Structural feature
Examples
Composition of particle core
Cholesteryl esters [54]
Inorganic scaffolds (Au) [89]
Shape
Discoidal [80]
Spherical [89]
Protein associated with particle
Full-length apoA-I [97]
ApoA-I-mimetic peptide [99]
ApoE [96]
Phospholipids
Phosphatidylcholine (PC) [69],
1,2-dioleoyl-sn-glycero-3-phosphocholine (DOPC) [98]
1,2-dimyris-toyl-sn-glycero-3-phosphocholine (DMPC) [98]
1,2-dioleoyl-sn-gly- cero-3-((N(5-amino-1-carboxypentyl)iminodiacetic acid) succinyl)(- nickel salt) (NiLipid) [98]
1,2-distearoyl-sn-glycero-3-phosphoethanolamine-N-[folate(polyethylene glycol)-2000] (DSPE-PEG2000-folate) (PF) [98]
Pyropheo-phorbide conjugated to the glycerol backbone of lysophospholipids [80]
Lipid layer surrounding the particle
Monolayer [91]
Bilayer [89]
Mechanism of drug loading
Covalent attachment [98]
Encapsulation [102]
Integration in lipid layer [73]
Additional targeting moieties
Folic acid, covalently linked to lysine residues of apoA-I [103]
Lipid-conjugated folate (1,2-distearoyl-sn-glycero-3- phosphoethanolamine-N-[folate(polyethylene glycol)-2000] (DSPE-PEG2000-folate) (PF) [98]
Incorporated drug
Paclitaxel [83]
Doxorubicin [70]
Valrubicin [62]
Fenretinide [74]
Incorporated fluorophore
Fluo-BOA [72]
Lipid-conjugated Rhodamine B [104]
3.3 HDL-like Nanoparticles for Drug Delivery
3.3.1 Discoidal HDL NPs for Drug Delivery
Both discoidal and spherical HDL-like NPs have been shown to target SR-B1 [64] and have therefore both been employed for targeted drug delivery to cancer cells. While spherical HDL-like NPs are more similar in shape to the more mature versions of their natural counterparts, discoidal HDL-like NPs are more reminiscent of nascent HDL. Like natural, nascent HDL, discoidal HDL-like NPs are prone to undergo maturation in the bloodstream due to interaction with LCAT [65–68]. This biological maturation of discoidal HDLs can lead to unwanted leakage of drug cargo [69]. To combat this problem, researchers used monocholesterylsuccinate (CHS) instead of cholesterol to prevent particle interaction with LCAT, and anchored apoA-I on the particle by covalently linking it to CHS [70, 71]. The authors employed a CHS-modified discoidal HDL-like NPs, termed recombinant HDL (rHDL), and loaded it with paclitaxel, a mitotic inhibitor used to treat a variety of cancers. Studies in rats showed improved drug levels in the blood over extended periods of time both compared to drug-loaded, but unmodified particle (P-d-rHDL), as well as compared to free or liposome-loaded drug [71]. Further, paclitaxel-loaded CHS-modified particles (cP-d-rHDL) showed increased cytotoxicity and uptake in the human cancer cell line MCF-7 compared to P-d-rHDL. In breast tumor bearing mice, cP-d-rHDL were superior in regard to both tumor targeting as well as limiting tumor growth compared to P-d-rHDL, liposome-loaded drug or free drug [70].
3.3.2 Spherical HDL-like NPs for Drug Delivery
Delivery of hydrophobic antineoplastic agents by HDL-like NPs is often achieved by encapsulation of the drug in the hydrophobic core. Recently, Lacko and co-workers showed that inclusion of highly water-insoluble drugs, like valrubicin, into spherical HDL-like NPs composed of phosphatidyl choline, apoA-I, free cholesterol, and cholesteryl oleate, led to increased toxicity in SR-B1 expressing malignant prostate and ovarian cancer cell lines compared to the free drug [62, 72]. Further, off-target cytotoxic effects in non-malignant epithelial prostate and ovarian cell lines with low SR-B1 expression were decreased for the drug-containing particle compared to the free drug [62, 72]. Encapsulation of valrubicin into HDL-like NP may expand the therapeutic spectrum of the drug, which had previously been used exclusively for the treatment of bladder cancer. The authors also used a similar NP construct to target the therapeutic agent fenretinide to two different neuroblastoma cells lines in vitro [72]. Compared to the free drug, cytotoxicity in the malignant cell lines was significantly increased, while cytotoxicity in retinal pigment epithelial cells, a control cell line for off-target fenretinide toxicity, was reduced [62, 72]. Feng and co-workers used a similar strategy of drug encapsulation to target the chemotherapeutic doxorubicin to hepatocellular carcinoma and hepatoma cells lines [73, 74]. In this work, HDL-like NPs were composed of egg phospholipids, apoA-I, and doxorubicin and showed increased cytotoxicity, apoptosis induction, and conjugate accumulation in target cells compared with the free or liposome encapsulated drug [73]. The drug-loaded HDL-like NPs also decreased tumor growth in a metastatic model of human hepatocellular carcinoma (HCC) in nude mice and reduced hemolysis-related side effects [74].
To better understand the uptake of lipophilic cargo from spherical HDL-like NPs through SR-B1, Lin et al. developed multifluorophore-labeled HDL-mimicking peptide phospholipid scaffold (HPPS) nanoparticles [75]. Although apoA-I is critical for determining the shape of HDLs and allowing for specific interactions with cellular receptors, the protein can be replaced by short (<20 amino acids) peptides that show no sequence similarity to the full-length protein [76]. These peptides mimic the amphipathic helical structure of apoA-I, including its receptor and lipid binding abilities [76]. Lin et al. [75] generated a variety of different cholesteryl oleate/phosphocholine HDL-like NPs with either fluorescent compounds in the particle core, fluorescently labeled apoA-I peptides and phospholipids on the surface, or combinations thereof. Through sequential inhibition studies, they found that after initial interaction of the HDL-like NP with SR-B1, the particle bound to a specific sub-domain of the receptor, leading to particle dissociation and internalization of the hydrophobic cargo into the cytosol by a lipid-raft/caveolae-like mechanism. Phospholipids and apoA-I-mimetic peptides were mainly retained on the cell surface. It is important to note that for natural, mature HDL, selective influx of CE payload does not require particle catabolism [77, 78], suggesting that the cellular fate of specific HDL-like NPs might be dependent on their specific composition and determined by additional factors that warrant further investigation.
3.3.3 HDL-like NPs for the Delivery of Photothermal Therapeutics
HDL-like NPs have also been used for the delivery of photothermal agents, which facilitate infrared light-induced temperature changes in tumor tissues leading to tissue necrosis. Mathew et al. generated an HDL-like NP composed of phosphocholine, apoA-I fused to a trans-activating transcriptional activator peptide (TAT-peptide, for enhanced cell internalization), and a water-insoluble gadolinium bis(naphthalocyanine) sandwich complex (as a photothermal compound) [79]. Using this particle, the authors achieved photothermal killing of human lung cancer cells in a near infrared light-irradiation-dependent manner. In addition, delivery of the photothermal compound was dependent on the expression of SR-B1 by target cells. Also, by conjugating pyropheophorbide, a reduced porphyrin, to lysophospholipids, Ng et al. created a phototherapeutic fluorescent pyro-lipid, which self-assembled with apoA-I into nanodiscs [80]. The authors used these particles to treat Chinese hamster ovary (CHO) cells that were transfected to express SR-B1. The fluorescence of the photosensitizer was quenched in intact particles; however, upon cellular uptake fluorescence of the particle became un-quenched, likely due to disruption of particle structure. Importantly, transfected SR-B1-expressing cells exhibited a dose-dependent decrease in survival when treated with 660 nm light, whereas SR-B1 negative, untransfected CHO cells neither took up the particles nor were affected by light treatment [80]. To our knowledge, these and other constructs are awaiting efficacy testing in murine models.
3.3.4 Modification of HDL-like NP for Uptake via Alternate Receptors
HDL-like NPs can also serve as scaffolds for the attachment of alternative targeting ligands for binding receptors beyond SR-B1. Corbin et al. [81] exploited the fact that over 90 % of non-mucinous ovarian cancers overexpress folate receptor-α (FR-α), as previously reported [82]. The authors first synthesized HDL-like NPs composed of apoA-1, egg yolk phosphatidylcholine, and cholesteryl oleate and then covalently attached folate to the lysine residues of apoA-I. Folate modification abolished the ability of the particle to interact with SR-B1, therefore re-routing the HDL-like NP to FR-α. The researchers used this technique to specifically deliver a near-infrared fluorescent dye to ovarian tumors in mice, enabling in vivo tumor imaging [81, 83]. Current studies are underway to utilize these particles to deliver therapeutic antineoplastic agents as well.
3.3.5 Natural HDLs for Systemic Delivery of siRNA
The potential of HDLs and HDL-like NPs as delivery vehicles for nucleic acids emerged from a few key studies. The first data demonstrating the interaction between natural HDLs and siRNAs focused on siRNA sequences terminally modified with lipophilic moieties, like cholesterol. Addition of lipophilic siRNAs resulted in siRNA-specific gene silencing in cultured cells in vitro and in the liver following systemic administration [84–86]. These observations prompted investigations to better understand how lipophilic siRNAs were being productively delivered to target cells and the liver. Toward this end, Wolfrum et al. demonstrated that systemically injected lipid-modified siRNAs spontaneously bound lipoproteins in the serum (i.e., HDL and LDL). As such, lipoprotein-bound siRNAs were then delivered to tissues that express receptors for specific lipoproteins. In the case of HDL-bound siRNAs, delivery was mediated by SR-B1 [87].
In addition, upon extraction of natural HDLs from human plasma, Vickers et al. demonstrated that HDLs naturally bind and stabilize microRNAs [14]. Further, they found distinct microRNA profiles from HDL of healthy human samples compared to subjects with hypercholesterolemia. For example, the most abundant microRNA associated with HDLs in healthy subjects was hsa-miR-135a, whereas hsa-miR-223 was the most abundant microRNA found in hypercholesterolemic patients. To determine if HDL had the capacity to load microRNAs in vivo, rHDLs free of RNA were intravenously injected into wild-type or apoE null mice. After 6 h of incubation, rHDLs were isolated from mouse plasma. rHDLs isolated from healthy mice produced 110 unique miRNAs while rHDLs isolated from the apoE null mice were found to contain 162 microRNAs. These results suggest that HDL has the capacity to bind microRNAs in vivo. In addition, Vickers et al. determined that HDL transfers microRNA to recipient cells though ABCA1. By increasing the expression of ABCA1 in J774 murine macrophage cells using a liver-X-receptor-α agonist (LXRα), they were able to demonstrate an increase in abundance of miR-223 associated with rHDL in the presence of the LXRα. Finally, to determine the importance of SR-B1 mediated microRNA delivery, baby hamster kidney (BHK) cells were stably transfected with an inducible human SR-B1 vector to increase the expression of SR-B1. Upon treatment of induced BHK cells with native HDL and native HDL pre-complexed with miR-223, induced BHK cells resulted in a 69-fold increase in intracellular levels of hsa-miR-223 compared to induced BHK cells treated with native HDL. These data suggest that HDL delivered microRNA depends on SR-B1 expression. These data demonstrate that natural HDLs bind, stabilize, and productively deliver RNAs to cells that express SR-B1.
Collectively, it has been demonstrated that HDLs are natural nucleic acid delivery vehicles with high potential for overcoming hurdles associated with systemic siRNA delivery. As such, there has been a significant focus on the development of synthetic HDL-like vectors for the delivery of nucleic acids. Importantly, and described below, synthetic HDL-like platforms vary with regard to size, charge, and surface chemistry. The goal of these efforts is to understand the parameters of natural HDLs that lead to RNA binding, stabilization, and productive delivery to target cells, like cancer cells, that express receptors for HDL.
3.4 Synthetic HDL-like Nanoparticles for Nucleic Acid Delivery
3.4.1 Spherical HDL Gold Nanoparticles for Delivery of Nucleic Acids
Our research group pioneered the synthesis of HDL-like NPs using a gold nanoparticle (AuNP) core template. We demonstrated that these particles tightly bind cholesterol (Kd = 3.8 nM) [88] and function to modulate cholesterol metabolism in target cells through the same receptors as natural HDLs [89, 90]. Because our HDL-like AuNPs tightly bind cholesterol, and natural HDLs spontaneously associate with lipidated siRNAs after systemic administration, we hypothesized that our HDL-like AuNP platform could be utilized to adsorb cholesterol modified nucleic acids for delivery to target cells that express SR-B1. Initially, we tested this hypothesis using cholesterylated antisense DNAs (chol-DNA) for delivery to prostate cancer cells that express SR-B1 [91]. As in our previous work, we developed a synthetic HDL-like nanostructure that closely mimics the size, shape, and surface chemistry of natural, mature spherical HDLs [88]. A 5 nm AuNP was used as a scaffold to control the size, shape, and composition of synthetic, spherical HDL-like AuNPs. We termed these high-density lipoprotein-like gold nanoparticles (HDL-like AuNPs). They are similar in size to their natural mature spherical HDL counterparts and have a similar surface composition: approximately 3 copies of apoA-I and an outer phospholipid layer of zwitterionic 1-2-dipalmitoyl-sn-glycero-3-phosphocholine (DPPC) [88], [89]. Moreover, chol-DNAs bound to HDL-like AuNPs (chol-DNA-HDL-like AuNPs) can overcome many of the difficulties associated with in vitro nucleic acid delivery. For instance, HDL-like AuNPs stabilize chol-DNA against nuclease degradation. Further, chol-DNA-HDL-like AuNPs do not exhibit off-target cellular toxicity and are efficient conjugates for delivering nucleic acid to regulate target gene expression. Following cell treatment, transmission electron micrographs suggested that chol-DNA-HDL-like AuNPs bypass endolysosomal sequestration, a major biological hurdle in nucleic acid delivery. This manuscript was the first to demonstrate synthetic, biomimetic HDL-like AuNPs as a delivery vehicle for nucleic acids [91].
In addition, our group recently reported the ability of HDL-like AuNPs to deliver cholesteryl-modified single stranded RNA (RNAi-HDL-like AuNPs) for modulating gene expression in the context of neovascularization and angiogenesis, both in vitro and in vivo [92]. RNAi-HDL-like AuNPs were functionalized with antisense RNA targeting vascular endothelial growth factor receptor 2 (VEGFR2), the receptor for vascular endothelial growth factor (VEGF), a key regulator of neoangiogenesis. The RNAi-HDL-like AuNPs were delivered to cultured vascular endothelial cells, which express SR-B1, and are responsible for the formation of new blood vessels. RNAi-HDL-like AuNPs effectively reduced VEGFR2 expression in target endothelial cells. In addition, treatment with RNAi-HDL-like AuNPs also reduced VEGF-induced endothelial cell survival and morphogenesis. Importantly, knockdown of SR-B1 expression by cultured endothelial cells reduced the uptake of RNAi-HDL-like AuNPs and drastically reduced VEGFR2 knockdown. Accordingly, SR-B1 expression, like for natural nucleic-acid-carrying HDLs, was shown to be required for the internalization and function of RNAi-HDL-like AuNPs. Most importantly, in vivo data showed that RNAi-HDL-like AuNPs reduce neovascularization induced by VEGF after local and systemic injection of the conjugates. Further, systemic administration of RNAi-HDL-like AuNPs targeting VEGFR2 significantly reduced target gene expression, tumor volume, and tumor weight in Lewis lung carcinoma tumor xenografts, a tumor model well known for its high degree of vascularity. Collectively, these data suggest that synthetic, spherical HDL-like AuNPs are an efficient delivery vehicle for systemic nucleic acid delivery to cells and tissues involved in carcinogenesis both in vitro and in vivo.
3.4.2 HDL-Mimicking Peptide-Phospholipid Scaffold (HPPS) Nanoparticles for siRNA Delivery
Other HDL-like NPs have been used to deliver nucleic acids. Yang et al. [93] designed an HDL-like NP using a peptide-phospholipid scaffold, termed HPPS particles. The particles consisted of phospholipids, cholesteryl oleate, and amphipathic α-helical peptides, which mimic apoA-I. The components self-assembled into structures similar to plasma-derived HDL. Direct incubation of HPPS particles with cholesteryl conjugated siRNAs (chol-siRNA) targeting the oncogene B-cell lymphoma-2 (chol-si-bcl-2) resulted in final constructs with a hydrodynamic diameter of 25.3 ± 1.2 nm and contained an average of 8 chol-siRNAs per particle. The surface charge of the particle shifted from −2.7 ± 1.9 mV to −15.2 ± 4.8 mV when chol-siRNA was added, which is consistent with RNA loading. Data showed that HPPS particles delivered siRNA cargo to the cytosol of SR-B1 expressing cells and regulated target gene expression. Treatment of KB cells expressing SR-B1 resulted in a 35 ± 9 % reduction of BCL-2 protein as compared to the control, and a 2.5-fold increase in apoptosis was measured when compared to chol-si-bcl-2 siRNA alone. Importantly, HPPS chol-si-bcl-2 particles were not effective in knocking down Bcl–2 in HT1080 cells that express minimal SR-B1. Furthermore, and consistent with SR-B1 expression, HPPS particles efficiently deliver siRNA cargo to the cytoplasm of target cells, bypassing endolysosomal sequestration. These results suggest that HPPS chol-si-bcl-2 particles utilize SR-B1 for efficient siRNA delivery and target gene knockdown.
3.4.3 Reconstituted HDL for siRNA Delivery
As mentioned above, reconstituted HDLs (rHDLs, distinct from the HDL-like NPs described in Sect. 3.3.1), are synthetic forms of human HDL essentially composed of phospholipids, apoA-I, cholesterol, and esterified cholesterol [63]. For siRNA delivery, Shahzad et al. [94] incorporated siRNA into rHDL nanoparticles. The particles were approximately 10 nm in diameter, had a net neutral charge (−3.2 mV), and demonstrated efficient delivery of siRNA to target cells. Further, rHDL-siRNA conjugates were shown to regulate target gene expression after systemic administration in orthotopic ovarian and colorectal cancer models. This group found that rHDLs loaded with fluorescently labeled siRNA showed preferential uptake in mouse tissues that highly expressed SR-B1 (i.e., tumor and liver), with minimal fluorophore-labeled siRNA in other tissues such as the brain, heart, lung, kidney, and spleen. Importantly, this work provides insight into cell-specific targeting in vivo, especially in tumor bearing mice, suggesting efficient delivery to tumor cells that express SR-B1.
A similar approach to delivering siRNA using an rHDL nanoparticle has been demonstrated by Ding et al. [95]. Here, rHDL nanoparticles were synthesized using a mixture of phospholipids, apoA-I, cholesterol, and cholesteryl esters. In contrast to the previously cited work, Ding et al. incorporated cholesteryl modified siRNA sequences into the rHDL nanostructure. This approach yielded a rHDL/chol-siRNA complex that was ~90 nm in diameter with a near-neutral charge of −4.2 mV. Consistent with previous findings, the rHDL protected siRNA from nuclease degradation and rHDL/chol-siRNA was effective in silencing gene expression in vitro and in vivo. The siRNA sequence was targeted to Pokemon. Pokemon is a proto-oncogene overexpressed in a number of human cancers and induces carcinogenesis by repressing two key tumor suppressive pathways: one, the alterative reading frame (ARF)-multiple murine double minute gene 2 (HDM2)-p53 pathway, and two, the retinoblastoma (Rb)-early-region-2 transcription factor (E2F) pathway. Systemic administration of rHDL/chol-siRNA particles complexed with siRNA targeting Pokemon demonstrated a reduction in tumor volume of HepG2 xenografts.
3.4.4 ApoE Lipopeptide Nanoparticles for siRNA Delivery
In addition to apoA-I, apoE has been used as a targeting moiety for HDL-like NPs. Dong et al. [96] generated a library of NPs composed of phospholipids, cholesterol, polyethylene glycol-lipid, siRNA, and different lipopeptides––lipids with constituent groups conjugated to different amino acids, peptides, and polypeptide head groups. The library was generated to evaluate combinations of lipoamino acid derivatives designed to mimic apolipoproteins associated with natural lipoproteins. They initially screened the lipopeptide nanoparticle’s (LNP) capacity to silence Factor VII (a blood clotting factor) in mice following intravenous injection. Upon further screening, a lead compound, CKK-E12, was used to evaluate the silencing of phosphatase and tensin homolog (PTEN) in different tissues after intravenous administration in mice. Data showed a significant silencing of PTEN in the liver compared to the lung, spleen, kidney, heart, and brain. On further examination of the liver, CKK-E12 LNPs silenced over 80 % of PTEN in hepatocytes; however, it showed no significant silencing in endothelia cells and leukocytes in the liver with comparison made to a luciferase siRNA-formulated CKK-E12 LPN, as a control. To study the effects of cell uptake and gene silencing with regard to apolipoprotein species, CKK-E12 LNPs were mixed with 11 isoforms of apoA, apoB, apoC, apoE and apoH. Upon transfection of HeLa cells, results showed that apoA, apoC, and apoH did not show significant gene silencing; however, four apoE isoforms showed improved luciferase silencing and the apoE-3 isoform increased cellular uptake and endolysosomal escape when added to cultured cells on treatment with CKK-E12 LNPs. Collectively, these data demonstrate that particle optimization can significantly improve specificity and efficacy, and improvements such as these offer the potential to broaden the therapeutic application of siRNA therapeutics. Further, the incorporation of apoE, instead of apoA-I into HDL-like particles appears to be superior in silencing gene targets in the liver [97]. ApoE-conjugated HDL-like NPs more efficiently delivered chol-siRNA to hepatocytes compared to apoA-I-conjugated particles, presumably due to the employment of a different uptake mechanism (LDL receptor for apoE vs. SR-B1 for apoA-I) [97].
In addition, Fischer et al. [98] explored the biodistribution, toxicity, and potential of human cloned apoE4 containing NPs to deliver a variety of different compounds, including cholesterol modified single stranded DNA (ss-DNA) [98]. The authors used a series of modifications to conjugate additional cargo to their apoE-HDL-like NPs, namely nickel-chelating lipids for binding His-tagged proteins, highly hydrophobic molecules like cholesterol for covalently linkage to cargo (like chol-ssDNA), and covalent conjugation of proteins to lipophilic moieties for binding to the lipid bilayer. The resulting suite of HDL-like NPs was stable in complex biological matrices and were shown to be non-cytotoxic in vitro at concentrations up to 320 mg/ml. Administration of the particles in vivo did not cause weight loss, organ specific toxicity, or overt immunogenicity. Biodistribution of the particles varied by route of administration, with preferred accumulation of the particles in kidney and liver after intravenous, intraperitoneal, intramuscular, or subcutaneous administration.
3.5 HDL-like Nanoparticles: Intrinsically Therapeutic Agents
More recently, HDL-like AuNP constructs have shown intrinsic therapeutic activity without requiring additional molecular drug cargo [90]. The HDL-like AuNPs are similar to the nanoparticles our group employed for cholesterylated nucleic acid formulation, as previously discussed. The structural conformation and number of apoA-I molecules, as well as phospholipid number, particle size, and negative particle charge are consistent with natural HDLs [91, 92]. Our data demonstrate that the HDL-like AuNPs directly compete with natural HDLs to target SR-B1, a high-affinity HDL receptor expressed by lymphoma cells. Uniquely, the AuNP template at the core of the HDL-like AuNP enables differential modulation of cholesterol flux compared with natural HDL. Data show that binding of SR-B1 and the manipulation of cholesterol homeostasis in B lymphoma cells upon treatment with the HDL-like AuNP leads to a selective induction of apoptosis. Furthermore, upon HDL-like AuNP treatment to mice bearing B-cell lymphoma xenografts, data show inhibition of B cell growth compared to mice treated with human HDL or saline. Collectively, these data suggest that HDL-like AuNPs may provide opportunities as stand-alone therapies due to cooperative SR-B1 targeting and manipulation of cellular cholesterol homeostasis in B cell lymphoma [90].
In addition to our findings, Zheng et al. found that their SR-B1-targeted HPPS NP inhibited motility and colony formation of nasopharyngeal carcinoma (NPC) cells [71, 99, 100]. The NPs were active in nude mice bearing subcutaneous tumors. This effect involved neither tumor cell necrosis nor apoptosis, suggesting an alternative tumor-shrinking mechanism that is related to HPPS-induced inhibition of NPC cell motility and colony formation. ApoA-I-mimetic peptides have been shown to exhibit antitumor activities by reducing plasma levels of lysophosphatidic acid, a stimulator of cell migration, invasion, and colony formation [101]. While apoA-I-peptide may be involved in the antitumor mechanism of HPPS, the exact mechanism is still unknown.
In conclusion, a significant body of evidence demonstrates that biomimicry may play an important role for next generation cancer therapies. Synthetic HDL-like NP mimics are appealing as new treatments, due to their inherent active targeting to cancer cells and their ability to deliver diverse therapeutic cargo. Importantly, synthetic HDL-like NPs can be manipulated such that they are able to bind and deliver small molecules, photothermal reactive agents, nucleic acids, and may also be stand-alone entities that are intrinsically active. Furthermore, HDL-like NPs can also serve as delivery vehicles for imaging agents. Research directed at these intriguing nanostructure conjugates has only begun. Further, preclinical and clinical development of these new approaches based on HDL biology may offer tremendous new therapeutic opportunities for patients with cancer.
References
1.
Camont L, Chapman MJ, Kontush A (2011) Biological activities of HDL subpopulations and their relevance to cardiovascular disease. Trends Mol Med 17(10):594–603. doi:10.1016/J.Molmed.05.013 CrossRefPubMed
2.
Xu S, Laccotripe M, Huang X et al (1997) Apolipoproteins of HDL can directly mediate binding to the scavenger receptor SR-BI, an HDL receptor that mediates selective lipid uptake. J Lipid Res 38(7):1289–1298PubMed
3.
Warnick GR, McNamara JR, Boggess CN et al (2006) Polyacrylamide gradient gel electrophoresis of lipoprotein subclasses. Clin Lab Med 26(4):803–846. doi:Doi 10.1016/J.Cll.2006.07.005
4.
Tabet F, Rye KA (2009) High-density lipoproteins, inflammation and oxidative stress. Clin Sci 116(1–2):87–98. doi:10.1042/Cs20080106 CrossRefPubMed
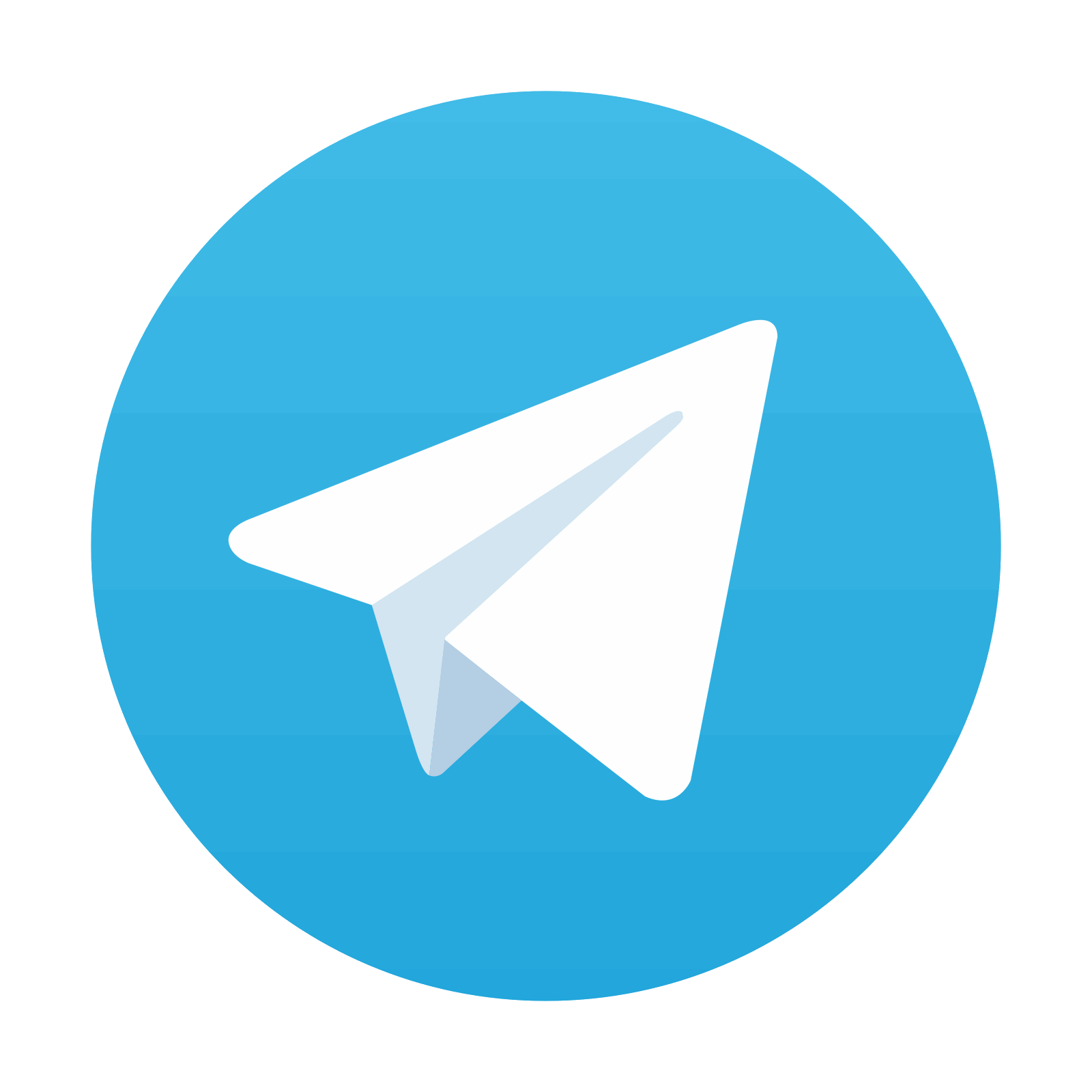
Stay updated, free articles. Join our Telegram channel

Full access? Get Clinical Tree
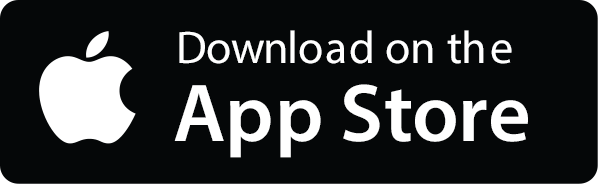
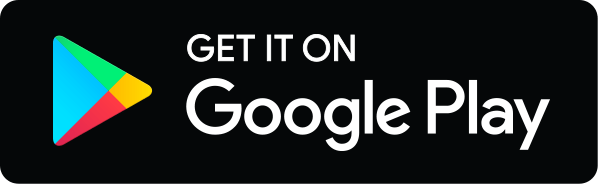