Visit the Endocrine Hypertension: From Basic Science to Clinical Practice , First Edition companion web site at: https://www.elsevier.com/books-and-journals/book-companion/9780323961202 .
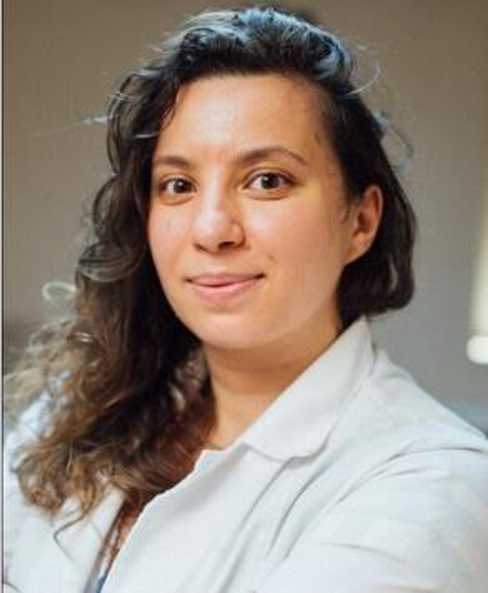
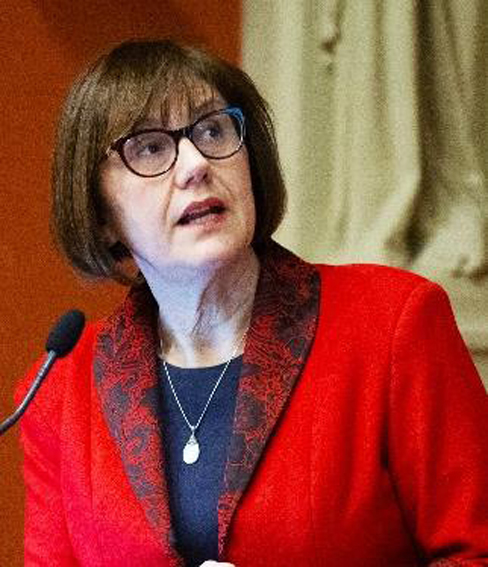
GH and IGF-1 physiological effects
Growth hormone (GH) plays an important role in the postnatal body growth and composition. Several actions of GH are mediated through insulin-like growth factor 1 (IGF-1). The original hypothesis postulated that GH acts on its receptor in the liver to stimulate IGF-1 synthesis which in turn promotes growth by acting on the peripheral tissues [ ]. This concept, however, was later challenged by the data from liver-specific GH receptor of IGF-1 knockout mice, as in these animals the concentration of circulating IGF-1 in the serum was drastically reduced, but body growth was close to normal suggesting that locally produced IGF-1 rather than circulating (mostly liver-derived) IGF-1 determines body growth [ , ]. In this review, we summarize the effects of GH on blood pressure (BP), with more concentration on states with GH excess or deficiency.
GH and IGF-1 in the blood vessels
The vascular endothelium covers the internal surface of blood vessels and has the ability to control vascular tone by releasing vasoconstrictors or vasodilatory agents such as nitric oxide (NO) and prostacyclin [ ]. GH infusion in the brachial artery has been shown to acutely increase the blood flow in the forearm and to lower the peripheral vascular resistance in healthy subjects [ ]. This vasodilatory effect was paralleled by an augmented forearm NO release. As circulating IGF-1 did not change, the vasodilatory effect could be due to GH per se, or due to local IGF-1 production. Similarly, intravascular IGF-1 infusion led to an increased response in forearm blood flow [ ] through a NO-dependent mechanism [ ]. Indirect evidence from animal studies points that both GH and IGF-1 can stimulate NO synthesis by regulating the expression of endothelial nitric oxide synthase (eNOS), the enzyme required for NO production in the endothelium [ ].
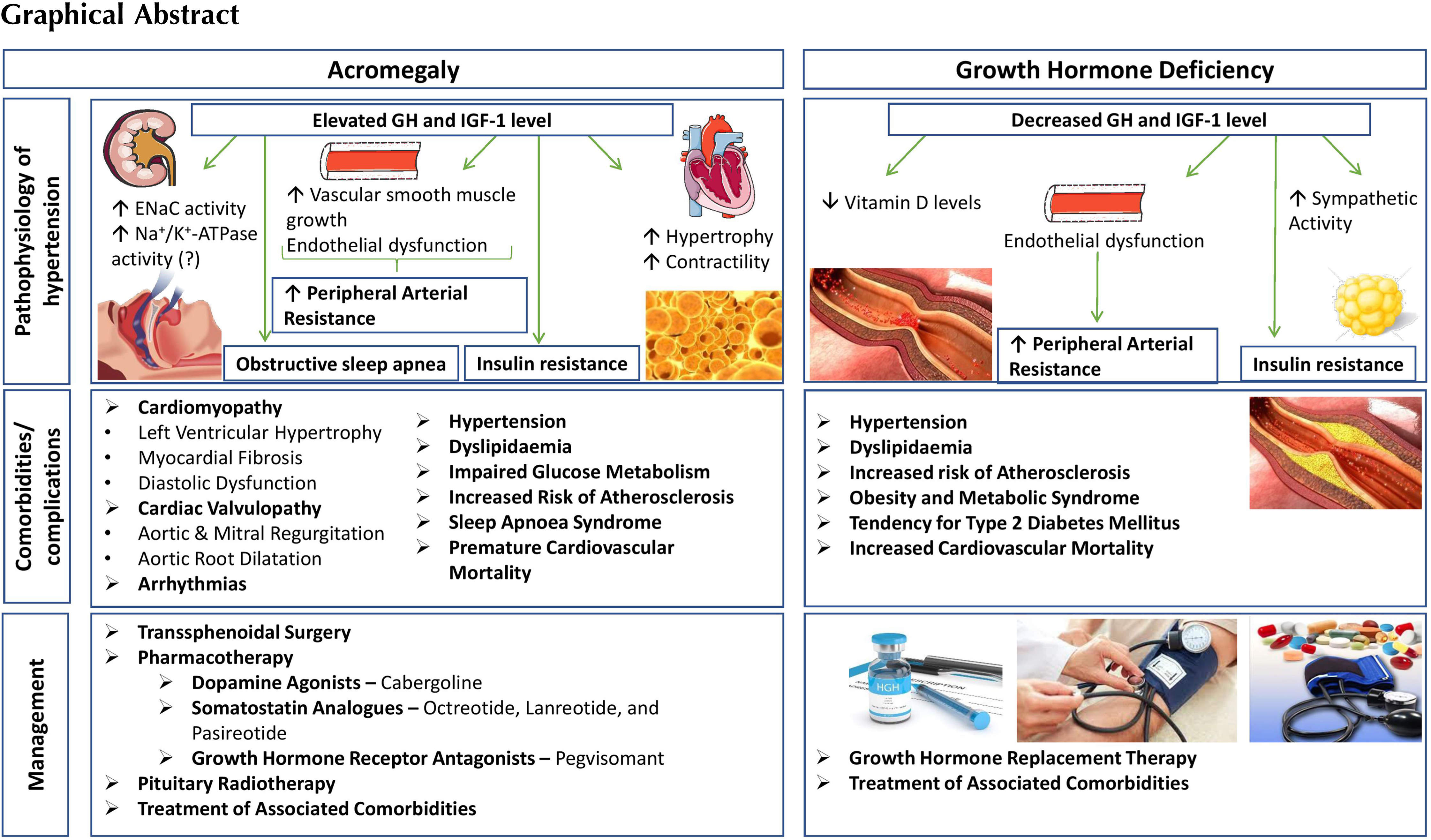
Decreased bioavailability of NO and subsequent increased vascular resistance can be encountered both in GH excess and GH deficiency (GHD). GH/IGF-1 may exert endothelium-independent vasodilatory effects by upregulating gene expression of the vascular smooth muscle ATP-sensitive potassium channel (KATP) [ ], or by enhancing vascular smooth muscle sodium-pump (Na + /K + -ATPase) activity [ ]. The main effects of GH and IGF-1 on blood vessels are summarized in Fig. 15.1 .
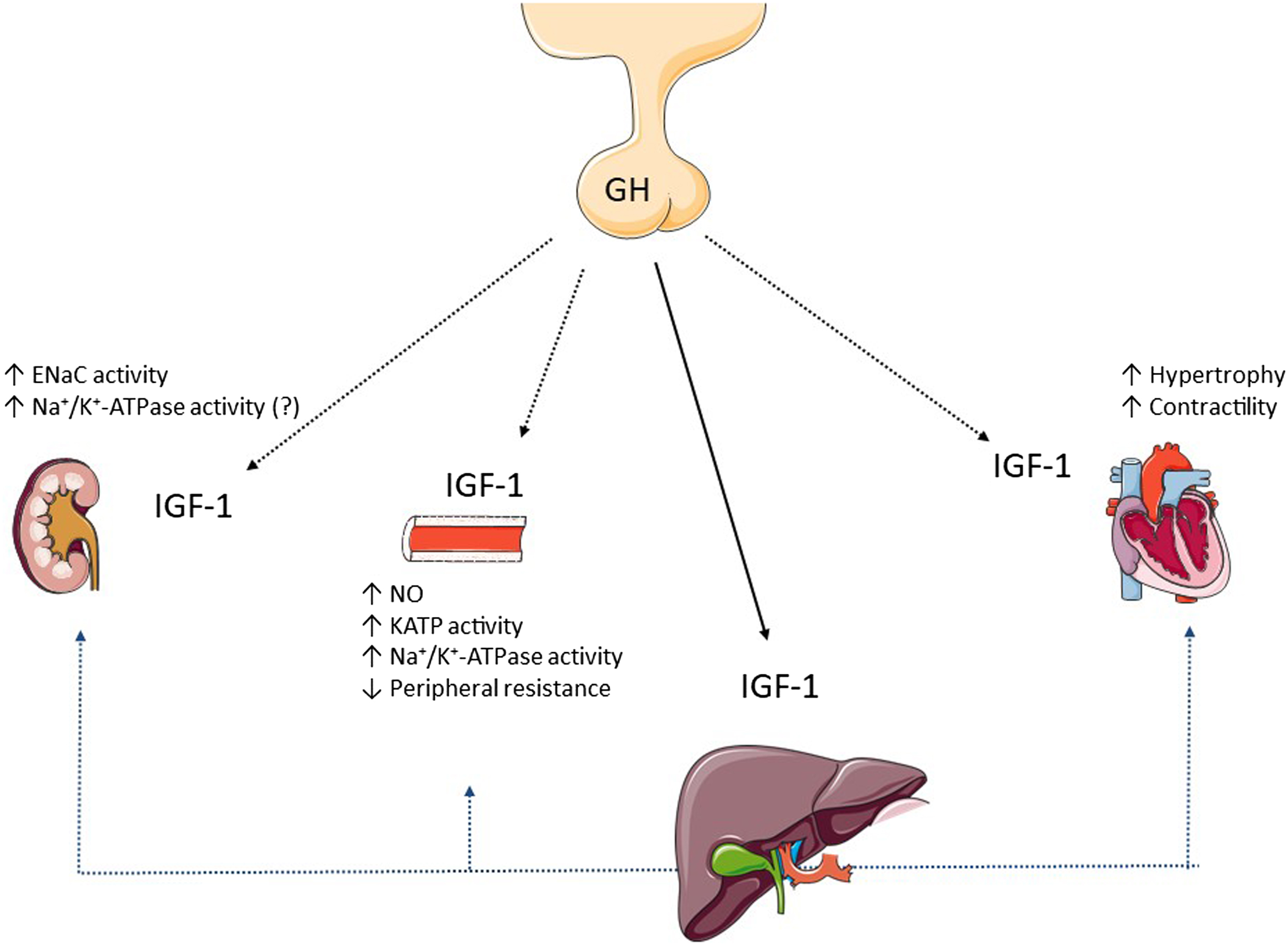
In addition to the vasodilatory effect, GH and IGF-1 have mitogenic activity in the vascular cells since they can induce proliferation of the endothelial cells [ ] and vascular smooth muscle cells [ ]. This aspect may play a role in acromegaly-related hypertension.
The role of GH and IGF-1 in the myocardium
In vitro studies showed that IGF-1 exerts a physiological hypertrophic effect on cardiomyocytes via enhancing the gene transcription and protein synthesis for cardiac and skeletal muscle-specific proteins [ ]. IGF-1 also has calcium-dependent positive inotropic effect on cardiomyocytes, resulting in increased contractility. The proposed mechanisms underlying the increased contractility include modulation of membrane channel activity [ ], enhancement of myofilament sensitivity to calcium [ ], or by upregulating the sarcoplasmic reticulum calcium-ATPase [ ]. The main effects of GH and IGF-1 in the myocardium are summarized in Fig. 15.1 .
The role of GH and IGF-1 in the kidney
The kidney plays a significant role in the regulation of body fluid homeostasis and BP by adjusting the sodium and water excretion depending on the dietary intake [ ]. The main effects of GH and IGF-1 in the kidneys are summarized in Fig. 15.1 . Short-term treatment with GH in human subjects provided the direct evidence for its effects on the kidney: (1) an increased glomerular filtration rate (GFR) and renal plasma flow; (2) sodium retention, (3) phosphate retention, and (4) hypercalciuria [ ]. A decrease in the arteriolar resistance is followed by an increased glomerular perfusion and consequently an increased GFR. The effect on the vascular resistance requires IGF-1-related NO and prostaglandin release [ ]. Data suggest that the sodium retention effect of GH/IGF-1 in the kidney is achieved by stimulating the amiloride-sensitive epithelial sodium channel (ENaC) [ ] in the aldosterone‐sensitive distal nephron (the distal convoluted tubule, connecting tubule, and collecting duct) [ ]. Renal effects of both IGF-1 [ , ] and GH [ , ] suggest a co-operative model between GH and IGF-1 to explain the sodium-retaining effect in the distal nephron [ ]. In addition to the increased ENaC activity, enhanced Na + /K + -ATPase activity in the collecting duct cells may represent another mechanism responsible for the increased sodium reabsorption in the distal nephron [ ]; however, the role of GH/IGF-1 on the Na + /K + -ATPase pump in the sodium-retaining effect remains yet to be fully elucidated [ , ]. GH/IGF-1 also modulates the phosphate and calcium metabolism in the kidney. IGF-1 stimulates the phosphate reabsorption via increased expression and activity of the sodium-dependent phosphate transport protein 2A (NaPi-2a) in the proximal tubule [ ]. Studies on animal models and human subjects suggest that GH (either directly or indirectly through IGF-1) increases the circulating levels of calcitriol (bioactive vitamin D) by stimulating 1-alpha hydroxylase, the enzyme responsible for calcitriol production in the kidney [ ]. The hypercalciuric effect observed after GH treatment is rather transient [ ]; however, in acromegaly, enhanced intestinal calcium absorption secondary to increased calcitriol synthesis is linked to absorptive hypercalciuria [ ].
Acromegaly and hypertension
Epidemiology
The global prevalence of hypertension in adults aged 30–79 years is 32% in women and 34% in men [ ]. Acromegaly, on the other hand, has a prevalence between 2.8 and 13.7 cases per 100,000 people with an annual incidence between 0.2 and 1.1 cases/100,000 people [ ]. While hypertension is a cardinal feature in acromegaly, it only represents a negligible percent of the global hypertension burden.
Hypertension is the most frequent cardiovascular comorbidity accompanying acromegaly [ ]. The prevalence of hypertension in patients with acromegaly is ranging between 11% and 57% ( Table 15.1 ) [ , , ]. Results from a recent large international study (ACROSTUDY, a global noninterventional surveillance study of long-term treatment with pegvisomant) showed that 56% of patients with acromegaly had hypertension at study entry and the percentage increased up to 64% during the follow-up period [ ]. However, the prevalence in this cohort is not representative of the true disease burden because it includes cases of uncontrolled acromegaly with a longer disease duration, as pegvisomant is generally used in patients who failed to achieve disease control after surgery and somatostatin analogue treatment [ ].
Number of studies analyzed | Publication period for the included studies | Hypertension prevalence | Publication year for the study analysis | Reference |
---|---|---|---|---|
18 | 1962–2001 | 18.0%–57.0% | 2001 | [ ] |
19 | 1987–2002 | 17.5%–57.0% | 2004 | [ ] |
14 | 2004–2017 | 11.0%–54.7% | 2019 | [ ] |
This wide range in the prevalence might be the result of different criteria used to define hypertension over different periods of recruitment, or because of different clinical characteristics of the selected patients [ ]. In addition, the majority of the aforementioned studies did not use ambulatory blood pressure monitoring (ABPM) for the assessment of hypertension, as this was reported only in three studies out of 19 in a meta-analysis [ ]. This is relevant as clinic-based BP measurements can overestimate the actual frequency of hypertension in patients with acromegaly compared with ABPM: 42.5% with office BP assessment versus 17.5% with ABPM in a study from 40 patients or 32.4% with office BP measurement versus 23.9% ABPM among 37 patients [ , ]. In a 10-year long retrospective observational study of 205 newly diagnosed patients with acromegaly and 410 age- and sex-matched controls, patients with acromegaly had 1.67-fold and 1.58-fold higher risk to develop mild and severe hypertension, respectively compared to the general population. Moreover, the risk was extremely high for patients with a diagnostic delay of more than 10 years from the onset of the disease [ ]. In acromegaly, hypertension occurs a decade earlier compared to the general population [ ]. The prevalence of hypertension increases with age, as nearly 50% of the patients with acromegaly older than 55 years were hypertensive, according to the data from an Italian registry ( n = 1512) [ ].
Pathophysiology of hypertension in acromegaly
Hypertension in acromegaly is considered to be multifactorial, but exact mechanisms are unclear. Proposed mechanisms ( Fig. 15.2 ) include increased plasma volume due to the fluid retention [ ], and increased vascular resistance due to vascular remodeling, and endothelial dysfunction [ ]. In addition, sleep apnea, insulin resistance, and increased cardiac output may contribute to the pathophysiology of hypertension [ ]. The way each factor contributes to hypertension is detailed below.
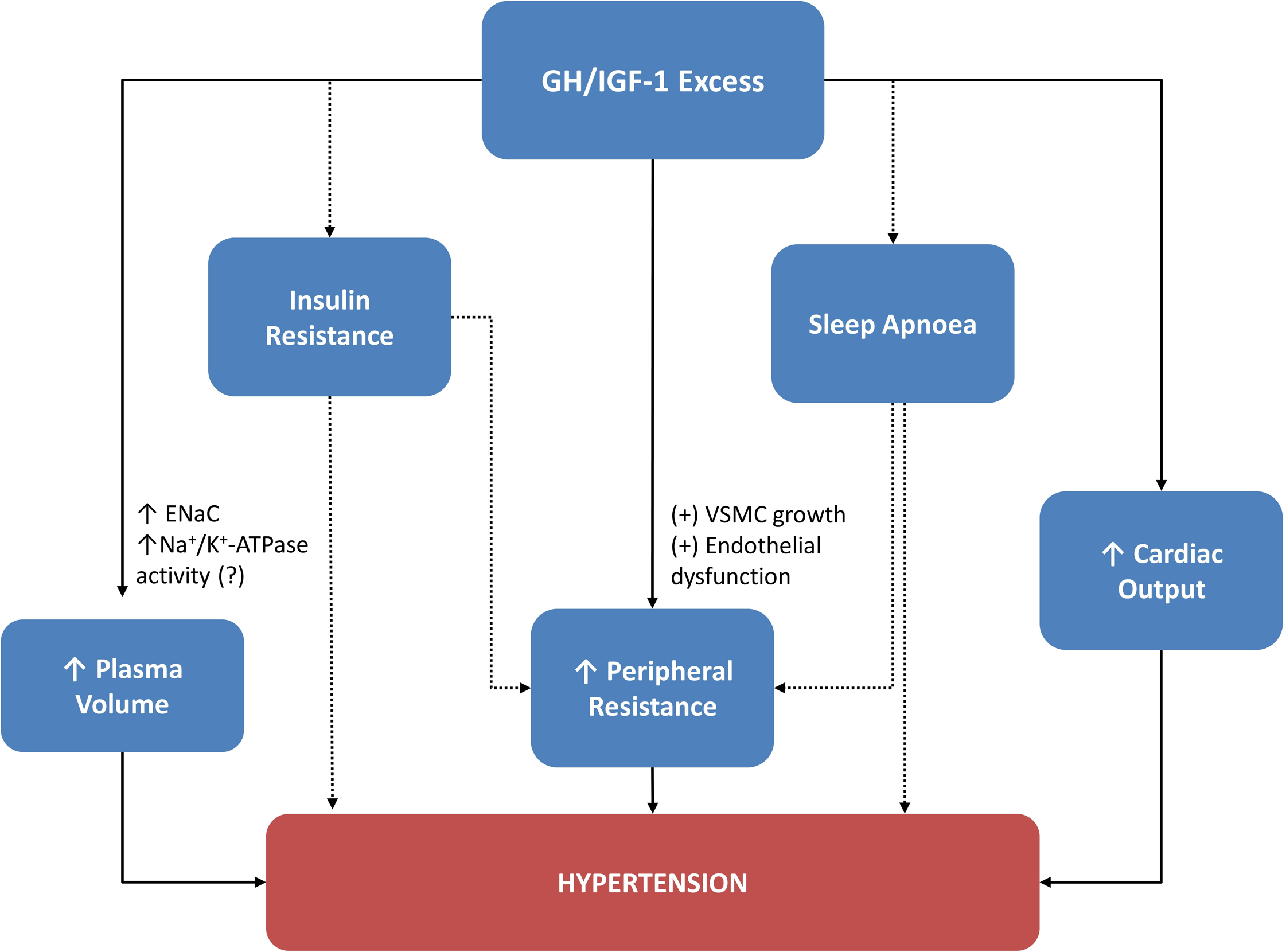
Plasma volume expansion
Sodium-retaining effect and the role of ENaC in acromegaly
In a set of elegant studies, Kamenický’s group established that enhanced action of ENaC is responsible for the sodium and water retention seen in acromegaly. Because it was suggested previously that the Na + -K + -2Cl – (NKCC2) cotransporter located in the thick ascending limb of Henle’s loop is indirectly activated by GH [ ], they evaluated the natriuretic and kaliuretic responses to furosemide (the drug that blocks NKCC2) or to amiloride (the drug that blocks ENaC) before and after treatment of acromegaly in 16 patients on a high-sodium diet. They observed that the urinary Na/K ratio was higher after amiloride and lower after furosemide in patients before treatment of acromegaly. By blocking NKCC2 with furosemide there is a higher amount of sodium delivered to the distal nephron, where an enhanced ENaC retains sodium (and water in response to the osmotic gradient created by sodium) and secretes potassium. Therefore, the urinary Na/K is lower after furosemide. In contrast, by blocking an active ENaC with amiloride, a potassium-sparing diuretic, potassium is preserved while sodium is lost in the urine, meaning a higher urinary Na/K ratio. Successful treatment of acromegaly reduced the effect of amiloride and enhanced the effect of furosemide on the urinary Na/K ratio. The stable natriuretic response after furosemide in acromegaly before and after treatment suggests that NKCC2 does not mediate sodium reabsorption with GH excess. In contrast, it appears that enhanced ENaC is responsible for the sodium and water retention effect seen in acromegaly [ ].
Overview of other possible mechanisms for enhanced fluid retention in acromegaly
Insulin can stimulate the sodium reabsorption through ENaC, but to be able to obtain the same effect as that of IGF-1 on the sodium transport in murine collecting duct-derived cells, insulin concentration had to be 50 times higher than IGF-1. This effect was achieved through the IGF-1 receptor (IGF-1R), as insulin can bind IGF-1R but with a lower affinity [ ].
The amiloride-sensitive ENaC is also positively modulated by aldosterone [ ]; therefore, an indirect antinatriuretic effect for GH excess through activation of renin–angiotensin–aldosterone system (RAAS) has been suggested [ , ]. This hypothesis remains controversial. Early results from GH treatment in adrenalectomized rats showed that the sodium-retaining effect after GH treatment was preserved, suggesting an aldosterone independent effect [ ]. On the other hand, by blocking RAAS, the fluid retention effect was prevented after GH treatment in human subjects [ ]. In patients with acromegaly, plasma aldosterone was increased which significantly dropped after successful treatment, while plasma renin was unaffected [ ]. In contrast, in another study, RAAS was partially supressed in patients with acromegaly on a high sodium diet and did not change after acromegaly treatment. Therefore, RAAS was not responsible for the antinatriuretic effect observed in GH/IGF-1 excess.
An inadequate response of natriuretic peptides to sodium retention and hypervolemia was suggested to be one of the factors responsible for hypertension in acromegaly [ ]; however, plasma atrial natriuretic peptide was normal in patients with acromegaly compared to controls [ ], and plasma brain natriuretic peptide did not change before and after acromegaly treatment [ ].
Sympathetic nervous system activity is commonly increased in hypertension and involves sodium and water retention as well. But there is controversy over the role of adrenergic system in acromegaly [ , ]. Plasma catecholamine concentrations [ ] and the mean 24-h plasma catecholamine levels did not differ between patients with acromegaly and controls [ ]. Normally, BP has a circadian variability, and there is a nocturnal dip. Subjects who have a blunted nocturnal dip are called nondippers and this pattern reflects deficient mechanisms to regulate BP [ ]. Patients with acromegaly manifest a nondipper behavior [ , ]. In spite of the fact that plasma catecholamine levels did not differ between patients and controls, a normal circadian rhythm of catecholamine levels and BP was observed only in cured patients, which might suggest an impaired sympathetic activity in GH excess. In addition, one of the mechanisms responsible for hypertension is sleep apnea, which is common in acromegaly, associated with an increased adrenergic tone [ ]; however, further studies are needed for a firm conclusion regarding the sympathetic overactivity among patients with acromegaly.
Finally, another possible player in the sodium-retaining effect of chronic GH excess might be the sodium pump Na + /K + -ATPase in the distal nephron. Enhanced Na + /K + -ATPase activity was found in leukocytes and skeletal muscles of patients with acromegaly, but its activity in the kidney remains unclear [ ]. The main mechanisms related to hypertension in acromegaly are summarized in Fig. 15.2 .
Increased peripheral vascular resistance
Small arteries and arterioles are the main determinants of peripheral resistance and play an important role in the pathophysiology of hypertension. Hypertension is associated with increased wall-to-lumen ratio in these arterial segments resulting from vascular (eutrophic or hypertrophic) remodeling [ ]. The increased wall-to-lumen ratio is a marker of vascular remodeling.
Vascular remodeling of the resistance arteries
Smooth muscle cells play an important role in the remodeling process of vascular structures, and GH may have mitogenic activity on these cells either directly or through IGF-1 [ ]. Vascular remodeling in the small arteries and arterioles narrow the lumen of the vessels and even minor changes in the lumen of these arteries significantly increase the peripheral resistance. In essential hypertension, there are two types of vascular remodeling: eutrophic and hypertrophic. In eutrophic remodeling, vascular smooth muscle cells rearrange around a smaller lumen without an actual hypertrophy or hyperplasia, while in hypertrophic remodeling vascular smooth muscle cells show hypertrophy [ ]. Using pressure myography, the vascular structure of subcutaneous small arteries was studied in patients with essential hypertension ( n = 12) and acromegaly ( n = 9). Hypertrophic remodeling and greater wall thickening were seen in patients with acromegaly compared to those with essential hypertension, who had eutrophic remodeling. IGF-1 levels in acromegaly were correlated to the wall-to-lumen ratio suggesting that the hypertrophic remodeling is probably due to the growth-promoting properties of GH or IGF-I [ ]. Similar structural changes were described in 41 patients (18 with active acromegaly and 23 in remission) and additional impaired endothelial-dependent relaxation was also reported. Successful treatment of acromegaly significantly improved the vascular structure and function, although without fully returning to normal [ ].
More recently, retinal imaging in 60 patients (19 with active disease vs. 41 with controlled disease) and 60 controls found that the wall-to-lumen ratio was increased in active acromegaly, while in controlled disease the ratio did not differ from controls. The vascular pattern described was eutrophic remodeling. Possible explanations for this discrepancy in vascular pattern include different vascular territories and techniques used for exploration. The lack of hypertrophy in their cohorts may be attributable to a reversible process in the treatment-stabilized patients [ ]. Although the precise pattern involved in the vascular remodeling is not clear, there is a positive relationship between the wall-to-lumen ratio and plasma IGF-1 level, suggesting that an active disease influences vascular structure, but acromegaly induced remodeling may be (partially or totally) reversible if IGF-1 is normalized [ , , ].
Endothelial dysfunction
The NO pathway regulates the peripheral resistance, and it has been shown that patients with essential hypertension have an altered endothelium-dependent vasodilation and reduced NO [ ]. The same hypothesis was suggested to be applicable in acromegaly [ , ]. This is an interesting hypothesis because GH and IGF-1 at physiological levels stimulate endothelial NO production, as we explained before ( Fig. 15.1 ). However, comparing cutaneous vasoreactivity responses in patients with active acromegaly ( n = 10) and normotensive subjects ( n = 10), it was confirmed that endothelium-dependent vasodilatation mediated through NO was impaired in acromegaly [ ], while in another study NO concentrations inversely correlated with GH and IGF‐1 levels and disease duration, probably due to reduced expression of eNOS protein [ ]. Possible mechanism to explain a reduced NO includes increased levels of oxidative stress or the presence of asymmetric dimethylarginine (ADMA), an endogenous eNOS inhibitor. Overproduction of reactive oxygen species by mitochondria diminishes NO by transforming it into peroxynitrite which is a toxic compound for endothelial cells [ ]. In fact, acromegaly has been associated with increased levels of oxidative stress and endothelial dysfunction [ ]. Endogenous ADMA, on the other hand, inhibits eNOS and, therefore, reduces NO formation. Patients with acromegaly ( n = 13) compared to matched controls ( n = 12) had impaired endothelial function assessed by flow-mediated dilatation of the brachial artery [ ], a method used to reflect NO production. ADMA levels did not differ between patients with acromegaly and matched controls, but there was a positive correlation between ADMA and arterial stiffness and negative correlation with small vessel compliance, suggesting a harmful effect on the small arterial elasticity [ ].
Impaired glucose metabolism
GH has the ability to antagonize the hepatic and peripheral effects of insulin on glucose metabolism, likely due to concomitant increase in lipid metabolites and uptake [ ]. Insulin resistance, glucose intolerance, and diabetes mellitus are common features of acromegaly [ ]. A relationship between insulin and indices of subcutaneous small resistance artery structure, hypertrophic remodeling, and endothelial dysfunction along with reduced NO bioavailability have been described in patients with noninsulin-dependent diabetes mellitus [ ], which is similar to the evidence obtained in patients with acromegaly when IGF-1 was analyzed. In clinical terms, impaired glucose tolerance and diabetes mellitus significantly contribute to increased BP values seen in acromegaly [ , ]. Suggested mechanisms include an impaired NO-mediated vasodilatation, activation of RAAS, or increased sympathetic activity [ ]. Indirect evidence supports that insulin stimulates angiotensinogen and vascular smooth muscle cell growth, thus promoting vascular hypertrophy through RAAS activation [ ]. Hyperinsulinemia increases peripheral resistance through an adrenergic-mediated vasoconstriction effect [ ]. However, the role of RAAS and sympathetic activity in the acromegaly related hypertension remains inconclusive [ , ].
Sleep apnea syndrome
Obstructive sleep apnea is a frequent complication of GH excess and is characterized by five or more episodes of apnea (air flow cessation ≥10 s) and hypopnoea (diminished airflow of ≥30% for ≥10 s) on a sleep study (respiratory polygraphy or polysomnography) [ ]. GH excess is linked to sleep apnea because of tissue infiltration of the upper respiratory system (tongue, soft palate, pharyngeal and laryngeal swelling as well as vocal cord thickening due to enhanced fluid retention), or anatomical changes in the cranio-facial region (maxilla and mandibular overgrowth) [ , ]. Up to a third of patients have a central component of the sleep apnea without the obstruction of airflow, and this may be related to high GH or IGF-1 [ ]. The reported prevalence for sleep apnea varies between 44% and 87.5% in active acromegaly [ ].
Sleep apnea is independently associated with hypertension and one of the mechanisms involved is an increased adrenergic tone (due to brief awakening and hypoxia during breathing cessation). Additionally, hypoxia promotes the formation of reactive oxygen species, potentially resulting in oxidative stress, inflammation, endothelial dysfunction, and ultimately increased BP [ ]. Similar to acromegaly [ , ], sleep apnea is associated with a nondipper BP behavior [ ]. In a cohort of 37 patients with acromegaly (including patients with active disease, controlled disease, or those in remission), the risk assessment for sleep apnea was done using a screening tool (STOP-bang questionnaire). Although a respiratory polygraphy or polysomnography were not used to confirm sleep apnea in patients at high risk, a trend toward an inverse correlation was described between a high risk for sleep apnea and a nondipper behavior in acromegaly, the small sample size accounting possibly for the borderline association. Furthermore, all hypertensive patients ( n = 22) had a high risk for sleep apnea compared to normotensive patients ( n = 15) where only 18% had this high risk [ ]. Sleep apnea improves with acromegaly treatment [ ], but it still persists in controlled acromegaly among 35%–58% of the cases [ ]. These aspects suggest that active disease is not the only determinant of sleep apnea in acromegaly. Even so, treatment with continuous positive airway pressure or mandibular advancement devices is associated with reductions in BP in unselected sleep apnea population [ ].
Hypertension phenotype and cardiovascular comorbidities
Characteristics of acromegaly related hypertension
Baseline characteristics for patients enrolled in the ACROSTUDY before pegvisomant treatment showed that patients with acromegaly-related hypertension had higher body mass index (BMI), higher prevalence of diabetes mellitus, hyperlipidemia, and cardiovascular disease (CVD) and were older compared to normotensive patients with acromegaly. Because pegvisomant is a second- or third-line treatment, patients in this cohort were older and had a longer duration of active disease which might explain the higher cardiovascular risk profile in hypertensive patients. Other findings include more frequent pituitary deficiencies in hypertensive patients. In addition, patients with hypertension had less likelihood of undergoing pituitary surgery and this might be related to the older age and multiple comorbidities compared to the normotensive patients [ ]. Although this study does not describe baseline characteristics at acromegaly diagnosis, it has the advantage to include a large number of patients with inadequate control of acromegaly.
Predictive factors ( Fig. 15.3 ) identified for acromegaly-related hypertension are the following: IGF-1 levels, age at diagnosis [ ], glucose levels, estimated disease duration [ ], and BMI [ ]. IGF-1 levels are not universally seen as a predictive factor since hypertension was poorly related to IGF-1 levels at diagnosis in other studies [ , ]. Hypertension is usually not very severe [ ]; however, in patients with severe hypertension, GH was found to be higher at baseline compared to those with controlled or mild hypertension [ ]. The predictive factors for hypertension and characteristics of acromegaly related factors are summarized in Fig. 15.3 . Results from a case–control study of 200 patients with ‘ acromegaly and hypertension ‘ matched with 200 hypertensive controls for age, sex, BMI, and smoking habits showed a preferential increase in diastolic BP (DBP) in acromegaly. This could be attributed to the increased peripheral resistance through the vascular remodeling induced by GH/IGF-1 excess [ ]. However, this was not confirmed in a similar although smaller cohort [ ]. A sex-dependent difference has been observed in some studies: a Mexican registry found hypertension being more common among female patients [ ], and the German Pegvisomant Observational Study showed that acromegalic men had increased DBP only, whereas women had both increased SBP and DBP [ ]. However, these differences were not seen in other studies [ , , , , , , ].
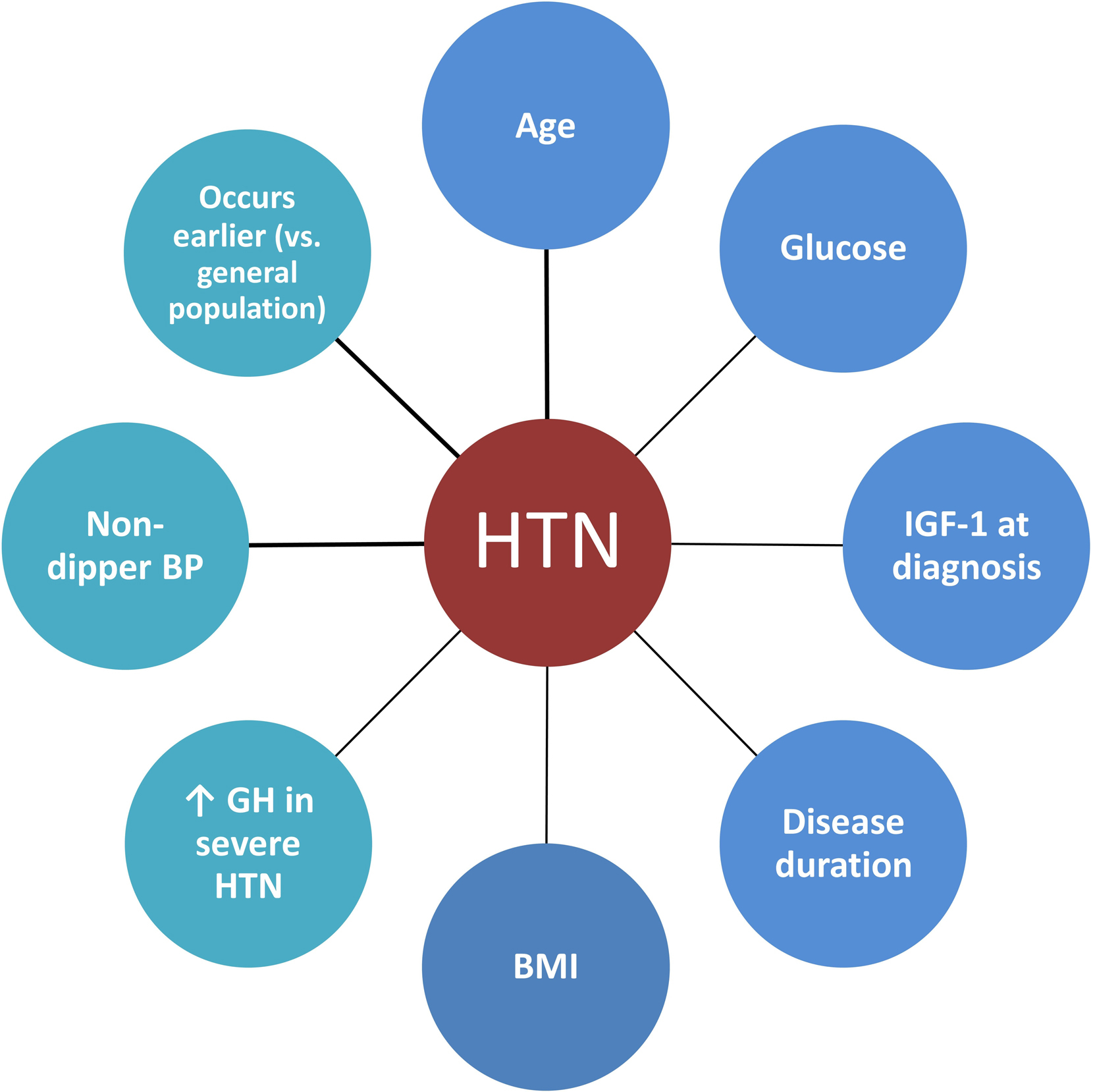
Acromegaly and comorbidities
Cardiomyopathy
Acromegaly related cardiomyopathy is a cardinal feature and develops in three stages [ , ]: (i) in the first stage, patients display a hyperkinetic cardiac syndrome which denotes increased cardiac contractility and output due to myocardial hypertrophy; (ii) the second stage is characterized by progressive hypertrophy, myocardial fibrosis, and damaged heart function which is mainly diastolic dysfunction and impaired systolic performance on effort; (iii) in the last stage, if acromegaly is untreated or uncontrolled, systolic dysfunction and heart failure occur. Left ventricular hypertrophy in acromegaly was observed in 11%–78% when analyzed by cardiac ultrasound and this wide variation in prevalence estimate may be the consequence of different methodologies used to define left ventricular hypertrophy, different study designs, or the characteristics of study population [ ]. A significantly lower frequency for left ventricular hypertrophy was detected using cardiac magnetic resonance imaging (cardiac MRI) when compared to cardiac ultrasound in patients with acromegaly ( n = 30) before treatment (5% vs. 31%) suggesting that cardiac ultrasound might overestimate the prevalence. Cardiac MRI has higher accuracy and reproducibility, and lower variability in comparison with echocardiography and this may explain the dissimilar results between the two methods of imaging [ ]. SBP, followed by GH levels [ ], and disease duration were found to be the most important predictors of left ventricular hypertrophy [ ]. Diastolic dysfunction assessed by ultrasound was observed in 11%–58% of patients, but it is usually mild with no clinical consequence and the progression to systolic dysfunction is infrequent in more recent studies [ ].
Valvulopathy
Aortic valve regurgitation has been described in a third of patients with acromegaly (30%–31% vs. controls 7%) [ , ]. Mild aortic root dilatation observed in some patients does not have any clinically relevant complications [ ]; however, gross aortic root dilation with heart failure was described in young women with acromegaly, necessitating aortic root replacement with a composite aortic graft prosthesis [ ]. A wider range of prevalence of mitral regurgitation has also been described (5%–26% vs. controls 0%–7%) [ , ].
Arrhythmias
Arrhythmias have been reported in 7%–40% of patients with acromegaly, more frequent during exercise [ ]. The prevalence of arrhythmia may vary depending on the presence of left ventricular hypertrophy because ventricular arrhythmias occurred more often in those with left ventricular hypertrophy [ ], whereas a low frequency of ventricular and supraventricular arrhythmias were reported in patients with lack of cardiac structural changes [ ]. Several types of arrhythmias have been linked to acromegaly such as paroxysmal atrial fibrillation and supraventricular tachycardia, sick sinus syndrome, isolated and paired ventricular ectopic beats, ventricular tachycardia as well as abnormally long or dispersed QT interval and a higher frequency of late potentials [ , ]. Malignant ventricular tachyarrhythmia may be the cause of repeated syncope and sudden cardiac death in patients with acromegaly [ ].
Diabetes mellitus
The prevalence of diabetes mellitus at the time of acromegaly diagnosis varies between 20% and 35% [ ]. Data from the Mexican registry ( n = 2057) showed that nearly two thirds of patients have abnormal glucose metabolism at diagnosis, since 30% of patients had diabetes mellitus, whereas 33% of patients displayed glucose intolerance or impaired fasting glucose [ ]. These data could be influenced by ethnic differences, as the prevalence of diabetes mellitus is higher in Mexicans than the average prevalence in the world (9.4% vs. 8.5%) [ ]. Furthermore, IGF-1 levels at diagnosis and the concomitant presence of hypertension were significantly associated with the presence of diabetes mellitus [ ]. In the ACROSTUDY ( n = 2090), diabetes mellitus was significantly more frequent in the hypertensive group compared with normotensive patients (20.2% vs. 11.4%) [ ].
Hyperlipidemia
GH stimulates free fatty acid release into the circulation from the adipose tissue [ ], and, therefore, dyslipidemia is expected at presentation. The prevalence of hyperlipidemia varies from 13% to 51%, typically with higher levels of triglycerides or reduced HDL levels compared to controls [ ]. Other lipid parameters such as total cholesterol, very low-density lipoprotein (VLDL), and low-density lipoprotein (LDL) levels were found to be significantly higher in 62 patients with acromegaly (50 with active disease) compared to controls [ ]. In the ACROSTUDY ( n = 2090), dyslipidemia was significantly more frequent in the hypertensive group compared with normotensive patients (17.0% vs. 5.6%) [ ].
Atherosclerosis and mortality
Patients with acromegaly present with risk factors for coronary artery disease such as hypertension, dyslipidaemia, and diabetes mellitus [ ]. Early markers of the peripheral vascular damage and predictors of cardiovascular events such as intima-media thickness (IMT) of common carotid arteries, flow- mediated dilatation (FMD), and arterial pulse wave velocity (PWV) were assessed in a meta-analysis. Results showed that patients with acromegaly had higher IMT, impaired FMD, and higher PWV compared to controls, yet controlled disease showed a significant improvement in IMT and FMD. This suggests that patients with acromegaly may be at increased risk of atherosclerosis, especially if the disease is active [ ]. The Framingham risk score is an algorithm used to calculate the likelihood of coronary artery disease over a period of 10 years, and this score was shown to be significantly higher in patients with acromegaly compared with controls [ ]. However, the incidence of myocardial infarction and stroke in acromegaly was found to be similar compared to controls in two national registries [ , ]. Hypertension rather than active acromegaly was linked to a higher rate of cardiovascular events since myocardial infarction or stroke were more common in patients with hypertension compared to those without (94% vs. 43.4%), whereas the proportion of patients with active disease was similar between the groups [ ]. An association between pituitary radiotherapy and cerebrovascular mortality has been described [ ] and similarly, in patients with acromegaly, treatment with radiotherapy was associated with increased mortality and cerebrovascular-related deaths. Compared to those without radiotherapy, the increased mortality risk persisted even after adjusting for GH and IGF-1 levels [ ].
Cardiovascular and cerebrovascular complications are among the leading causes of death in acromegaly [ ], but it seems that other factors such as hypertension [ ] or radiotherapy [ ] are linked to coronary artery disease rather than acromegaly itself. The limited use of radiotherapy [ ] due to improvement in the surgical and medical treatment of acromegaly or optimal hypertension management (82% of the patients without myocardial infarction and stroke were on antihypertensive drugs) [ ] could explain the lower risk for cardiovascular events described in the two national registries of acromegaly.
In the ACROSTUDY, a total of 68 deaths (5.1%) were observed in hypertensive patients with acromegaly compared to 10 deaths (1.3%) in patients with acromegaly and normal BP. The mortality rate was 13.2 per 1000 patient years in the hypertension group and 4 per 1000 patient years in the normotensive group. The main causes of death in these patients were cardiovascular disease (31%), cerebrovascular disease (18%), cancer (18%), and respiratory problems/sepsis (13%). The most frequent cause of death due to cardiovascular disease was heart failure, which accounted for nine cases. Two deaths were caused by ventricular fibrillation, and aortic aneurysm rupture was the cause of death in one patient. Cardiovascular disease and pituitary deficiencies at study entry predicted mortality independently. In hypertensive patients, pituitary deficiency was present in 72% of hypertensive patients who died, and in 51% of survivors. However, in patients with acromegaly and hypertension the survival was reduced in the presence of concomitant cardiovascular disease rather than pituitary deficiency [ ]. Hypopituitarism is linked to increased cardiovascular mortality, and GHD is suggested to be the culprit [ ], but this was not the case for the acromegaly cohort. Over-replacement of glucocorticoids increases the risks of metabolic and cardiovascular disease [ ], and given the fact that study is lacking information on how adequately pituitary deficiencies were treated, mortality related to overreplacement of glucocorticoids cannot be excluded [ ].
The main clinical consequences of acromegaly are depicted in the Fig. 15.4 .
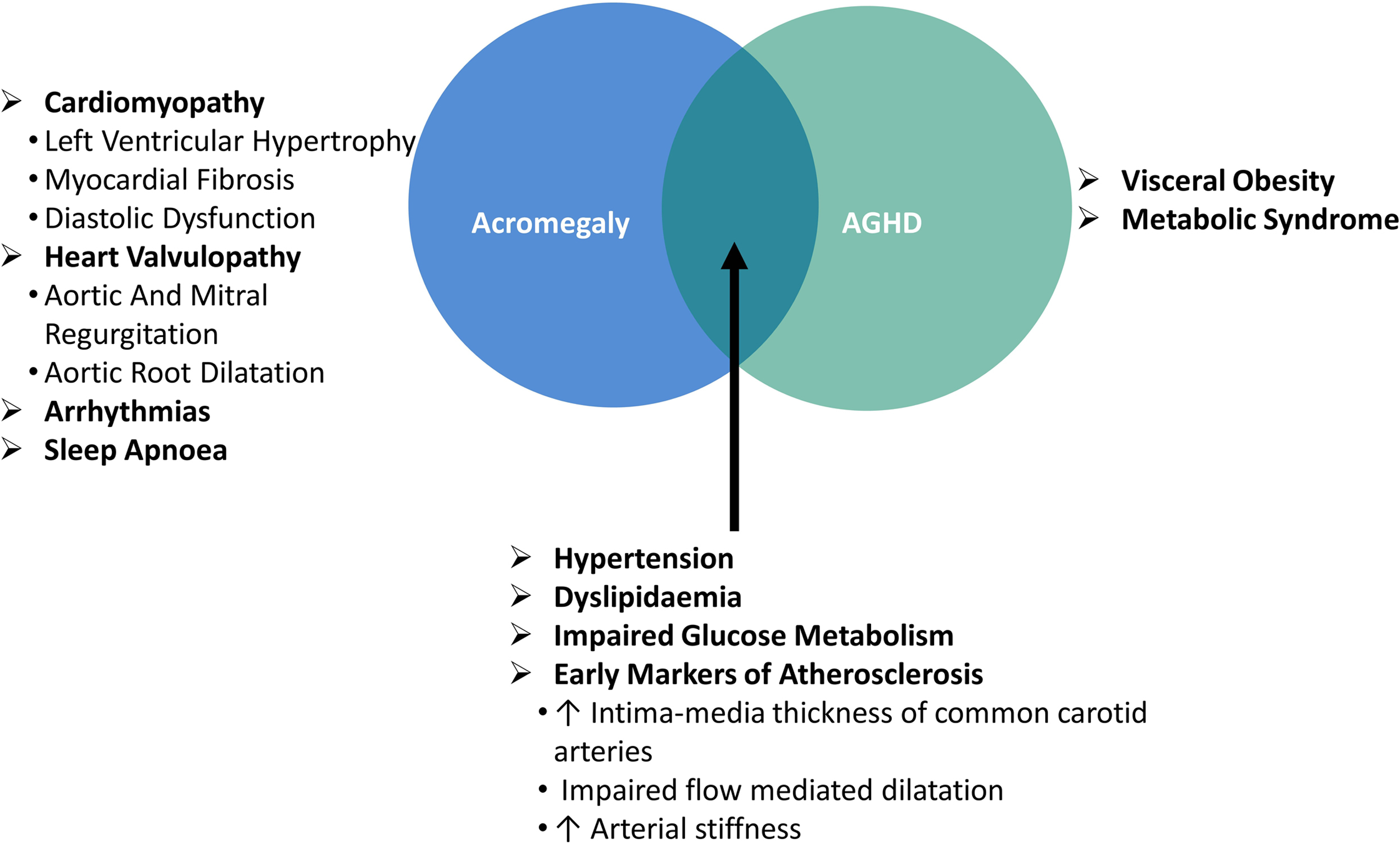
Screening for acromegaly in hypertensive patients
When to suspect acromegaly in hypertensive patients
Screening for secondary causes of hypertension should be considered after confirming that BP is elevated with ABPM [ ]. Secondary hypertension due to acromegaly ( Fig. 15.2 ) should be suspected especially in those patients with acral enlargement and facial features. In patients without typical manifestations, the suspicion of acromegaly should be raised in hypertensive patients who have several of these associated conditions: sleep apnea syndrome, diabetes mellitus (type 2), debilitating arthritis, carpal tunnel syndrome, and hyperhidrosis [ ]. Biochemical screening of every hypertensive patient systematically for acromegaly is not recommended.
A study that gathered 1,209 patients seen by the general practitioners with newly diagnosed hypertension with at least one comorbidity (chronic headache, diabetes mellitus, chronic diffuse arthralgia, carpal tunnel syndrome, and hyperhidrosis) in the absence of acral enlargement showed that 22 patients had elevated IGF-1. Of those, four had inadequate GH suppression after glucose load; however, MRI did not reveal any changes in the pituitary, nor did chest and abdominal contrast-enhanced computed tomography. At the time of the publication, patients were followed for up to 3 years. Though an ectopic source for GH was considered unlikely in the absence of imaging findings or disease progression, it was not ruled out as longer follow-up is needed [ ]. It is well known that in some cases there is a discrepancy between IGF-1 and GH. This can be encountered in certain conditions such as hepatic and renal failure, hypothyroidism, malnutrition, severe infection, and poorly controlled diabetes mellitus where IGF-1 can be falsely elevated, normal, or low [ ]. The degree of GH suppression depends on BMI, sex, and estrogen status in women [ ]. Secondary hypertension in patients with acromegaly without enlargement of the extremities is rare; however, acromegaly remains underdiagnosed. Data from 324 patients with acromegaly presenting between 1981 and 2006 were retrospectively analyzed and results showed that the delay in diagnosis was an average of 5.3 ± 4 years [ ], and patients typically visit on an average 3.4 physicians prior to diagnosis [ ]. Delays of 15 or even 25 years have been reported in the diagnosis of acromegaly [ ]. Patients with acromegaly may present with common signs and symptoms (including hypertension), but a long diagnostic delay among these patients could reflect a failure of healthcare professionals to recognize the disease earlier [ ]. As such, an increased awareness of acromegaly among healthcare professionals is needed.
Patients with newly diagnosed acromegaly should be screened for hypertension, cardiovascular disease, sleep apnea, diabetes mellitus [ ], and dyslipidaemia [ ]. The diagnosis of hypertension should be based on repeated office-based BP measurements on more than one visit (except when hypertension is severe), or on out-of-office BP measurements (using ABPM). The latter has the advantage to identify white-coat and masked hypertension but can be expensive and has limited availability [ ]. Cardiac ultrasound is recommended at diagnosis to evaluate the presence of cardiomyopathy [ ]. If suggestive symptoms are present for cardiac rhythm abnormalities, a 12-lead electrocardiogram, or a 24-hour Holter monitoring could be used depending on the circumstances [ ]. It is worth mentioning that additional screening is recommended in acromegaly for bone fractures (vertebral fracture assessment), colon neoplasia, and thyroid neoplasia if clinically indicated (palpable thyroid nodule) [ , ].
Treatment
Therapeutical options in acromegaly
Treatment options include transsphenoidal surgery (TSS), medical therapy, or radiotherapy. TSS is the primary treatment for most of the patients. Tumor size and invasiveness of surrounding structures, especially the cavernous sinus, are important determinants of surgical remission [ ]. If the surgical resection is unlikely due to parasellar extension, surgical debulking to improve treatment response to medical therapy is recommended [ ].
In case of persistent disease (patients who do not achieve biochemical control), medical therapy is recommended, although reoperation is also an option. For milder disease, a trial of dopamine agonists (cabergoline) is suggested, whereas for significant disease, somatostatin analogues (SSAs: lanreotide, octreotide, or pasireotide in some patients) or GH-receptor antagonists (pegvisomant) can be used. If the patient is a poor surgical candidate, medical treatment can be used as a first line. Radiotherapy is regarded as an adjuvant therapy when surgery and medical therapy have failed to control disease, although, it may be considered at any point after incomplete surgery [ , ].
The effect of disease activity on blood pressure
Successful treatment of acromegaly improves the BP in hypertensive patients in up to a third of the cases [ ]. In one study, after 24 months of treatment for acromegaly, in those who had controlled disease, up to 70% also had controlled hypertension, whereas in those with inadequate control of acromegaly, hypertension had been controlled only in 24% of the cases [ ]. Not all studies report an improvement in BP after acromegaly treatment [ ]. Both first-line surgery and SSA treatment reduced the BP in a similar way [ ], but when compared to pegvisomant, surgery was associated with a significant BP reduction [ ]. A possible explanation for this latter result is the fact that most patients on pegvisomant treatment have more challenging acromegaly, and hence it is more difficult to control the disease and consequently the BP. Acromegaly treatment may impact differently the components of BP: a preferential decline in SBP [ , , ] or DBP [ , ] or both [ , ] have been reported.
The impact of each treatment modality on BP is summarized in Table 15.2 .
No. of patients | Cohort description (pre-treatment) | Treatment | Controlled acromegaly | Poorly controlled | Reference |
---|---|---|---|---|---|
30 (15 CD vs. 15 UD) | HTN (40%); BP nondipper profile (35%) | TSS | ↓24-h-SBP∗ ↑ 24-h-DBP BP circadian rhythm restored in 83% | ↑ 24-h-SBP ↑ 24-h-DBP Persistent non-dipper pattern | [ ] |
31 | HTN ( n = 10) BP nondipper profile ( n = 15) | TSS | ↓24-h-SBP∗ ↔ 24-h-DBP HTN cured ∼ 33% BP circadian rhythm restored in 80% | [ ] | |
380 | Systematic review; hypertensive cohort in 1 study | SSAs | (3 studies, n = 72) ↓ BP (14 studies, n = 308) ↔ BP | [ ] | |
89 (56 first line SSAs vs. 33 first-line surgery) | For stage 1 HTN: 33.9 vs. 36.4% For stage 2 HTN: 25% vs. 18.2% | Surgery, SSAs | ↔ SBP ↓ DBP∗ For stage 1 HTN: 25% vs. 27.3% For stage 2 HTN: 10.7% vs. 6.1% | [ ] | |
96 | HTN ( n = 21) | Surgery, SSAs, PEGV | ↓24-h-SBP∗ in HT; ↓24-h-SBP∗ surgery vs. PEGV | [ ] | |
105 (76 CD vs. 29 UD) | All patients were HT | SSAs alone or as part of multimodal treatment in 103/105 | ↓SBP∗,↓ DBP∗; Controlled HTN in ∼70% | ↓ SBP, ↓ DBP Controlled HTN in ∼24% | [ ] |
58 (28 NT vs.30 HT, of which 17 CD vs. 13 UD) | Surgically naïve patients; HT cohort | SSAs ± PEGV or PEGV alone | CD in 56.7% of HT vs. 53.6% of NT; ↓24-h-SBP and ↓24-h-DBP∗ | ↑ 24-h-SBP∗, ↓24-h-DBP Overt HTN in 46% of NT | [ ] |
2,090 (1,344 HT vs. 747 NT) | 64% HT | PEGV alone or PEGV ± SSAs ± DAs | ↓ SBP and ↓ DBP in HT and NT | [ ] |
Hypertension management
In patients where acromegaly treatment did not improve the BP, standard antihypertensive drugs should be used [ ]. There is not enough evidence for preferring any particular antihypertensive drug class over other classes for the treatment of hypertension in patients with acromegaly; however, given the enhanced activity of ENaC, treatment with amiloride could be more efficient in theory [ ]. Currently, amiloride is reserved for cases when spironolactone is not tolerated [ ].
Growth hormone deficiency and hypertension
Adult GH deficiency (AGHD) is a clinical entity that has been recognized as a syndrome three decades ago [ ], and is characterized by a clustering of cardiovascular risk factors which overlap with the metabolic syndrome regarding visceral obesity, impaired glucose metabolism, dyslipidaemia, and hypertension [ ]. AGHD is a relatively rare disease with an estimated prevalence of 2–3 per 10,000 population [ ], and an average incidence rate of 1.65 per 100,000 [ ].
Epidemiology
The reported prevalence of hypertension varies from less than 20%–66% [ , ], but it can reach up to 90% [ ] in AGHD patients ≥65 years of age. Hypertension is more frequent in AGHD compared to controls or background population. In a cohort of patients ( n = 104) with adulthood-onset of GHD without GH-replacement, but with adequate substitution for other pituitary hormone deficiencies, the prevalence of hypertension was 11.3% for men and 15.1% for women [ ]. In a larger cohort ( n = 926), the prevalence of hypertension was reported to be 22.6% for men and 21.7% for women [ ]. In another large cohort ( n = 632), hypertension was present in 18% of GHD patients [ ]. In smaller cohorts, hypertension was present at study entry in 66% of patients with treatment-naïve AGHD ( n = 50) [ ]. More recently, the prevalence of hypertension at study entry was reported to be 90% ( n = 9) in AGHD patients ≥65 years of age and 13.7% ( n = 4) in AGHD patients below 65 years [ ]. The prevalence of hypertension may vary due to different criteria to define hypertension or different background of the studied population, and different methods to assess BP. Additionally, given the relative rarity of the disease, the group of AGHD is very heterogeneous, and the presence of other pituitary deficiencies or certain etiologies that resulted in AGHD (craniopharyngioma, Cushing’s disease or acromegaly) may represent an additional cardiovascular risk factor [ ].
KIMS is a multinational, pharmacoepidemiological surveillance database that contains prospectively collected observational data from almost 16,000 adult patients with GHD from 31 countries [ , ]. The KIMS database provides subgroup analysis for naïve- (without GH treatment) and seminaïve AGHD patients (with GH replacement discontinued for at least 6 months before the enrollment in the study). The presence of multiple pituitary hormone deficiencies is suggested to be associated with a worse cardiovascular profile compared to isolated GHD [ ]; however, hypertension was present in about 15% in both subgroups at study entry into KIMS [ ].
The etiology of AGHD is heterogeneous, but the most frequent cause is a pituitary tumor (with the nonfunctioning pituitary adenoma being the most common subtype) followed by craniopharyngiomas. These two etiologies account for approximately 57% of the cases, whereas treated acromegaly or treated Cushing’s disease account for 1.7% and 6.14%, respectively of AGHD cases [ ].
Hypertension was slightly more common (9.4% vs. 8.7%) in patients with craniopharyngioma ( n = 351) compared to patients with nonfunctioning pituitary adenomas ( n = 370) but did not differ significantly between the groups at study entry [ ]. The prevalence of hypertension did not differ (25% vs. 26%) between post-acromegaly GHD ( n = 115) and GHD due to a nonfunctioning pituitary adenoma ( n = 142) [ ]. This was not the case for patients post-Cushing’s disease GHD ( n = 135), since the prevalence of hypertension was higher in this group compared to GHD due to other etiologies ( n = 1392) including nonfunctioning pituitary tumors [ ].
Childhood-onset of GHD (CO-GHD) represent approximately 22% of all AGHD [ ]. Differences exist between CO-AGHD and adulthood-onset of GHD (AO-GHD) in terms of etiology and prevalence of hypertension. In CO-GHD idiopathic/congenital GHD is responsible for 30%–50% of the cases, and is followed by craniopharyngioma in 23% of the cases with GHD and multiple pituitary deficiencies, or by other sellar and extrasellar tumors in isolated GHD. Hypertension in CO-AGHD is less frequent than in AO-GHD (<5%) [ ]. The prevalence of hypertension in AGHD is summarized in Table 15.3 . The AGHD groups are mainly AO-GHD.
Country of study | No. of patients | AO-GHD or CO-GHD | GH-treatment naïve | Etiology for AGHD | HT prevalence | Reference | |
---|---|---|---|---|---|---|---|
AGHD | Controls or reference population | ||||||
Sweden | 104 (66 M, 38 F) | AO-GHD | Naïve | Mixed | 11.3% (men) 15.1% (women) | 8% (men) 5.5% (women) | [ ] |
Spain | 926 (441 M, 485 F) | AO-GHD | Naïve | Mixed | 22.6% (men) 21.7% (women) | 14.9% (ranging from 12.2% to 16.2%) | [ ] |
Germany | 122 (79 M, 43 F) | N/A | Naïve | Mixed | 18% (men) 19% (women) | 16.5% (men)∗ 13.9% (women)∗ | [ ] |
The Netherlands | 50 (48% M, 52% F) | AO-GHD | Naïve | Mixed | 66% | 35% | [ ] |
Italy | 29 (10 EGHD patients, 29 AGHD) | N/A | Naïve | Mixed | 90% EGHD 13.3% AGHD | 50% EGHD 33.3% AGHD | [ ] |
International (KIMS data) | 167 IGHD, 1992 MPHD | AO-GHD | Mixed (naïve and seminaïve) | Mixed | 15% IGHD 15% MPHD | [ ] | |
International (KIMS data) | 351 CP patients (189 M and 162 F; vs. 370 NFPA (185 M, 185 F) | AO-GHD | Mixed (naïve and seminaïve) | CP and NFPA | 9.4% CP 8.7% NFPA | [ ] | |
International (KIMS) | 115 adults Post-acro GHD (71 M, 74F); 142 NFPA (79 M, 63 F) | N/A | Mixed (naïve and seminaïve) | Post-acro GHD and NFPA | 25% Post-acro GHD 26% NFPA | [ ] | |
International (KIMS) | 135 post-Cushing (78% F, 22% M) | AO-GHD | 66% of patients had previously received GH therapy | Post-Cushing’s AGHD | ∼25% (men) 30% (women) | [ ] | |
International (KIMS) | 1.395 AGHD <65yr; 170 AGHD >65yr | AO-GHD | Mixed (naïve and seminaïve) | Mixed | 43.1% (>65yr) 13.6% (<65yr) | [ ] |
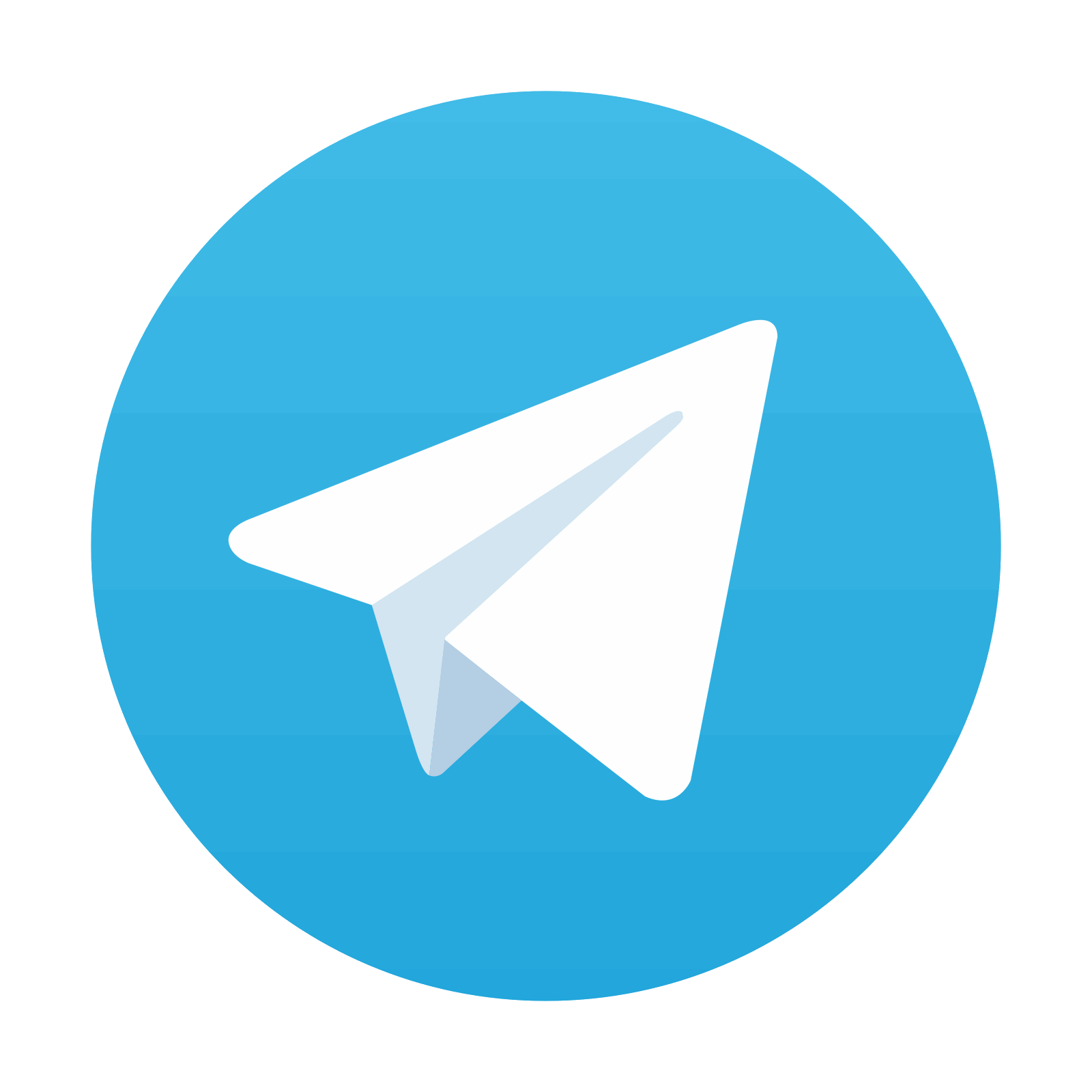
Stay updated, free articles. Join our Telegram channel

Full access? Get Clinical Tree
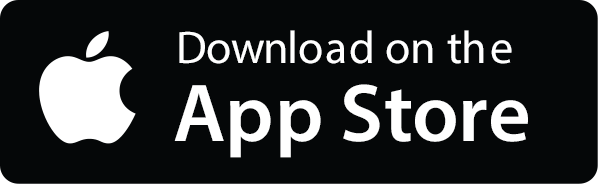
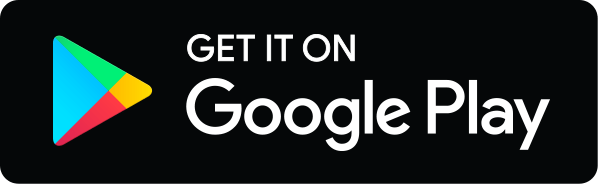
