathyroidism, and vitamin D deficiency
Vitamin D deficiency is frequently seen in patients with primary HPT [49–51]. Hypovitaminosis D itself also causes decreased absorption of calcium from the intestine leading to hypocalcemia which triggers secondary elevation of PTH. Generally, the parathyroid level starts to rise when the serum 25(OH)D concentration drops below 30 ng/mL. Primary HPT with an inappropriately elevated PTH level has been shown to be associated with hypertension (in up to 40% of cases) but the mechanism of developing hypertension has remained controversial (see also chapter by Carrelli & Silverberg in this book). Low vitamin D status is associated with secondary elevation of PTH and increased arterial resistance leading to hypertension [52]. It has also been implicated directly and indirectly to affect cardiovascular risk by increasing susceptibility to arterial calcification [53]. The patients with mild primary HPT have been found to have increased stiffness measured in the radial artery [54]. Elevated PTH level may also contribute to vascular smooth muscle damage and cardiac hypertrophy and predisposition to valvular sclerosis as well as valvular and myocardial calcification [55, 56]. Hypertension generally persists in patients with primary HPT after parathyroidectomy [57]. Elevated PTH from vitamin D deficiency can increase the risk of inflammation with decreased levels of the anti-inflammatory cytokine interleukin-10 (IL-10) which increases after replacement of vitamin D [58, 59]. Increased vitamin D levels are associated with a TH2 shift and lack of vitamin D is proatherogenic because TH1 is stimulated [60–62]. A recent study found the combination of lower serum 25-OHD and higher serum PTH concentrations to be associated independently with sudden cardiac death risk among older adults without CVD disease [63].
Case studies showed improvement or complete resolution of systemic hypertension after parathyroidectomy [64]. In primary HPT with parathyroid adenoma, plasma renin activity and plasma aldosterone levels are higher among hypertensive patients and blood pressure, plasma renin activity, and plasma aldosterone levels normalize after parathyroidectomy [65]. These results are consistent with the hypothesis of a possible direct effect of PTH on renin secretion, which could contribute to the pathogenesis of hypertension.
(c)
Vitamin D deficiency, calcium and endothelial function
Hypovitaminosis D has been shown to have direct effects on the vasculature causing increased vascular resistance [66]. It is also independently associated with increased carotid intima-media thickness [67], decreased arterial compliance, and endothelial dysfunction [68, 69]. Endothelial function (as assessed by flow-mediated dilation) and blood pressure were improved in diabetics after ingestion of only a single dose of vitamin D (100,000 IU) [70]. Elevated serum calcium and PTH levels can independently predict impaired endothelial function [71]. All of these above effects on vasculature may contribute to elevated blood pressure.
(d)
Vitamin D deficiency and cardiovascular mortality
Numerous studies have suggested that vitamin D deficiency is associated with increased mortality from CVDs. Vitamin D deficiency is more prevalent in patients with coronary artery disease. Lee et al. recently published a multicenter study which demonstrated that 96% of patients admitted in the hospital with acute coronary syndrome had inadequate vitamin D levels (<30 ng/mL) [72]. Low levels of serum 25(OH)D are independently associated with cardiovascular and all-cause mortality [73]. An analysis of Framingham study subpopulations showed an increased rate of cardiovascular adverse events (up to 80% higher) among those with vitamin D deficiency (defined in this study as a 25(OH)D < 15 ng/mL) [42]. Analysis of NHANES III study data yielded similar findings of an elevated mortality rate among people with the lowest quartile of vitamin D compared to those with the highest quartile [74]. A systematic review of studies assessing the effect of vitamin D supplementation on CVD events demonstrated a slight but statistically nonsignificant reduction in CVD risk [pooled relative risk, 0.90 (95% CI, 0.77–1.05)] with vitamin D supplementation (approximately 1,000 IU/day) [75]. In a meta-analysis of 18 randomized controlled trials, the intake of ordinary doses of vitamin D supplements (300–2,000 IU/day) was associated with decreases in total all-cause mortality rates (summary relative risk 0.93, 95% CI 0.87–0.99) [43].
Diagnosis of Vitamin D Deficiency
Even though vitamin D assays are now readily available in most laboratories in the USA, the clinicians are often confused about which test to order. Whereas individual immunoassays can easily deviate by 20% from those of LC-MS/MS, there is an outstanding precision between LC-MS/MS and the all method mean/mean of all the methods. LC-MS/MS can quantify vitamin D2 (ergocalciferol) and D3 (cholecalciferol) metabolites separately. This can help in assessing the patient’s compliance with the intake of vitamin D supplements (consider LC-MS/MS if/when patient takes vitamin D2/ergocalciferol). Vitamin D produced cutaneously or ingested orally is quickly converted to 25(OH)D and this is the main storage form in the body. Only a fraction of this 25(OH)D is converted to its active metabolite 1,25(OH)2D and is present in serum in a very low concentration compared to 25(OH)D. In mild to moderate vitamin D deficiency, the serum 1,25(OH)2D may still be normal at the expense of 25(OH)D, which will be invariably low. Therefore, checking the serum 25(OH)D level is the most appropriate test in diagnosing vitamin D deficiency. However, the measurement of 1,25(OH)2D may be useful where there is defect in metabolism of 25(OH)D and phosphate including pseudo vitamin D deficiency rickets, vitamin D resistant rickets, hereditary phosphate losing disorders, malignancy-related osteomalacia, sarcoidosis, and certain lymphomas.
There is no consensus yet as to what concentration of serum 25(OH)D constitutes optimal vitamin D status. Recent evidence suggests that the serum 25(OH)D concentration considered to be optimum for bone health may not be enough for cardiovascular and other extra-skeletal functions. The serum 25(OH)D concentration necessary to prevent rickets and osteomalacia (10–20 ng/mL) is lower than that which suppresses PTH levels (20–30 ng/mL) [76]. This level, in turn, is lower than the level needed to optimize intestinal calcium absorption (34 ng/mL) [77]. Lappe et al. recently reported that 25(OH)D levels of around 40 ng/mL were associated with a significant reduction in the incidence of internal cancers [78]. Finally, neuromuscular performance steadily improves as the serum 25(OH)D level increases and the maximum performance has been associated with a level around 50 ng/mL [79]. Levels for optimal brain development and function are still unclear. Although higher serum 25(OH)D concentrations have been associated with decreased risk of several diseases, these relationships have not been vigorously tested in clinical studies. There have not been many randomized controlled trials demonstrating treatment with vitamin D to achieve higher serum 25(OH)D concentrations improves outcomes. Therefore, these associations should be interpreted with caution. It would seem reasonable to target serum 25(OH)D concentrations above 30 ng/mL for optimal health benefits at least for skeletal health.
Treatment of Vitamin D Deficiency
Vitamin D deficiency can be easily treated with oral vitamin D supplements. However, the problem arises when someone asks: when to treat, what is the optimum regimen, and how long to treat. Based on the current level of evidence, vitamin D deficiency may be defined as a serum 25(OH)D level <20 ng/mL, insufficiency as a 25(OH)D level of 21–29 ng/mL and sufficiency as a 25(OH)D of 30–100 ng/mL [6]. A serum 25(OH)D level of 30–44 ng/mL is believed to be optimal for beneficial outcome for cardiovascular, musculoskeletal health, and malignancies [80]. Achieving a vitamin D level of at least 30 ng/mL has a significant efficacy in reducing the incidence of falls (up to 19%) and fractures in the elderly and the efficacy is highest when vitamin D levels reach 44 ng/mL [81, 82]. Patients with chronic kidney disease (stages 2–5) commonly have poorly controlled hypertension and they also suffer from vitamin D deficiency very frequently [83]. The vitamin D level is an independent predictor of disease progression and mortality in these patients and vitamin D supplementation decreases serum PTH without increasing phosphatemia or calcemia in dialysis patients [84, 85].
As a rule of thumb, serum 25(OH)D can be expected to rise by about 1 ng/mL for every 100 IU of additional vitamin D each day and up to 10,000 IU of vitamin D per day may be taken without significant toxicity for up to 3 months [86, 87]. In a healthy adult, sunbathing for prolonged periods in the summer can produce vitamin D doses equivalent to an oral supplementation of 20,000 IU daily but the serum 25(OH)D level rarely exceeds 100 ng/mL [88]. The risk of hypercalcemia related to vitamin D toxicity in healthy individuals increases when the daily vitamin D intake is > 10,000 IU for a prolonged period or the serum 25(OH)D concentration exceeds 100 ng/mL [80]. Therefore, contrary to common misconceptions, vitamin D supplementation is relatively safe and toxicity is unlikely to develop unless a very high dose is used for a very prolonged period of time.
Vitamin D supplementation at a dose of 1,000–2,000 IU/day may be given empirically without a baseline serum 25(OH)D measurement to those likely to be vitamin D deficient including dark skinned or veiled persons, elderly and institutional subjects living mostly indoors [87, 89–91]. On the other hand, individuals with or at risk of cardiovascular, autoimmune disease and cancer may have their serum 25(OH)D level measured and appropriate treatment regimen should be initiated if the 25(OH)D level is <30 ng/mL. In these individuals, a large loading dose of 50,000 IU once a week may be given for 8–12 weeks, followed by a maintenance dose of 800–2,000 IU/day to prevent dropping of vitamin D level again to the pretreatment level [89–91]. The interval between initiating vitamin D supplementation and rechecking the vitamin D level should be at least 3 months to assess the treatment response and patient compliance.
The Institute of Medicine (IOM) [91] updated its guideline in 2010 regarding recommended daily allowances and tolerable upper limit of intake levels of vitamin D which is believed to be mostly inadequate by most experts. In this recent update, the IOM has concluded that in infants up to 12 months of age, 400 IU/day of vitamin D intake is adequate. In all other age groups, the estimated average requirement has been recommended to be also 400 IU daily. The tolerable upper intake levels have been set at 4,000 IU/day for everyone above age nine and older including pregnant and lactating females. In contrast, the Endocrine Society released Clinical Practice Guidelines on Vitamin D [87] that state the daily requirements for infants and children (up to 8 years) should be between 400 and 1,000 IU and for most adults it should be 1,500–2,000 IU daily. However, to maintain the serum 25(OH)D concentration constantly above 30 ng/mL, most adults (>18 years) will require at least 1,500–2,000 IU of vitamin D supplementation daily. The tolerable upper daily intake amounts have been suggested to be 2,000 IU for infants (0–12 months), 4,000 IU for children and adults up to 18 years and 10,000 IU for most other adults. Pregnancy and lactation requires special attention as the fetus is in utero exclusively dependent on vitamin D sufficiency of the mother. Maternal breast milk contains very little amount of vitamin D and thus, the infants who are exclusively breast fed are at a high risk of developing vitamin D deficiency. Vitamin D fortified formula and the sun exposure are the only ways to maintain vitamin D sufficiency in infants.
Conclusions
Hypertension is one of the most prevalent chronic disease conditions in the modern society. Adequate blood pressure control is the key to prevent complications related to poorly controlled hypertension. In clinical practice, the most common cause of uncontrolled hypertension is the lack of patient compliance. However, there are many instances when blood pressure is still very difficult to bring to/at the desired level despite using a modest regimen of medications with good patient compliance. This is when a clinician should begin to suspect an underlying secondary cause or other contributing causes. Vitamin D deficiency is not commonly considered in this regard. As discussed above, there is plenty of evidence from preclinical, epidemiological studies and early clinical to support a relationship between low vitamin D status and hypertension. The unique and complex interactions between hypovitaminosis D, PTH and calcium make it especially difficult to figure out how much of these effects are truly unique and distinct to vitamin D.
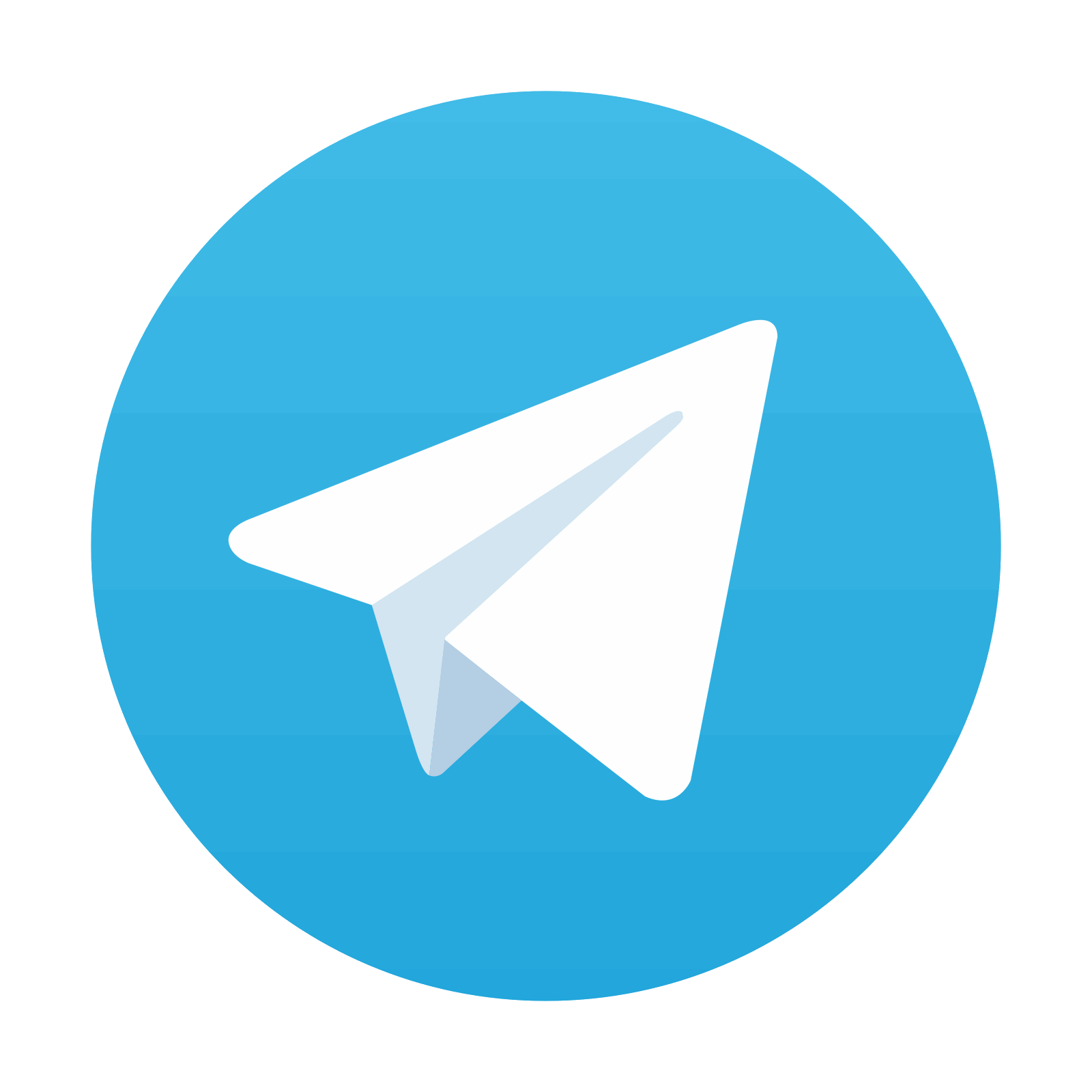
Stay updated, free articles. Join our Telegram channel

Full access? Get Clinical Tree
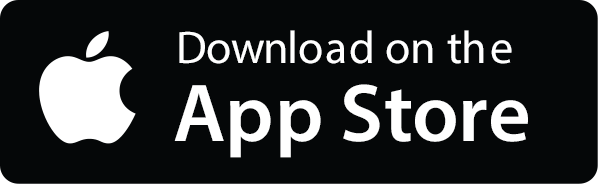
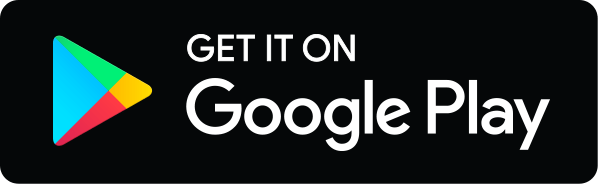