(1)
Medical Sciences Division Northern Ontario School of Medicine West Campus, Lakehead University, Thunder Bay, Ontario, Canada
Key Topics
Molecular pathology of hepatocellular carcinoma (HCC)
Circulating cell-free nucleic acids as HCC biomarkers
Circulating HCC epigenetic biomarkers
Circulating HCC genetic biomarkers
Circulating HCC miRNA biomarkers
Circulating HCC protein biomarkers
Circulating HCC cells
Key Points
HCC is the most common primary liver cancer. It is endemic in Asia and Africa due to increased prevalence of established risk factors in these geographic regions.
Serum biomarkers including AFP and DCP levels have proven useful in disease management, especially in prognostication and staging. Panel biomarkers hold potential for screening of the entire population at risk.
Alterations in HCC epigenome (e.g., methylated CDKN2A and CDH1), genome (e.g., TP53 mutations), miRNA (e.g., miR-122), as well as CTCs are detectable in circulation. The clinical potential of these circulating HCC biomarkers is being evaluated.
8.1 Introduction
Hepatocellular carcinoma (HCC) is the most common primary liver cancer. Globally, HCC ranks fifth in incidence and is a major cause of cancer-related deaths. In 2012, 782,000 new cases were estimated with 745,000 case fatalities in the world. Similar dismal outcome statistics apply to the US where 39,230 new cases and 27,170 deaths are expected in 2016. Thus, similar to lung cancer, the incidence mirrors the mortality rates, suggestive of the need for early detection and effective treatment. Validated biomarkers in body fluids should help revert this dismal statistics. The majority of HCCs (~80 %) occurs in Eastern and Southeastern Asia (where over half of the cases are diagnosed) and sub-Saharan Africa, due to the increased prevalence of known risk factors in these regions. The incidence is equally rising in the Western world due to the increasing incidences of hepatitis C viral (HCV) infections. The established risk factors for HCC include chronic hepatitis B and C viral infections, cirrhosis of any cause, and high-level exposure to aflatoxin-b1 (AFB1). Additional factors that modestly elevate the risk include diabetes, nonalcoholic fatty liver disease, obesity, and smoking.
Liver resection and transplantation are useful treatment strategies for tumors detected early, while advanced stage disease patients are subjected to chemotherapy and other targeted therapies. The 5-year survival rate for patients with HCC in general is ~40 %, but when detected early, this can be as high as 60–70 %. Hence the need for screening programs, especially in endemic areas. Imaging modalities (US, CT, MRI) are generally used in the Western world for HCC screening. However, serum biomarkers that offer a cost-effective means of early detection are the screening methods of choice in Asia. But the alpha-fetoprotein (AFP) serum biomarker for HCC suffers from sensitivity and specificity. Even when combined with des-γ-carboxyprothrombin (DCP) in a panel, sensitivity ranges from 48 to 94 % with equally variable specificities of 53–99 % for early HCC detection. The need for more accurate early detection biomarkers in body fluids is obvious and being pursued.
8.2 Screening Recommendations for HCC
Screening for HCC is not recommended for the general population, even in regions such as Asia with higher incidences (up to 80/100,000 in China) than other parts of the world. The NCI notices that screening of people at elevated risk does not reduce mortality and also observes that invasive procedural screening such as needle aspiration cytology may carry risks such as bleeding, pneumothorax, and bile peritonitis. Surveillance is however recommended for people with HBV infection or carriers, HIV coinfection, or cirrhosis of any cause. Surveillance is not recommended for HCV-infected people except in the presence of cirrhosis or coinfection with HBV or HIV. For this population at elevated risk above average, 6–12 monthly abdominal ultrasound and serum AFP assay are a cost-effective means of early cancer detection.
8.3 Molecular Pathology of HCC
Primary liver cancer can originate from any cellular components of the liver. However, HCCs, which are cancers of hepatocyte origin, constitute 85–90 % of all liver cancers. The next in frequency are intrahepatic cholangiocarcinomas (5–10 %) that arise from the cholangiocytes lining bile ductules in the liver. Constituting <5 % of cancers of the liver are hepatocellular adenomas, hepatoblastomas, epithelioid hemangioendotheliomas, and fibrolamellar hepatocellular angiosarcomas. The best-studied molecular pathology pertains to primary HCC.
The risk factors of HCC implicate an inflammatory process in its pathogenesis. Consistent with inflammatory-mediated diseases, reactive oxygen and nitrous species, as well as cytokine excess can damage hepatocytes with subsequent regeneration. Chronically damaging events to cells can result in epigenetic and genetic alterations leading to oncogene induction and/or tumor suppressor gene inactivation. These events in turn can impinge upon at least four important signaling pathways (WNT, TGFβ, PI3K/AKT, and RAF/MEK/ERK) implicated in HCC progression.
8.3.1 Specific Genetic and Signaling Pathway Alterations in HCC
Of importance in hepatocellular carcinogenesis are chromosomal and microsatellite alterations. Chromosomal losses at 1p, 4q, 6q, 8p, 9p, 13q, 16q, 16p, and 17p, as well as gains at 1q, 6p, 8q and 17q, characterize HCCs. These chromosomal regions harbor tumor suppressor genes such as RB, TP53, CDKN2A, and IGF2R and oncogenes including CTNNB1 that play important roles in the pathogenesis of HCC.
The epigenome is equally deregulated in HCC, and this is an early event in tumor progression. Altered promoter methylation, histone modifications, and miRNA expression underlie disease progression. Promoter hypomethylation and activation of oncogenes, as well as hypermethylation and silencing of tumor suppressor genes, are established in HCC, as in other cancers. PTEN, RB, and CDKN2A are inactivated in HCC partly via promoter hypermethylation. Also hypermethylated are BMP4, SPDY1, DAB2IP, GSTP1, FZD7, ZFP41, APCNFATC1, and RASSF1A that are established and putative tumor suppressor genes. Promoter hypermethylation of DCC, CSPG2, and NAT2 is associated with HBV-mediated HCC. PAX4, SCGB1D1, and WFDCG promoters are hypomethylated in HCC. Of interest, DNA methyltransferase (DNMT) enzymes are overexpressed early in HCC development, underscoring the early gene methylation status in this cancer.
In addition to mutations, epigenetic and chromosomal alterations, liver cancer cells express and respond to several growth factors including EGF, FGF, PDGF, IGF, and VEGF that act on receptor tyrosine kinase receptors to induce various signaling pathways to sustain malignancy and promote invasiveness. Thus, growth factor signaling is associated with proliferation, invasiveness, portal thrombosis, and increased neovascularization, which is a characteristic of HCC.
8.3.1.1 RAS-MAPK Pathway Alterations in HCC
The RAS/RAF/MEK/ERK pathway plays important roles in liver cancer development. Similar to many signaling networks, this pathway is activated in HCC through diverse mechanisms. HBV protein HBx and HCV core protein can activate this pathway. Downregulation of RKIP and RAS inhibitors, SPRED1 and SPRED2 in HCC is associated with pathway activation as well. Besides, growth factor signaling plays a role in activating this pathway. Not surprisingly, almost all HCCs have the RAS/RAF/MEK/ERK pathway engaged, although mutations in RAS (except NRAS, which is mutated in ~30 % of liver cancers) and RAF are infrequent in HCC. The importance of this pathway in HCC has led to its exploration as a therapeutic target, with the pathway inhibitor, sorafenib (Nexavar®), indicated for the treatment of patients.
8.3.1.2 PI3K/AKT Pathway Alterations in HCC
The PI3K pathway is activated in HCC via multiple mechanisms. In addition to mutations in genes of this pathway and growth factor interactions with RTK receptors, inactivation of PTEN occurs in many HCCs. LOH at the PTEN locus occurs in about 40–60 % of HCCs, and PTEN is also silenced via promoter hypermethylation. AKT activation as a consequence of loss of PTEN function enables the acquisition of aggressive tumor phenotypes.
8.3.1.3 Alterations in Cell Cycle Regulators in HCC
TP53, CDKN2A, and RB are important regulators of the cell cycle, and they are all deregulated in subsets of HCC. Aflatoxin-b1 (AFB1) in grains and peanuts is an established mutagen of TP53. Specifically, this toxin causes G:C to T:A transversions at base 3 in codon 249 leading to the substitution of arginine for serine (R249S). Additionally, TP53 is a target of viral oncoproteins, especially HBV-encoded HBx, and hence mutation rate accelerates with viral hepatitis. Promutagenic N7-deoxyguanosine adduct formation from AFB1 mediates the G:C to T:A transversion mutation. In non-AFB1-mediated HCC, TP53 mutations occur in later stages of the disease. For example, TP53 mutations and LOH occur in moderately to poorly differentiated HCC of non-AFB1 origin, and this is not a feature of precursor lesions or AFB1-induced HCC. In addition to its association with aggressive tumors with poor prognosis, TP53 R249S causes reprogramming of terminally differentiated cells into acquisition of stem cell-like phenotypes. Several other members of the cell cycle are deranged in HCC. RB is inactivated in about 28 % of HCCs. CDKN2A expression is lost in ~34 % of HCC, while overexpression of CCND1 and CDK4 occurs in a majority (58 %) of these cancers. Additionally, CCNE1 is a possible target of the dsRNA-binding protein and nuclear factor 90 (NF90), both of which are elevated in HCC. These changes serve to propel the cell cycle through G1/S phase transit.
8.3.1.4 WNT/β-Catenin Pathway Alterations in HCC
Aberrant WNT/β-catenin pathway activation due to altered expression of pathway members as a consequence of epigenetic modifications, mutations, and deletions plays an important role in HCC progression. Pathway ligands, receptors, and antagonists, among other players, are deregulated in HCC, and some of these alterations occur early in precursor lesions. The WNT/β-catenin pathway gene most mutated (at a frequency of ~33 %) in HCC is CTNNB1. In addition to activating mutations, CTNNB1 is a target of some miRNAs. For example, miR-214 targets this gene through EZH2 to activate the WNT/β-catenin pathway in HCC. Also mutated in HCC is AXIN, a tumor suppressor gene that regulates the degradation of β-catenin. AXIN mutations occur in about 6.2 % of HCCs, and these are late events, being almost absent in precursor lesions. APC mutations are rare, but epigenetic silencing is an important event in HCC. APC loss of function leads to lack of β-catenin phosphorylation and hence its accumulation and pathway activation. Aberrant expression is also observed in the WNT/β-catenin pathway receptors, agonists, and antagonists. The WNT/β-catenin pathway receptors, FZD3, FZD6, and FZD7, as well as co-receptor, LRP6, are all upregulated in HCC. Similarly, canonical pathway members, WNT1 and WNT3, are overexpressed, while noncanonical WNT5a and WNT11 are downregulated in HCC. The downregulation of several WNT/β-catenin pathway antagonists including SFRPs (via methylation), DKKs, and WIF1 is noted in HCC. Importantly, some of these alterations occur early in HCC. Another important aspect of WNT/β-catenin pathway activation is its involvement in EpCAM+ (progenitor cell-type) HCCs.
8.3.1.5 TGFβ Pathway Alterations in HCC
The TGFβ signaling pathway controls multiple cellular activities including proliferation, growth, differentiation, apoptosis, extracellular matrix remodeling, and general cellular homeostasis. The signaling output tends to be cell-type dependent. For example, signaling in fibroblast may lead to proliferation, cytokine secretion, and extracellular matrix production, while in epithelia, apoptosis, cell cycle arrest, and adhesion may occur, and yet in endothelium, cellular proliferation, growth, migration, and morphogenesis may be triggered. The role in liver physiology and pathology may be dual and context dependent. Indeed, the TGFβ pathway mediates all stages of chronic liver pathology, from initial injury, inflammation, fibrosis, cirrhosis, to eventual development of HCC. One role of the TGFβ pathway is in controlling liver volume, by inducing cytostasis and cellular apoptosis. On the contrary, liver damage induces increased TGFβ levels leading to liver cell damage and repair. Loss of this signaling pathway, however, causes increased hepatocyte proliferation and neoplastic transformation. Thus, TGFβ performs a tumor-suppressive function during early stages of liver damage to facilitate regeneration. On the other hand, during hepatocellular carcinogenesis, there may be a switch to enhance tumor progression to invasion and metastasis. These “fluid” roles of the pathway are evidenced in the complex of its interactome, which enables switches in receptor types and deployment of other signaling pathways including JNK signaling. This complexity has made therapeutic targeting of this pathway a formidable task. Normal hepatocytes are devoid of active TGFβ, but levels are detectable with acute liver injury and increase progressively mirroring the severity of liver damage. This increase is measurable in serum and can be used to monitor or detect live disease.
8.3.2 Molecular Subtypes of HCC
Primarily based on gene expression data, molecular subtypes of HCC have been identified and proven to have various clinical implications. In 2004, Lee et al. delineated two distinct types of HCC using gene expression signatures, and these distinct groups had different prognosis [1]. The group associated with poor prognosis had increased expression of genes involved in cell proliferation (PCNA, CDK4, CCNB1, CCNA2, and CKS2), prevention of apoptosis (PTMA/ProT), epigenetic histone modifications (HRMT1L2, which encodes a histone H4-specific methyltransferase), ubiquitination (UBE2D), and resistance to hypoxic conditions (HIF1α). This group was also enriched for AFP-positive tumors that already confer poor survival outcomes.
Another gene expression profiling placed all HCCs into six subgroups. However, based on chromosomal alterations, these six subgroups could indeed be subclassified into just two distinct categories. The first group (1–3) exhibits chromosomal instability, while the second group (4–6) harbors mostly normal chromosomes [2]. The characteristics of the first group are absence of TP53 mutations and presence of AXIN1 mutations in association with increased expression of imprinted genes. Moreover, this group of tumors is associated with low copy number HBV infections. The second group has increased LOH, presence of TP53 and AXIN1 mutations, as well as high copy number HBV infections.
Yamashita et al. also dichotomized HCCs based on expression of EpCAM and its related genes, as well as hepatic stem cell marker expressions [3]. The EpCAM-negative tumors phenotypically resembled mature hepatocytes. However, tumors positive for EpCAM were of hepatic progenitor cell type with possible tumor-initiating abilities. Additionally, EpCAM-positive cancer cells expressed stem cell markers such as C-KIT and CK19 and had WNT/β-catenin pathway activation. Incorporating the presence or absence of AFP enabled the subclassification of these tumors into four groups with prognostic implications. The EpCAM-positive/AFP-negative (type A) tumors were associated with good prognosis; EpCAM-negative/AFP-negative (type D) tumors were of intermediate prognosis, while the worse prognosis was among patients with EpCAM-positive/AFP-positive (type B) and EpCAM-negative/AFP-positive (type C) tumors.
8.4 Circulating HCC Biomarkers
Circulating biomarkers of HCC are continuously being explored. While traditional serum biomarkers remain the mainstay of HCC detection and management, the potential of detecting tumor-specific epigenetic and genetic changes in ctDNA cannot be overlooked. Thus efforts are directed toward uncovering the clinical utility of these biomarkers. Additionally, the promise of miRNA, and the prognostic roles of circulating HCC cells need coordinated validation work.
8.4.1 Circulating Cell-Free Nucleic Acid Content as HCC Biomarkers
Circulating cell-free DNA (ccfDNA) is elevated in patients with HCC, and this may have diagnostic, staging, and prognostic utility. Using a genetic approach, Jiang et al. demonstrated elevated ccfDNA in HCC patients compared to controls [4]. Cancer patients harbored higher levels of shorter DNA molecules that preferentially carried tumor-associated copy number aberrations. Circulating mtDNA was also elevated in HCC patients, and these molecules were much shorter than circulating cell-free nuclear DNA. CcfDNA was elevated in 56.4 % of HCC patients compared to 4.4 % of healthy controls [5]. In another series, ccfDNA levels did not correlate with AFP and l-fucosidase, and the combination of the three biomarkers elevated the sensitivity from 56.4 to 89.7 % for HCC detection. CcfDNA is elevated in people with benign hepatic conditions such as cirrhosis and chronic hepatitis. The diagnostic performance for differentiating HCC patients from those with benign conditions achieved a sensitivity, specificity, and AUROCC of 91 %, 43 % and 0.69, respectively. Additionally, high ccfDNA levels were associated with shorter survival [6]. Chen et al. targeted two DNA fragments (100 bp and 400 bp) in β-actin gene to determine ccfDNA levels and to develop a DII assay for HCC [7]. Elevated DNA levels significantly differed between cancer and healthy control individuals but not those with HBV infection. However, DII was significantly higher in HCC patients than HBV-infected individuals and healthy controls and was thus of much diagnostic utility. DII was associated with tumor size and metastasis. CcfDNA (median 173 ng/ml) levels were significantly higher in cancer patients than healthy controls (median 9 ng/ml) and people with cirrhosis and chronic hepatitis (median 46 ng/ml) [8]. The AUROCC was 0.949 and 0.874, respectively, for differentiating HCC patients from healthy controls and those with benign liver conditions. At a cutoff value of 18.2 ng/ml, ccfDNA had a sensitivity and specificity of 90.2 % and 90.3 %, respectively, for HCC detection. This improved to diagnostic accuracy with AUROCC of 0.974 (95.1 % sensitivity and 94.4 % specificity) when combined with serum AFP levels. As a prognostic biomarker, elevated ccfDNA levels were associated with tumor size, intrahepatic spread, vascular invasion, and shorter survival.
A meta-analysis was performed on the quantitative (seven studies) and qualitative (15 methylation analysis) studies of ccfDNA in HCC detection. These studies included 2424 subjects of which 1280 were cancer patients [9]. The pooled sensitivity, specificity, PLR, NLR, DOR, and SAUROCC were 74.1 %, 85.1 %, 4.97, 30.4 %, 16.347, and 0.86, respectively, for the quantitative studies and 53.8 %, 94.4 %, 9.545, 49.0 %, 19.491, and 0.87, respectively, for the qualitative methylation studies. With the addition of AFP levels, the overall performances of both quantitative and qualitative studies increased with a DOR of 106.27 and SAUROCC of 0.96. Thus, ccfDNA has potential diagnostic applications and can augment the performance of AFP in HCC surveillance in high-risk individuals.
8.4.2 Circulating HCC Epigenetic Biomarkers
Genes found methylated at various frequencies in circulation of patients with HCC include CDKN2A (most frequently studied), RASSF1A, CDH1, RUNX3, ZFP41, DAB21P, BMP4, and SPDY1. Hypomethylation of PAX4, ATK3, CCL20, WFD6, and SCGB1D1 in circulation of HCC patients has also been demonstrated.
Wong et al. examined CDKN2A methylation in tissue and plasma samples from HCC patients [10]. CDKN2A was methylated in 73 % of tissue samples, and these methylation patterns were detected in 81 % of paired plasma and serum samples. Promoter methylation of CDKN2B was demonstrated in 64 % of HCC and 25 % of plasma and serum samples as well. Either CDKN2B or CDKN2A methylation was positive in 48 % of tumors and in 92 % of plasma/serum samples. All available buffy coat samples were positive for CDKN2B methylation, indicative of possible contribution from CTC presence. Of the patients with positive serum/plasma samples, concurrent methylations in tumors were demonstrated in 75 % of patients who had metastatic or recurrent disease. Collectively, ccfDNA and CTCs were detected in 87 % of the 92 % who had CDKN2B and CDKN2A methylation [11]. CDKN2A methylation in plasma/serum and blood cells collected pre-, intra-, and post-operatively from patients with HCC was studied for its diagnostic utility. CDKN2A was methylated in 80 % of peripheral circulation (i.e., plasma/serum or blood cells) and decreased 12- to 15-fold after surgery [12]. Methylation of CDKN2A in sera from 23 patients with cirrhosis and 46 HCC patients was studied, and methylation was demonstrable at a frequency of 47.8 % and 17.4 % of samples from patients with HCC and cirrhosis, respectively. Of note, these methylation patterns had no association with AFP levels, indicating a possible complementary use [13].
Tan et al. studied gene methylation in serum samples to uncover tumor-specific biomarkers of multiple cancers [14]. Promoter methylations of four tumor suppressor genes (CDKN2A, RASSF1A, CDH1, and RUNX3) were demonstrated in metastatic breast cancer, NSCLC, gastric, pancreatic, colorectal, and liver cancers. Hypermethylation of at least one of these genes was found in 88.6 % of serum samples from cancer patients, with the following frequencies in the different cancers: 80 % of metastatic breast cancers, 95 % of NSCLC, 82.4 % of colorectal cancer, and all of the limited gastric, pancreatic, and liver cancer samples. This gene panel appears useful as noninvasive screening for cancer detection in general. However, the most frequently methylated (62.9 %) gene in circulation of cancer patients was RUNX3 [14]. In a study whereby plasma samples from HCC patients were subjected to genome-wide methylation analysis, the genes frequently hypermethylated were CDKN2A, ZFP41, DAB21P, BMP4, and SPDY1, while PAX4, ATK3, CCL20, WFD6, and SCGB1D1 were mostly hypomethylated [15].
Although no strong diagnostic association is made in these pilot studies, the potential of using circulating methylation biomarkers in HCC patient management exists should in-depth analysis be performed using sensitive technologies. Thus, Wen et al. developed a methylated CpG tandem amplification and sequencing (MCTA-Seq) method that could detect thousands of promoter hypermethylation simultaneously using just 7.5 pg of ccfDNA [16]. This approach enabled detection of gene methylations in plasma from patients with small tumors (≤3 cm) and even those negative for AFP serum assay. Genes of interest in HCC diagnostics detected by this method include RUNX2, VIM, RGS10, and ST8SIA6. Similarly, targeted deep methylation analysis of plasma ccfDNA using massively parallel semiconductor sequencing enabled detection of methylation in H19, IGF2, VIM, and FBLN1 in cancer patients [17].
8.4.3 Circulating HCC Genetic Biomarkers
8.4.3.1 Circulating Mutated Genes as HCC Biomarkers
Mutations in TP53 are detected in clinical samples from patients with HCC. It appears that the TP53 codon 249 mutation is a tumor marker, and its detection in plasma from patients with liver cirrhosis predates the development of liver cancer by several months. Plasma and tissue analyses of TP53 mutations show concordance in over 50 % of cases, indicating its usefulness as a noninvasive biomarker for HCC.
In endemic areas such as sub-Saharan Africa and China, TP53 ser249 mutations in plasma are strongly associated with HCC. Its frequency is lower in samples from healthy controls compared to patients with liver cirrhosis and much higher in patients with HCC [18]. Using a more sensitive method for mutation detection in plasma (short oligonucleotide mass analysis – electrospray ionizing mass spectrometry), Jackson et al. were able to detect this mutation in plasma from >50 % of patient samples [19]. Four plasma samples in this series that tested positive for mutations had no associated tissue mutation (another example of tissue heterogeneity that is overcome by body fluid analysis). In another study, this mutation was found in over 46 % of post-diagnostic patient plasma samples and was demonstrable in plasma over a year prior to diagnosis in some cases, suggesting its noninvasive use in early detection of HCC, at least in endemic areas. Szymanska et al. detected this mutation at a similar high frequency of 64 % in pre-diagnostic plasma using the short oligonucleotide mass analysis, confirming this method as useful for such analysis [20]. The circulating levels has strong concordance (88.5 %) with tissue presence [21], indicative of its applicability as an authentic biomarker for patient management.
8.4.3.2 Circulating MSA as HCC Biomarkers
Loss of tumor suppressor genes (TSGs) on chromosome 8p is associated with HCC progression and metastasis. The metastasis suppressor, HTPAP-1, for instance, is strongly associated with HCC metastasis and prognosis [22]. Another potential TSG located on 8p22 that may be involved in hepatocellular carcinogenesis is deleted in liver cancer 1 (DLC1).
An initial study of LOH using three chromosome 8 markers (D8S277, D8S298, and D8S1771) found a high concordance rate between tissue and plasma MSA. In considering all three loci, concordance was 73.3 %, indicating plasma MSAs are of HCC origin [23]. In a follow-up study, the three chromosome 8 markers were used for LOH detection in plasma from HCC patients as a biomarker of clinical disease course. LOH at one or more loci was observed in 58.1 % of plasma samples and was associated with intrahepatic metastasis but not other clinical parameters studied, including HBsAg, cirrhosis, AFP levels, tumor size, and differentiation [24]. Chromosome 8 deletions were however shown to be associated with metastasis, and whether these changes could be assayed in plasma was questioned. Other chromosome 8 markers (D8S258 and D8S264) have been used for analysis of MSA. The levels of ccfDNA in plasma were associated with tumor size, TNM stage, and poor 3-year DFS and OS. Additionally, allelic imbalance (AI) at D8S258 was associated with tumor differentiation, TNM stage, vascular invasion, and poor 3-year DFS and OS. The presence of both high circulating DNA levels and AI at D8S258 in the same patient was associated with an even much dismal 3-year survival outcome [25].
8.4.4 Circulating HCC Coding RNA Biomarkers
HTERT and TGFβ1 transcripts have been investigated as circulating biomarkers of HCC. Miura et al. aimed at identifying a biomarker for early detection of HCC that could potentially detect small tumors usually undetectable by abdominal ultrasound and other conventional tumor markers [26]. Their initial study focused on evaluating the clinical value of hTERT mRNA assay in serum of patients with HCC. Cancer, chronic hepatitis, and cirrhotic patient sera, as well as sera from healthy control individuals, were assayed. Nearly 90 % of cancer, 70 % of cirrhotic, and 41.7 % of chronic hepatitis patient samples had detectable hTERT message in circulation, compared to none in healthy controls. Multivariate analysis showed an independent association of circulating hTERT transcripts with AFP positivity, tumor size, and degree of differentiation [26]. In a follow-up study, this group developed a quantitative method to measure hTERT mRNA levels in serum. Serum hTERT mRNA was much higher in HCC patient samples than those from people with chronic liver diseases. For detection of HCC, serum hTERT mRNA levels performed at a sensitivity of 88.2 % and a specificity of 70 %, which is much superior to other tumor markers such as AFP levels, AFP mRNA, and des-gamma carboxyprothrombin (DCP). In multivariate analysis, hTERT mRNA levels independently correlated with tumor size and degree of differentiation as noted in previous studies [27]. In yet another study by this group, a large-scale multicenter validation study of serum hTERT mRNA for HCC detection and related clinical value was undertaken. Serum hTERT mRNA was detected in samples from HCC patients and achieved a sensitivity of 90 % and a specificity of 85.4 %, which again was much superior to other HCC biomarkers (AFP, AFP-L3, and DCP). Serum hTERT transcript detection was also superior to the conventional markers used for the detection of small curable tumors. In a multivariate analysis, hTERT mRNA had significant independent correlation with tumor size, and differentiation status, confirming previous findings by these investigators [28].
Transcripts of TGFβ1 and protein levels in peripheral blood were assayed in relation to HBV status and their potential utility for HCC diagnosis [29]. Both tissue and blood samples were available for study. TGFβ1 expression was present in 83.3 % of HCC and 43.3 % of adjacent noncancerous tissues (evidence of field cancerization). This finding was also more associated with HBV DNA-positive (94.7 %) than HBV DNA-negative (63.6 %) status. There was also a relationship of TGFβ1 expression with the degree of differentiation and HBV replication. The expression of TGFβ1 (both mRNA and protein) in circulation was significantly higher in patients with HCC than those with benign liver diseases. At levels >1.2 ug/L cutoff, TGFβ1 levels detected HCC at a sensitivity of 89.5 % and specificity of 94 %. Together with AFP, sensitivity increased to 97.4 %.
8.4.5 Circulating HCC Noncoding RNA Biomarkers
MicroRNAs play important roles in liver carcinogenesis, as oncomirs, tumor suppressormirs, and miRNAs that coordinate or modulate viral risk factor events. Oncomirs are upregulated in HCC, and these serve to target and degrade established tumor suppressor genes. Many miRNAs are upregulated in HCC, but miR-21 and miR-221/222 homologues have such critical roles in HCC. MiR-21 is upregulated in many cancers including HCC. This miRNA targets programmed cell death 4 (PDCD4) and induces EMT via AKT and ERK pathway signaling. The highly homologous miR-221 and miR-222 are upregulated in HCC and also target PTEN/AKT signaling of the PI3K pathway to enhance tumor progression. Noteworthy, numerous oncogenic miRNAs of HCC are uncovered with target genes that include MYC, BCL2, BIM, E2F, CCND1, CDKN1B, CDKN1C, RHOA, and TLR. Several miRNAs are also downregulated in HCC, and these have tumor suppressor functions. MiR-99a-1, miR-199a-2, and miR-199b family target HIF1α, mTOR, and MET to reduce liver cancer cell proliferation, resistance to hypoxia, and enhanced invasive capacity. Loss of miR-122 in model organisms leads to liver inflammation, fibrosis, and development of HCC-like tumors. MiR-122 targets several genes including CCNG1 with subsequent alterations in p53 tumor suppressor functions.
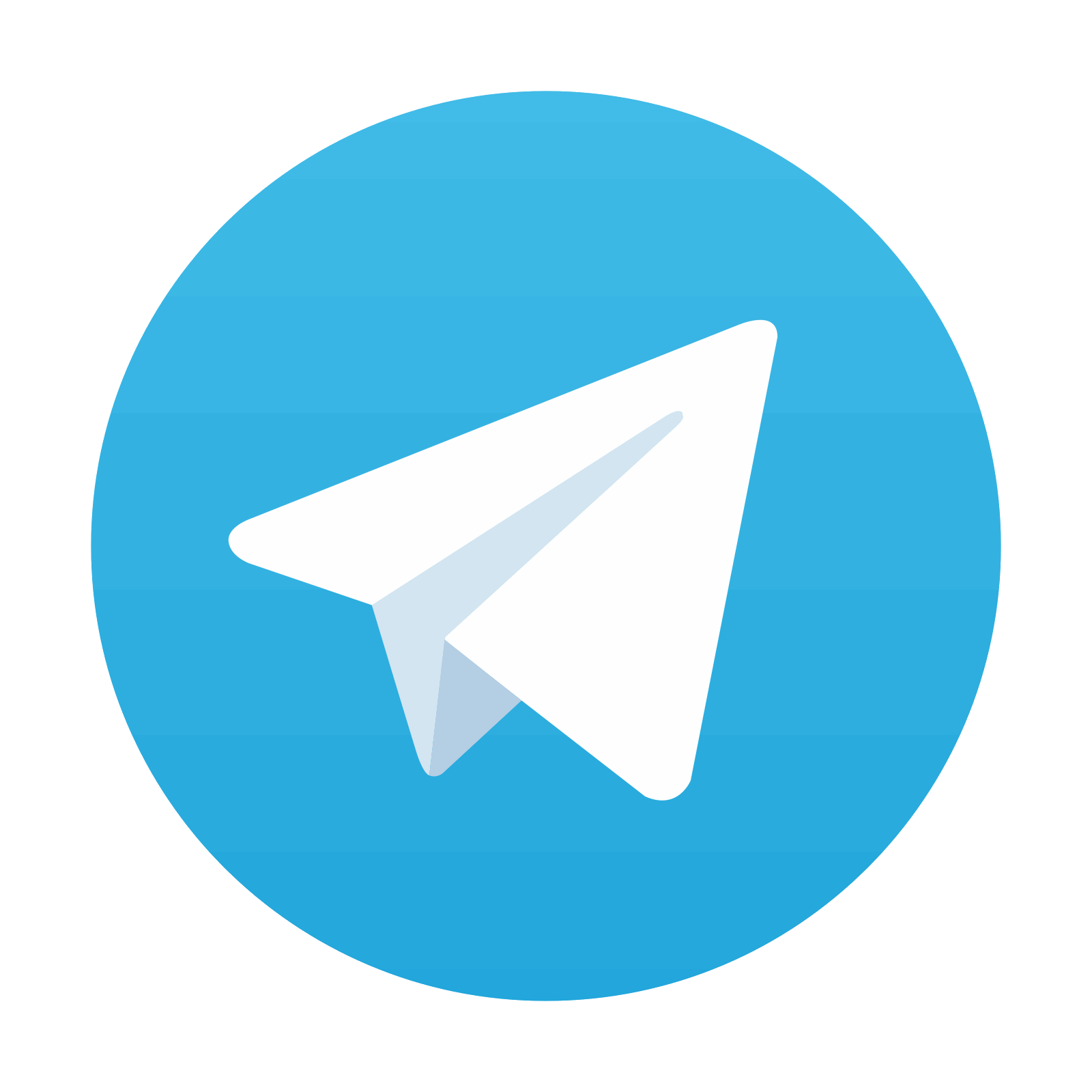
Stay updated, free articles. Join our Telegram channel

Full access? Get Clinical Tree
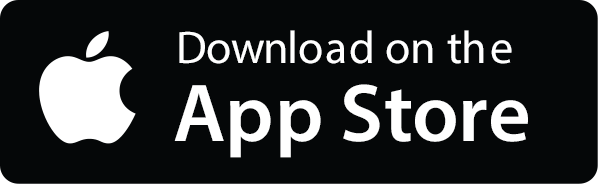
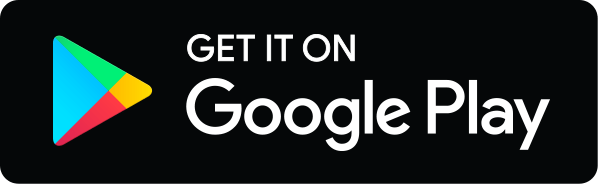