Hematopoietic cell transplantation
Roy Jones, MD, PhD Elizabeth Shpall, MD
Richard Champlin, MD
Overview
Hematopoietic cell transplantation is an effective therapy for a variety of hematologic malignancies and selected other cancers, immunodeficiency states, and genetic disorders. Refinement of methods has allowed the application of this technique to older patients as well as the use of human leukocyte antigen mismatched donors and products. While toxic, the majority of these treatments have curative potential for otherwise fatal cancers. The immunotherapeutic activity of allotransplantation provides both therapeutic benefit and lessons for future immune treatments of cancer.
Hematopoietic cell transplantation involves engraftment of stem cells that can be collected from the bone marrow, peripheral blood, or umbilical cord blood (UCB). Allogeneic transplants are obtained from another individual. Autologous transplants involve use of a patient’s own hematopoietic cells, usually after cryopreservation. Syngeneic transplants are between genetically identical twins.
Hematopoietic transplantation is an effective treatment for many life-threatening hematologic, immune, metabolic, and neoplastic diseases (Table 1).1, 2 The basis for autologous hematopoietic cell transplantation is to use patient-derived hematopoietic stem cells to prevent otherwise lethal myelosuppression from intensive treatment. More recently, autologous cells manipulated in vitro to produce augmented immune effects are being explored. The basis of allogeneic hematopoietic transplantation is the engraftment of donor-derived stem cells; in the recipient, these cells reconstitute hematopoiesis and immunity. Following successful transplantation, recipients are considered chimeras with hematopoietic and immune cells derived from the donor. Recently, it has been recognized that hematopoietic stem cells are capable of limited differentiation into nonhematopoietic tissues4 including mesenchymal cells,5, 6 liver,7, 8 cardiovascular tissues,9 and, possibly, neural tissue.10 There is considerable interest in stem cell transplantation for restoration of damaged or diseased organs and tissues.11, 12
Table 1 Diseases treated with hematopoietic transplantation
Malignant
|
Nonmalignant
|
Methods of transplantation
In most cases, transplanted hematopoietic cells are infused following intensive chemotherapy, irradiation, or other therapies such as antibodies. Over time, a variety of chemotherapeutic agents has been explored for use in transplantation, emphasizing the alkylating agents cyclophosphamide, busulfan, melphalan, thiotepa, and carmustine (BCNU). Total body radiation (TBI) is also employed. In allogeneic transplantation, these drugs are often combined with agents designed to produce additional tumor killing and immunosuppression, such as fludarabine.13
The existence of graft-versus-malignancy (GVM) effect is now well-established as an important component of therapeutic activity for allotransplantation.14 This alloimmune effect is often associated with normal tissue toxicity and termed graft-versus-host disease (GVHD). A major focus of allotransplant research is the exploration of specific cell types and techniques that might produce GVM without GVHD.15 Donor lymphocyte infusions (DLI) following transplantation can be used to augment the GVM effect.16
Hematopoietic transplantation as treatment for malignancies
Most hematopoietic transplants have been performed for treatment of hematologic malignancies.17 Many chemotherapy drugs (predominantly alkylating agents18) and irradiation produce a dose-dependent antitumor response. Myelosuppression is the dose-limiting toxicity of many chemotherapeutic agents and whole-body irradiation. Doses of many agents can be escalated three- to fivefold above conventional maximally tolerated dose if followed by autologous or allogeneic transplantation to restore hematopoiesis (Figure 1).
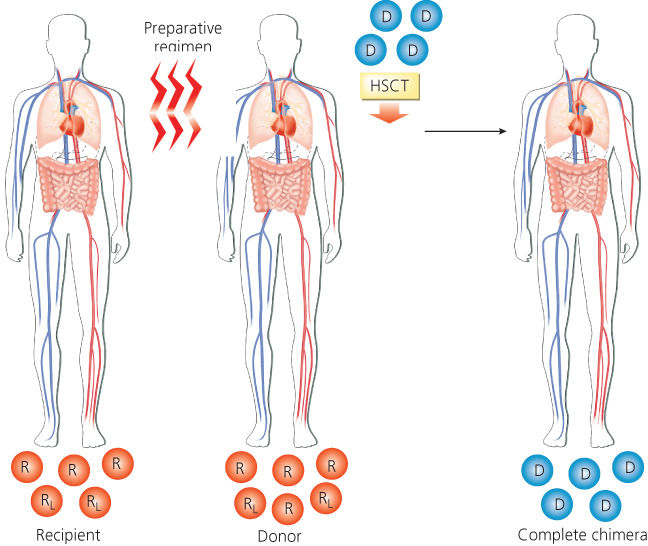
Figure 1 Scheme of allogeneic hematopoietic transplantation. The recipient receives a myeloablative preparative regimen designed to eradicate malignant and normal cells. The recipient then receives donor hematopoietic cells that restore hematopoiesis. Abbreviations: D, donor hematopoietic cells; HSCT, hematopoietic stem cell transplant; R, recipient’s normal bone marrow cells; RL, recipient’s leukemia cells in bone marrow.
Autologous transplantation
As a general rule, autologous hematopoietic transplantation is most effective in patients with malignancies shown to be responsive to conventional treatment immediately before the transplant regimen.19 Factors known to predispose to treatment failure include higher volume residual tumor,20, 21 tumor types not known to exhibit a steep dose-killing relationship,22 or treatment of patients at high risk for toxicities from the proposed treatment regimen.
Immune augmentation strategies used in the posttransplant setting are being actively explored to avoid the prolonged immunosuppression that follows transplantation and may increase the risk of relapse.23 An additional factor that might predispose to relapse is contamination of the harvested cells with tumor. Although limited investigation using gene-marked tumor cells has shown that cryopreserved tumor cells can engraft and contribute to relapse, the clinical importance of this phenomenon is unclear.24 Malignancies shown to be cured by autologous hematopoietic cell transplantation include intermediate grade and other selected lymphomas,25 Hodgkin’s disease,26 acute lymphoblastic leukemia,27, 28 and acute myelogenous leukemia (AML).29, 30 Although not proven to be curative, major improvement in survival in myeloma patients receiving autologous transplantation has made the technique a standard treatment for appropriate patients with this condition.31, 32 Benefit is likely for patients with germ cell cancers,33 low grade lymphomas,34 and a variety of pediatric solid tumors.35
Allogeneic transplantation
Allogeneic hematopoietic transplants combine cytotoxic treatments with the immune-mediated GVM effect. Donor-derived lymphoid cells may react against and eradicate malignant cells that survive high-dose cytotoxic therapy.36, 37 Patients with acute or chronic GVHD have reduced risk of relapse, suggesting a relationship between GVM and GVHD.38, 39 The most direct evidence for GVM comes from the observation that DLI can reinduce remission in patients who relapse posttransplant.40, 41
There are major differences among malignancies in their susceptibility to GVM effects. Indolent myeloid and lymphoid malignancies are highly sensitive to this process, as evidenced by durable remissions after modulation of immunosuppression or DLI in patients who have relapsed after an allogeneic transplant. GVM effects have been best documented in patients with chronic myelogenous leukemia (CML).
GVM effects also occur but with lesser intensity against other hematologic malignancies including AML, multiple myeloma (MM), Hodgkin’s disease, and intermediate-grade lymphoma. In these diagnoses, allogeneic transplants produce a greater frequency of durable remissions than syngeneic or autologous transplants, but these disorders respond less frequently to DLI and responses are usually transient. Acute lymphoblastic leukemia and high-grade lymphoma appear relatively insensitive to graft-versus-leukemia (GVL) effects,42 although patients with GVHD do have a reduced risk of relapse in some studies.43
GVM effects may be directed against a number of potential target antigens. Development of methods to separate GVM effects and GVHD and generate antigen-specific antitumor responses is a major goal of ongoing research. For example, natural killer (NK) cells may produce GVM with decreased risk of GVHD,44 and regulatory T cells (Treg) may moderate GVHD with lesser effects on GVM.45
Donor selection
Autologous transplantation
Autologous transplants use the patient as their own donor. In the closely related special case, syngeneic transplants are donations from a genetically identical twin. Autologous transplants require no histocompatibility matching but often come from patients who have received extensive prior chemotherapy. This treatment often compromises marrow stem cell function making adequate stem cell collection more difficult. Stem cells can be collected from the peripheral blood following mobilization with hematopoietic stimulants [G-CSF (granulocyte colony-stimulating factor), chemotherapy] or from bone marrow. A minimum of 2 × 106 CD34 cells/kg recipient body weight is required for satisfactory engraftment. Tumor contamination of collected cells represents a possible source of relapse and patients with significant tumor infiltration of marrow may be less desirable autotransplant candidates.46, 47 Various “purging” techniques to reduce or eliminate tumor cell contamination in vitro are being investigated.
Patients undergo collection of bone marrow or peripheral blood progenitor cells that are then cryopreserved in a viable state. Patients subsequently receive high-dose myelosuppressive therapy to eradicate the malignancy, followed by reinfusion of the stored cells to reconstitute hematopoiesis. Autologous hematopoietic cells can potentially be used as vehicles for gene therapy designed to correct deficiency states.
Allogeneic transplantation
Allogeneic transplants are collected from related or unrelated donors. The human leukocyte antigen (HLA) system is the major histocompatibility complex (MHC) in man, and the results of allogeneic transplantation depend on the histocompatibility between donor and recipient. The HLA system is encoded by several closely linked loci on the short arm of chromosome 6.48, 49 Class I loci include HLA-A, -B, and -C alleles. The class II region includes DR, DQ, and DP loci.
Historically, transplants have been from HLA-identical sibling donors. The HLA genes are inherited as a haplotype with one haplotype derived from each parent. There is approximately a one in four chance that any given sibling will be HLA matched with a potential recipient. Registries of potential unrelated donors have been established to provide hematopoietic transplants for patients lacking a histocompatible relative. HLA gene frequencies vary considerably among racial and ethnic groups, and patients are most likely to find transplants among potential donors of their own genetic background. Linkage disequilibrium occurs such that some haplotypes occur commonly, but approximately half of the population have rare haplotypes. There are now over 18 million potential unrelated bone marrow donors accessible in registries worldwide, and approximately half of patients can access an HLA-A, -B, -C, -DR-, and -DQ identical donor. Ten-antigen matched unrelated donor transplants now have outcomes that approximate those for histocompatible sibling donors.50, 51
Transplant recipients who are mismatched at one or more loci are at higher risk of graft rejection, GVHD, and associated complications. One haplotype-identical related donors are readily available for most patients. The use of posttransplant high-dose cyclophosphamide has been shown to moderate GVHD risk and make haploidentical-related transplants increasingly possible.52 In addition, UCB is now frequently used.53, 54 Constraints for HLA matching of UCB are much less than that for other cell sources, with mismatches at 2 of 6 HLA-A, -B, and DR loci being acceptable.55 Owing to the size of the units, UCB transplants have been most successful in children but can also be accomplished in adults when large or multiple cord products are used. Delayed immune reconstitution and a high frequency of opportunistic infections remain major complications of both these techniques.
Like autotransplantation, allogeneic cells are usually administered after an intensive chemotherapy program. In addition to tumor killing, these programs are also designed to produce immunosuppression sufficient to facilitate engraftment of the allo cells. The intensity required is, in part, proportional to the immunocompetence of the recipient. After hematopoietic recovery, the degree of donor engraftment relative to host hematopoiesis (termed chimerism) is most commonly measured by PCR of DNA-restriction fragment length polymorphisms of the donor. In some circumstances, reduced-intensity chemotherapy is used to decrease regimen-related toxicity or to produce a mixed chimeric state. Delayed DLI can be used subsequently to produce full donor chimerism.56 This technique is particularly useful when older patients are treated.57, 58
Nonablative hematopoietic transplant
The recipient, a patient with normal and malignant leukemia cells in the bone marrow, receives a nonmyeloablative preparative regimen designed to prevent rejection of the transplant. The patient receives hematopoietic cells from the donor, which, after engraftment, produces a state of mixed chimerism in which both donor- and recipient-derived cells coexist (Figure 2). A subsequent graft-versus-hematopoietic effect may then occur, which eradicates residual normal and malignant cells of host origin, resulting in complete chimerism (presence of only donor derived hematopoietic and immune cells). A DLI may be administered to augment the GVM effect.
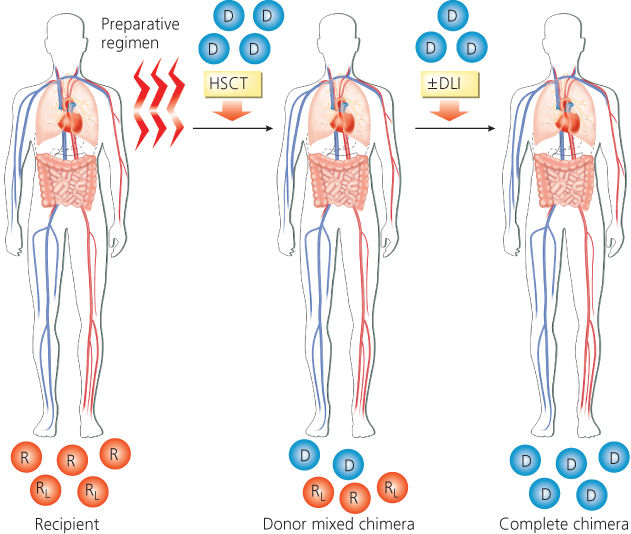
Figure 2 Scheme of nonablative allogeneic hematopoietic transplantation. Abbreviations: D, donor hematopoietic cells; DLI, donor lymphocyte infusion; HSCT, hematopoietic stem cell transplant; R, recipient’s normal bone marrow cells; RL, recipient’s leukemia cells in bone marrow.
Selection of autologous or allogeneic transplantation
Selection of the type of transplantation for a patient, autologous or allogeneic, depends on the type of malignancy, age of the recipient, availability of a suitable donor, ability to collect a tumor-free autograft, stage and status of disease (bone marrow involvement, bulk of disease, chemosensitivity to conventional chemotherapy), and the malignancy’s susceptibility to GVM effects.
Autologous transplantation is readily available, and there is no need to identify an HLA-matched donor. Autologous transplants have a lower risk of life-threatening complications; there are no risk of GVHD and no need for immunosuppressive therapy to prevent GVHD and graft rejection. Immune reconstitution is more rapid than after an allogeneic transplant and there is a lower risk of opportunistic infections. Graft failure occurs rarely. Treatment-related mortality is lower than 5% in most studies, and elderly patients can tolerate treatment relatively well.59, 60 However, autologous transplants have several drawbacks. Clonogenic tumor cells involving blood or bone marrow can contribute to relapse. Autologous transplantation relies solely on the effect of high-dose cytoreductive treatment for tumor eradication and lacks the immune-mediated GVM effect resulting after allogeneic transplantation. In most malignancies, relapse rates are higher after autologous transplants than after allogeneic transplantation, although this is often offset by a lower rate of treatment-related mortality. Patients with extensive prior therapy are at high risk for developing myelodysplasia and secondary acute leukemia after autologous hematopoietic transplantation.61, 62
Allogeneic transplantation has the advantage that the graft is free of contaminating tumor cells and includes donor-derived immunocompetent cells that may produce an immune GVM effect. Allogeneic transplants may be associated with a number of potentially fatal complications such as regimen-related organ toxicity, graft failure, and GVHD. Immune reconstitution is slower after allogeneic transplantation and opportunistic infections are more frequent. Treatment-related mortality is significantly higher than with autologous transplantation.
In general, allogeneic transplants have been used in the treatment of leukemias and myelodysplastic syndromes (MDS). Autologous transplants have been used more often in solid tumors, lymphoma, and myeloma, although nonablative allogeneic transplants are under evaluation in these disorders as a means to induce GVM effects.
The outcome of transplantation relates to the selection of patients and timing of transplant during the natural history of the malignancy. The best results occur when the transplant is performed early in the disease course, when the malignancy is still sensitive to chemoradiotherapy treatment, and when the tumor burden is low. Conversely, transplants done as a last resort are associated with high rates of both relapse and treatment-related toxicity. Responsiveness to conventional-dose chemotherapy is a major predictive factor for the outcome of hematopoietic transplant. The best results have been achieved in patients with chemosensitive relapse or when transplant is performed in high-risk patients as consolidation of response, particularly in patients with minimal disease at the time of transplant. Primary resistance can sometimes be overcome by dose-intense treatment, such as in patients with acute leukemia, Hodgkin’s disease, or MM who have failed to achieve an initial remission. However, patients with bulky disease, refractory relapse, or multiple relapses of their malignancy have a poor prognosis.
Pretransplant therapy
Autologous transplantation
Early autologous treatment regimens were copied from the allogeneic experience and utilized cyclophosphamide plus either TBI or busulfan. TBI has the advantage of being able to be delivered in precise dose and with protection of vulnerable organs such as lungs and kidneys. TBI is suboptimal for the treatment of bulk tumor masses where hypoxia and resistance may characterize deep-seated tumor cells. In contrast, chemotherapy agents such as busulfan penetrate tumor vascularity and reach deep-seated tumors more effectively.
Alkylating agents are the most commonly used drugs in transplant regimens because they usually kill tumor cells in proportion to increasing dose. The most commonly used alkylating agents include cyclophosphamide, melphalan, busulfan, BCNU, and thiotepa. The platinum derivatives, carboplatin63 and cisplatin,64 are also used. Other commonly used nonalkylators are etoposide (VP-16), cytosine arabinoside, and fludarabine. The most frequently used chemotherapy regimen is BCNU, etoposide, Ara-C, and melphalan (BEAM), commonly used for Hodgkin disease and lymphomas.65 The ifosfamide, carboplatin, and etoposide (ICE) regimen is also used for these diseases. In recent years, monoclonal antibodies have proven to be useful when added to standard treatments for leukemia and lymphoma. The anti-CD20 monoclonal antibody rituximab, shown to improve the survival of patients with intermediate grade lymphoma when combined with cyclophosphamide, doxorubicin, vincristine, and prednisone (CHOP) chemotherapy, appears to improve the effectiveness of BEAM.66
Allogeneic transplantation
The preparative regimen generally involves chemotherapy drugs alone or with TBI. The classical “myeloablative” regimens used in the treatment of leukemia are designed to ablate both hematopoiesis and immunity in the recipient and typically involve the combination of high-dose cyclophosphamide with either TBI67, 68 or high-dose busulfan.69, 70
The toxicity of high-dose myeloablative therapy limits the use of this modality to relatively young patients in good medical condition. The discovery of the curative potential of the immune-mediated GVM effect has led to a novel approach using lower dose, reduced intensity preparative regimens as a means to reduce the toxicity of allogeneic hematopoietic transplantation. These reduced intensity conditioning regimens have been designed not to fully eradicate the malignancy, but rather to provide sufficient immunosuppression to achieve engraftment and to allow induction of GVM (Figure 2).71, 72 These regimens can be tolerated by older patients and those with comorbidities who are not eligible to receive an ablative preparative regimen. Most reduced-intensity regimens include a purine analog, such as fludarabine, combined with an alkylating agent (cyclophosphamide, melphalan, or busulfan), or low-dose TBI. Engraftment has been achieved in most patients, and this approach is effective against a range of hematologic malignancies. Antithymocyte globulin is often added to produce additional immune suppression.
Complications of hematopoietic transplantation
Intensive chemoradiotherapy and hematopoietic transplantations may be associated with a number of serious complications as listed in Table 2. These include immune-mediated processes such as graft rejection and GVHD, toxicities resulting from the pretransplant conditioning regimen, infections owing to neutropenia, and posttransplant immune deficiency.
Table 2 Complications after hematopoietic transplantation
Immune complications
|
Regimen-related toxicity of the preparative regimen
|
Hematologic complications
|
Infections and immunodeficiency
|
Late complications
|
Abbreviation: EBV, Epstein–Barr virus.
Graft rejection and graft failure
Graft failure is defined as the failure to establish hematopoietic engraftment (primary graft failure) or loss of an established graft (secondary graft failure). Graft failure after autologous transplant occurs rarely and is most often related to infusion of an inadequate number of viable stem cells.
Graft failure after allogeneic transplantation is also caused by immunologic graft rejection.73, 74 With current regimens, rejection occurs in fewer than 2% of transplants from an HLA-identical sibling. The risk is increased in recipients of HLA-mismatched grafts.75, 76 Donor CD8+ T lymphocytes provide a graft-facilitating effect, and T lymphocyte depletion from the allogeneic graft increases the risk of graft failure.77, 78 Cord blood transplants containing a lower number of CD34+ and CD8+ cells are more prone to engraftment failure.
Secondary graft failure or poor graft function may be caused by administration of myelosuppressive drugs, GVHD, and infections in the early posttransplant period. Ganciclovir or trimethoprim-sulfamethoxazole can produce pancytopenia, which is usually reversible after discontinuation of drug. Cytomegalovirus (CMV),79 parvovirus,80 human herpesvirus,81, 82 and mycobacterial and fungal infections may also compromise the graft. Poor engraftment may also result from microenvironment or marrow stroma dysfunction related to the patient’s underlying disease or prior therapy.
Graft failure that is not due to rejection can often be successfully treated with G-CSF or second hematopoietic stem cell infusion from the same donor or an alternative donor.
Graft-versus-host disease
GVHD is a major, potentially life-threatening complication of allogeneic hematopoietic transplantation. Acute and chronic GVHD are distinct but interrelated syndromes. Acute GVHD typically occurs within the first 100 days posttransplant and results from reactivity of mature donor T lymphocytes present in the graft directed against disparate major or minor histocompatibility antigens of the recipient (the host).83 Acute GVHD involves the skin, gastrointestinal (GI) tract, and liver as the primary target tissues. The hematopoietic and immune systems are also involved. A maculopapular rash is usually the first presentation and is typically pruritic and confluent. When severe, generalized erythroderma, bullae, and desquamation may occur. Acute GVHD of the liver targets the biliary epithelium and produces cholestatic hepatitis with marked elevation of bilirubin and alkaline phosphatase. GVHD can affect the entire GI tract, targeting epithelial cells. GI GVHD characteristically produces secretory diarrhea, abdominal pain, and on rare occasions, ileus. Upper GI GVHD produces nausea, vomiting, and anorexia, all which may occur without lower GI tract or other tissue involvement.84 Conjunctivitis and other ocular manifestations, anemia, and thrombocytopenia often occur.
Severe GVHD is associated with a poor prognosis because of direct tissue damage, debilitation, and severe immunodeficiency caused by the GVHD process itself and by its treatment with immunosuppressive drugs.
The most important factor predicting the risk of GVHD is HLA disparity between the donor and recipient.85–88 With current immunosuppressive prophylaxis, acute GVHD occurs in 25–50% of patients after transplants from an HLA identical sibling; this may be related to disparity between minor histocompatibility antigens. A higher incidence up to 61–90% has been reported following transplants from mismatched and unrelated donors. Results are improving for unrelated donor transplantation with the use of more precise molecular histocompatibility typing to identify donors. Older age is associated with an increased incidence of acute and chronic GVHD. Less intensive, nonmyeloablative conditioning regimens may also limit the severity of GVHD, presumably owing to reduction in tissue damage limiting the proinflammatory phase that facilitates development of GVHD.
Pharmacologic immunosuppression is generally administered for the first 6 months posttransplant to reduce the incidence and severity of GVHD. The current standard of care combines either cyclosporine or tacrolimus with a short course of methotrexate.89, 90 Corticosteroids are the first line of therapy in patients who develop acute GVHD. Approximately half of patients have a sustained response,91 and the steroid dose can be gradually tapered off. Steroid-resistant GVHD has an unfavorable prognosis. The prognosis is best for GVHD limited to the skin. Acute GVHD involving the liver or multiple organs has a poorer prognosis than other sites. A number of other immunosuppressive agents are being studied. For mismatched grafts, recent studies have demonstrated that the use of post-transplant cyclophosphamide can decrease the risk of GVHD and permit related haploidentical transplants to be performed with acceptable risk.92
Chronic GVHD is a related syndrome affecting 25–60% of recipients of allogeneic transplantation who survive more than 6 months after transplant.93 It most often occurs between 80 and 200 days after transplant, but onset may be delayed to the second year. Chronic GVHD is more common in older patients and in patients with prior acute GVHD although approximately one-third of affected patients have a de novo presentation without prior acute GVHD.94 Chronic GVHD is more prevalent after transplants with peripheral blood stem cells than with marrow transplantation.95
Chronic GVHD has multiple clinical manifestations similar to those seen in several autoimmune disorders such as progressive systemic sclerosis, Sjögren syndrome, and primary biliary cirrhosis. Chronic GVHD is associated with profound immunosuppression and a major risk to the patients of opportunistic infections. Bronchiolitis obliterans without other major manifestations of chronic GVHD and intestinal involvement with anorexia, dysphagia, malabsorption, and wasting may occur. Polymyositis, serositis, and autoimmune manifestations occur rarely. Chronic GVHD may become a chronic debilitating disease affecting quality of life and remains the major determinant of late transplant-related morbidity. Progressive onset of extensive chronic GVHD from acute GVHD and the presence of thrombocytopenia are poor prognostic factors (Table 3).96
Table 3 Clinical staging and grading of graft-versus-host disease (GVHD)
Acute GVHD | ||||||
Stage | Skina | Liverb | Gutb | |||
1 | Maculopapular rash <25% | Bilirubin 2–3 mg/dL | Diarrhea 500–1000 mL or persistent nauseac | |||
2 | Maculopapular rash 25–50% | Bilirubin 3–6 mg/dL | Diarrhea 1000–1500 mL | |||
3 | Generalized erythroderma | Bilirubin 6–15 mg/dL | Diarrhea >1500 mL | |||
4 | Desquamation and bulla | Bilirubin >15 mg/dL | Severe abdominal pain or ileus | |||
Overall graded | Severity | Skin | Liver | Gut | ||
0 | None | 0 | — | 0 | — | 0 |
I | Mild | 1–2 | — | 0 | — | 0 |
II | Moderate | 3 | or | 1 or | or | 1 |
III | Severe | — | — | 2–3 or | — | 2–3 |
IVe | Life threatening | 4 | or | 4 or | or | —4 |
Chronic GVHD | ||||||
Limited | Localized skin involvement and/or hepatic dysfunction | |||||
Extensive | ||||||
|
a Extents determined by rule of nines or burn chart.
b Downgrade one stage for additional causes of elevated bilirubin or diarrhea.
c Requires histologic evidence of GVHD in the stomach or duodenum.
d
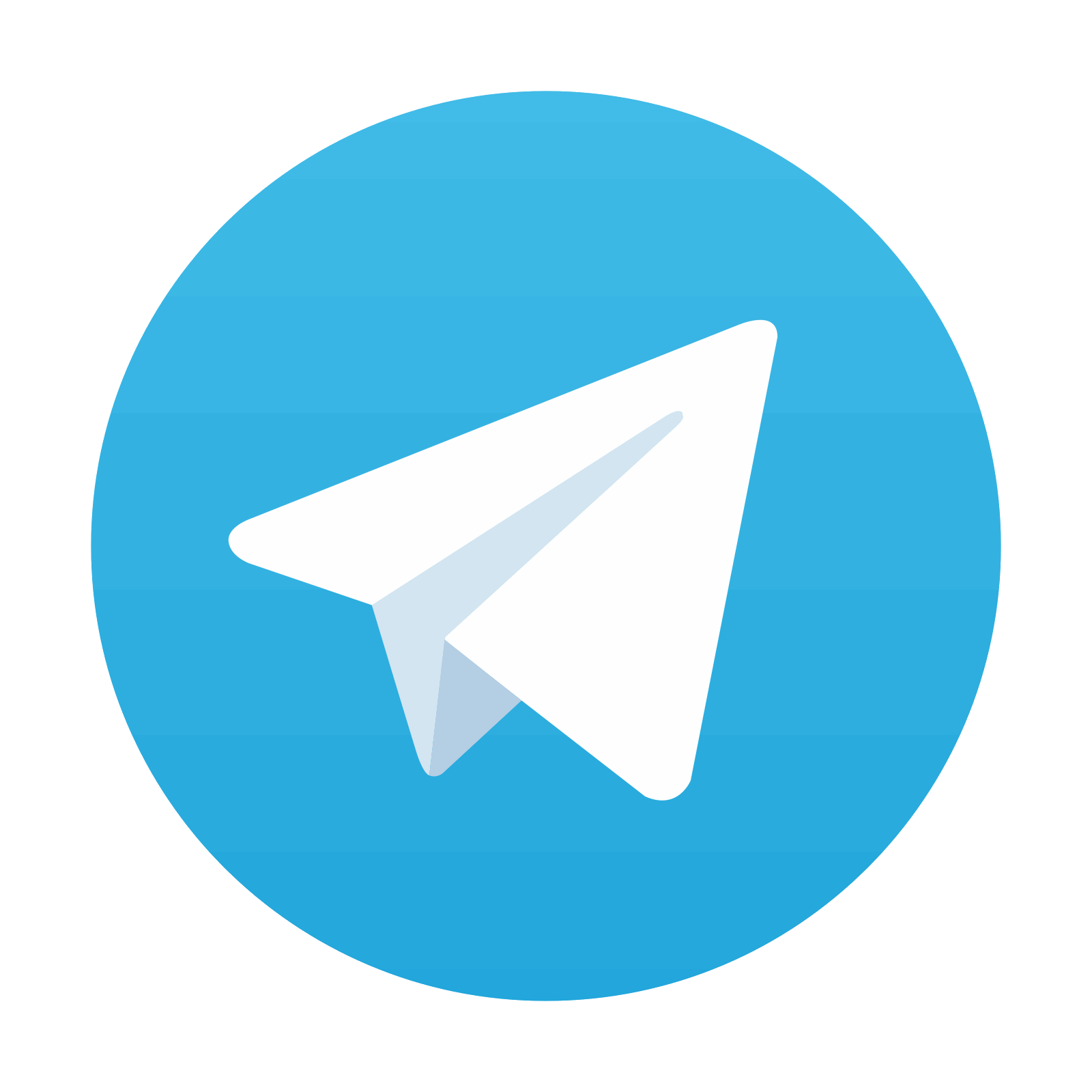
Stay updated, free articles. Join our Telegram channel

Full access? Get Clinical Tree
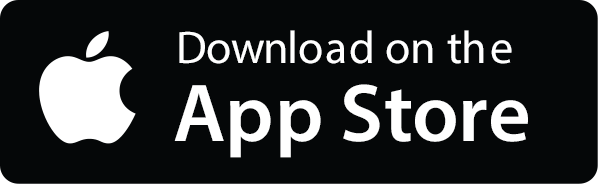
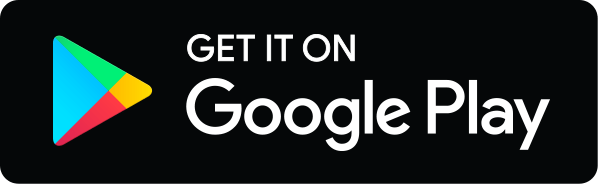