Hematologic complications and blood bank support
Richard M. Kaufman, MD Kenneth C. Anderson, MD
Overview
Many of the fundamental therapies used to treat cancer (chemotherapy and stem cell transplantation) disrupt normal hematopoiesis in the bone marrow. As a result, blood transfusion for patients with cancer is an essential supportive modality. The primary focus of this chapter is on “classical” blood component support: how red cells, plasma, platelets, and granulocytes are collected, tested, stored, and administered to address specific deficiencies in patients with cancer. Particular attention is paid to studies of prophylactic platelet transfusion, which over the past several decades has been critical in allowing myelosuppressive treatments to be applied to a variety of malignant disease states. Current infectious risks of blood products are reviewed.
Hematologic complications occur commonly in patients with cancer, related either to the underlying disease or to its treatment.1, 2 Abnormalities in the red blood cell (RBC), leukocyte (WBC), and platelet number and function require transfusion medicine expertise for the provision of appropriate blood component support.3, 4 Indeed, the therapeutic advances made using high-dose combination chemotherapeutic approaches to date would not have been possible without the parallel development of technology to support patients through the hematologic complications of therapy.
Causes of pancytopenia
Cancer and its treatment may alter normal hematopoiesis either by direct effects on hematopoietic stem cells or by inhibiting production of and responsiveness to hematopoietic growth factors (Table 1).
Table 1 Causes of anemia, thrombocytopenia, and leukopenia in cancer
Bone marrow replacement by primary tumor (e.g., leukemia) |
Bone marrow involvement by metastatic tumor (e.g., breast and prostate) |
Derangement of normal physiology
|
Myelosuppression by chemotherapy or radiotherapy |
Disease related
Bone marrow hematopoietic cells can be replaced either by primary tumor derived from marrow cells, or by metastatic spread of tumor to the marrow from neoplasms of other organs. Hodgkin and non-Hodgkin lymphoma (NHL), malignant melanoma, neuroblastoma, as well as carcinoma of the breast, prostate, lung, adrenal, thyroid, and kidney commonly manifest marrow involvement. Ultimately, diffuse involvement of marrow with tumor can lead to either marrow fibrosis or necrosis, which may be associated with splenomegaly, thrombocytopenia, and immature cells of all lineages in the peripheral blood.5
Chemotherapy related
The role of treatment in marrow injury and recovery varies both with the drugs employed and with the normal turnover rate of cells of different hematologic lineages. The characteristic effects of several drugs on marrow are shown in Table 2. The bone marrow has a storage compartment that can supply mature cells to the peripheral blood for 8–10 days after the stem-cell pool has ceased to function. Events in the peripheral blood are therefore a week behind the events in the bone marrow. In previously untreated patients, leukopenia and thrombocytopenia are described on the ninth or tenth day after treatment, with the nadir of counts on days 14–18. Recovery of counts is evident by day 21 and complete by day 28. The cytotoxic dose–response effect is usually related to the nadir WBC and platelet count, not the duration of cytopenia. This is due to the resting state of the stem cells of normal bone marrow, which protects them from damage.
Table 2 Characteristic effects of drugs and treatments on bone marrow
Treatment | Hematologic complication |
Most chemotherapeutic agents | Leukopenia and thrombocytopenia at 9–10 days; nadir counts at 14–18 days; recovery of counts at 21–28 days |
Nitrosourea | Myelosuppression at 4–6 weeks |
Vincristine, 1-asparaginase, bleomycin, myelosuppression methotrexate with leucovorin | No myelosuppression |
Gamma irradiation | Chronic lymphopenia |
Whole-body irradiation | Profound suppression of humoral and cellular immune response |
Gamma radiation related
Cells damaged by irradiation may divide one time or more before all progeny are rendered reproductively sterile; thus, an irradiated cell will not appear damaged until it divides.6 At the time of the first postirradiation subdivision, the cell may die, divide aberrantly and produce unusual forms, be unable to divide and remain physiologically functional, or give rise to one or more generation of progeny until cells become sterile. As bone marrow stem cells have a very low capacity for repair of sublethal irradiation damage, multiple smaller radiation fractions may preserve other normal tissues (e.g., lung and intestine), but will not spare bone marrow.
Associated processes
It is essential to remain cognizant of derangements in normal physiology that may occur in the setting of cancer and/or treatment and that contribute to pancytopenia. These include nutritional factors, such as folate, iron, or vitamin deficiencies. There may be abnormal feedback loops in hematopoiesis, such as cell-mediated suppression of hematopoiesis in aplastic anemia or stimulation of thrombopoiesis in the setting of antibody-mediated platelet destruction.7 Fibrosis can occur either as part of a disease process or as a reaction to therapy, thereby compromising bone marrow reserve. Immunologically mediated destruction of cells and other factors, such as splenomegaly, can result in cytopenias. Moreover, occult bleeding must always be considered as a cause of persistent anemia and refractory thrombocytopenia. These clinical examples emphasize the importance of carefully assessing patients with cancer for treatable medical etiologies of their hematologic complications before attributing these effects to their underlying neoplasms.
Abnormalities of red cells and red cell support
Anemia
Anemia in patients with cancer can be mild to severe and may be attributable to many causes. Hematopoiesis in patients with early stages of cancer may be normal. On the other hand, replacement of marrow cells by tumor is not essential for the development of anemia, even in patients with metastatic cancer. Most commonly, the incidence and magnitude of anemia in patients with cancer increase as the disease progresses. This anemia is designated as anemia of chronic disease only if the cellular pattern in the marrow is nearly normal, the serum iron and iron-binding capacity are low, the iron content of the marrow is normal or increased, and the serum ferritin is elevated.8 The coexistence of low plasma iron levels with adequate amounts of storage iron helps distinguish anemia of chronic disease from iron-deficiency anemia. Moreover, other causes of anemia, for example, overt hemolysis, bleeding, nutritional deficiency, or marrow replacement, must be ruled out. In some patients, such as those with Hodgkin disease (HD), erythrophagocytosis or hypersplenism may account for this decrease in red-cell survival, but in others its etiology is unclear.
Red-cell transfusion
Red-cell transfusions are indicated to increase oxygen-carrying capacity in patients with anemia that is not adequately compensated by normal physiologic mechanisms. Sufficient oxygen-carrying capacity to maintain cardiopulmonary function can be met by a hemoglobin of 7 g/dL (a hematocrit of approximately 21%) when the intravascular volume is adequate for perfusion.9 In a multicenter randomized trial of critical care patients, a restrictive transfusion strategy (maintaining hemoglobin levels between 7 and 9 g/dL) was demonstrated to be at least as safe as a more liberal transfusion strategy, with the possible exception of patients with acute myocardial infarction or unstable angina.10 Subsequent randomized trials have compared restrictive versus liberal RBC transfusion strategies in patients with cardiovascular disease having hip surgery11 or cardiac surgery,12–14 in patients with sepsis,15 and in patients with upper gastrointestinal bleeding.16 A restrictive transfusion strategy (hemoglobin transfusion threshold of 7–8 g/dL) appears to be safe and appropriate for the vast majority of hospitalized inpatients. Currently, however, high-quality clinical trial data to guide RBC transfusion in patients with malignancy are still awaited. Overall, there is no single hemoglobin level that can be universally applied as a “transfusion trigger.” In deciding whether to transfuse a specific patient, the physician should consider the patient’s age, degree of anemia, the intravascular volume, and the presence of coexisting cardiac, pulmonary, or vascular conditions.17 To meet oxygen needs, some patients may require RBC transfusions at higher hemoglobin levels. In particular, hemoglobin levels are commonly maintained at levels of 8 g/dL in the setting of cancer and its therapy. Transfusing one unit of RBC will usually increase the hemoglobin by 1 g/dL and the hematocrit by 2–3% in the average adult weighing 70 kg.
Packed red blood cells (PRBC) are prepared either from whole blood (WB) by the removal of plasma or by erythrocytapheresis. Red cells can be depleted of leukocytes by filtration to produce leukoreduced red cells (LRBC). Leukoreduction can prevent a significant percentage of febrile nonhemolytic transfusion reactions and cytomegalovirus (CMV) infection in transfusion recipients.18 In the United States and internationally, there is a trend to universally transfuse leukoreduced cellular components to avoid the multiple adverse sequelae of leukocytes. Washed RBCs are prepared by further removal of plasma from PRBCs. These products are sometimes indicated to prevent allergic reactions to plasma proteins.19 PRBCs are currently stored for up to 42 days at 4°C. Retrospective studies have suggested that transfusing older stored PRBC units may be associated with adverse consequences for the recipient.20 However, older RBC units have not been demonstrated to increase morbidity or mortality when compared with fresher RBC units in multiple randomized controlled trials.21–23
Leukopenia and white cell support
Leukopenia
Leukopenia may occur related to cancer and its treatment. In 1965, Hersh and colleagues summarized the causes of death in patients with acute leukemia treated at the National Cancer Institute and noted a marked decline in fatal hemorrhage, owing to the availability of platelet transfusions, with a concomitant increase in the occurrence of infection alone as a cause of death.24 A quantitative relationship between circulating leukocytes and infection was established in patients with leukemia; in particular, the probability of being infected is proportional to both the severity and duration of leukopenia.25
Granulocyte transfusion
Therapeutic granulocytes were first utilized nearly 30 years ago in leukemic patients with leukopenia and serious infection. The earliest trials, which demonstrated the potential value of granulocyte transfusions, utilized granulocytes harvested from patients with chronic myelogenous leukemia and achieved cell dosages never approached when normal donors were utilized.26 The importance of dose was defined: less than 1010 granulocytes were ineffective whereas greater than 1011 cells were effective. Indeed, in an afebrile, uninfected man, the half-life of granulocytes in the circulation is 6.7(4–10) h and the daily turnover rate is 230%. Parallel work in canine models had also demonstrated that dogs deliberately made leukopenic by irradiation and given Gram-negative bacteremia and pneumonia could be successfully treated with granulocyte transfusions.27
There have beensix randomized prospective trials of prophylactic leukocyte transfusions, given to prevent infections in leukopenic recipients.28, 29 However, none of the studies demonstrated improved survival because alloimmunization, transfusion reactions, CMV infection, and pulmonary infiltrates occurred more frequently in the transfused group. These studies have been criticized owing to inadequate donor-recipient matching and inadequate doses of granulocytes transfused. A large randomized trial of high-dose granulocytes collected from donors mobilized with a combination of G-CSF and dexamethasone has been completed.29 Currently, the role for granulocyte transfusions remains controversial. When granulocytes transfusions are used, the products must irradiated to prevent graft-versus-host disease (GVHD) when transfused to an immunocompromised host.
Thrombocytopenia and platelet support
Thrombocytopenia
Thrombocytopenia in cancer patients is usually attributable to treatment with chemotherapy and radiotherapy. Impaired production of platelets due to a decrease or absence of megakaryocytes is therefore the most common cause of thrombocytopenia in patients with cancer (Table 3). However, thrombocytopenia may also be due to splenic sequestration in patients who have splenomegaly as part of their primary neoplastic process. In this setting, increased numbers of megakaryocytes are evident unless extensive marrow infiltration is present. Immune-mediated thrombocytopenia may also occur related to antihuman leukocyte antigen (anti-HLA) or antiplatelet-specific alloantibodies. Finally, thrombocytopenia may be related to diffuse intravascular coagulation (DIC), especially in patients with acute myelocytic leukemias, lymphomas, and carcinoma of lung, breast, gastrointestinal, or urologic origin. DIC commonly complicates acute promyelocytic leukemia owing to the presence of both thromboplastic material and fibrinolytic proteases in the promyelocytic subcellular components.30
Table 3 Causes of thrombocytopenia
Acute thrombocytopenia due to increased
|
Immune destruction of platelets
|
Hereditary defects |
Thrombocytopenia with decreased platelet production
|
Idiopathic megakaryocytic aplasia |
Marrow infiltration
|
Following radiation or myelosuppressive drugs |
Drugs producing specific suppression of platelet production (e.g., thiazides, ethanol, and estrogens) |
Nutritional deficiency—megaloblastic anemia and severe iron deficiency (rare) |
Viral infections |
Paroxysmal nocturnal hemoglobinuria |
Abnormalities in platelet function
Platelet function can be abnormal in several chronic myeloproliferative disorders. Although most bleeding in patients with acute myeloid leukemia (AML) is related to thrombocytopenia, intrinsic abnormalities in platelet function have been described including decreased platelet procoagulant activity and decreased aggregation and serotonin release responses to ADP, epinephrine, or collagen.31 Platelet dysfunction is evident in a fraction of patients with IgA myeloma or Waldenstrom macroglobulinemia, multiple myeloma, and monoclonal gammopathy of undetermined significance.32
Platelet transfusion support
In 1910, fresh WB was first transfused to thrombocytopenic patients, resulting in a significant rise in the platelet count, hemostasis, and improvement of the bleeding time.33 In the 1950s, platelets were first used for the treatment of thrombocytopenia related to combination chemotherapeutic treatments of leukemias.34 Data from the National Cancer Institute in the early 1960s clearly demonstrated that leukemia patients died of hemorrhage during induction of remission with chemotherapy and established the quantitative relationship between platelet count and hemorrhage.35 It was shown that platelet therapy could modify the course of hemorrhage in both pediatric and adult settings, the only difference being the doses required. Two recent randomized trials compared a prophylactic platelet-transfusion strategy with a therapeutic platelet-transfusion strategy in patients with hypoproliferative thrombocytopenia. Both studies demonstrated a benefit, albeit limited, of platelet prophylaxis, and both showed that prophylaxis has a greater effect on bleeding risk in patients treated with chemotherapy for leukemia compared with autologous stem cell transplant recipients.36
Single- and multiple-donor platelets
In the United States, one unit of platelet concentrate is obtained from one unit of WB by centrifugation, and contains approximately 5.5 × 1010 platelets/unit. (This method of preparing platelets is known as the “platelet-rich plasma” (PRP) method. In other countries, platelet concentrates are prepared from WB using the alternate “buffy coat” method.) Concentrates from multiple (4–6) donors are pooled to produce a single component for transfusion. Apheresis technology has permitted harvesting the equivalent of several platelet concentrates from a single donor during a single donation. A single-donor platelet unit typically contains at least 3 × 1011 platelets/unit. The use of single-donor platelets is increasing; they are generally considered to be the platelet product of choice for patients being treated for malignancy.37 Single-donor platelet collections are utilized to provide HLA-matched donors for alloimmunized recipients who have not responded to platelets from random donors. In the United States, platelet units may be stored at room temperature for up to 5 days.
Indications for therapeutic and prophylactic platelet transfusion
Platelets are commonly transfused to patients with cancer. The majority of platelet transfusions are given prophylactically to prevent bleeding, as opposed to therapeutically, to treat active bleeding.38 The appropriate indications for transfusion of platelets have been the subject of a recent clinical practice guideline from the AABB.39 At present, one dose of one apheresis platelets (or equivalent pool of random donor-platelet concentrates) is routinely transfused to cancer patients with platelet counts less than 10,000/mm3 to reduce the risk of hemorrhage. Data from Gmur and colleagues suggested that the threshold for prophylactic platelet transfusion can safely be set at 5 × 109/L in patients with acute leukemia without fever or bleeding manifestations and at 10 × 109/L in patients without such signs.40 Prospective clinical trials have shown that the risk of major bleeding was similar whether 10 × 109/L or 20 × 109/L was used as the platelet-transfusion threshold in patients with acute leukemia, and that the lower threshold reduced platelet use.41–43
The risk of bleeding at a given platelet count may vary in distinct clinical settings. For example, patients with thrombocytopenia due to AML were reported to have increased bleeding at less than 10,000/mm3 platelets, in contrast to patients with acute lymphocytic leukemia (ALL), who had similar risk of hemorrhage at less than 20,000/mm3 platelets.44 Patients with chronic thrombocytopenia due to decreased platelet production (i.e., myelodysplastic disorders) may require transfusions, in contrast to patients with accelerated destruction but active production of platelets (i.e., idiopathic thrombocytopenic purpura), who may not require routine platelet transfusions. Moreover, patients with chronic thrombocytopenia may tolerate lower absolute platelet counts without transfusion. In patients with abnormalities of platelet function, it is not the absolute platelet count but rather the number of functional platelets that is important for the prevention of bleeding. Thus, it is difficult to define an absolute platelet threshold for transfusion for all patients, and both the timing and the dose of prophylactic platelet transfusion must therefore be determined on a clinical basis.39, 45–47
The multicenter randomized PLADO (PLAtelet DOsing) study demonstrated that prophylactic transfusion with low-dose platelets does not increase the risk of Grade 2 or higher bleeding as compared with standard-dose prophylaxis among inpatients with hypoproliferative thrombocytopenia. Patients receiving low-dose prophylaxis do require more frequent platelet transfusions, although fewer total platelets are required overall.48
Clinical and laboratory assessment of the effectiveness of platelet transfusion
The effectiveness of platelet transfusion can be assessed by laboratory parameters (the platelet count increment 1 h or 10–15 min after transfusion) and by the observed clinical outcome after transfusion.49–52 The corrected count increment is defined as the increment in platelet counts from pre- to post-transfusion corrected for the number of units transfused and for the body surface area of the recipient. A CCI of 15,000–20,000/mm3
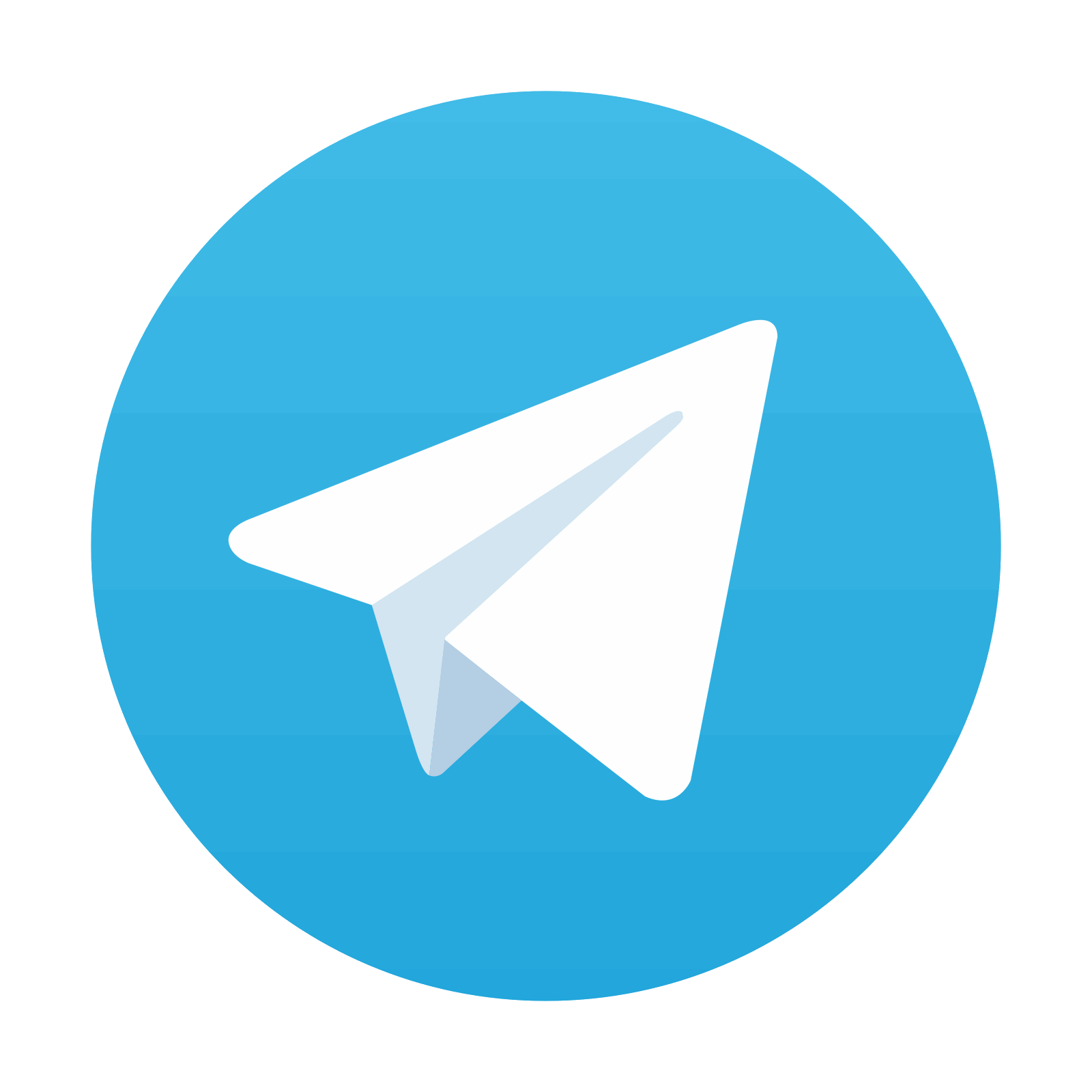
Stay updated, free articles. Join our Telegram channel

Full access? Get Clinical Tree
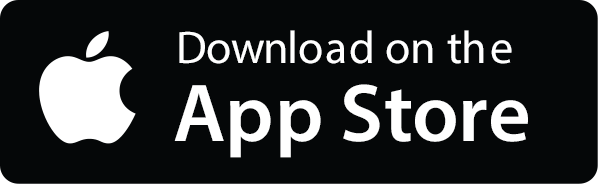
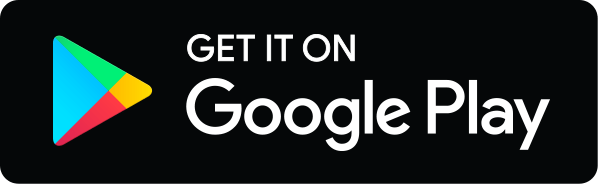