Considerable progress has been made in recent years in understanding of the genetic basis for congenital neutropenia syndromes. With the advent of high-throughput genomic analyzing technologies, the underlying genetic causes of other congenital neutropenia syndromes are expected to be resolved in the near future. This knowledge will provide the foundation for genotype-phenotype correlations for infection susceptibility, response to therapy, and risk of malignant transformation, enabling optimal care for individual patients depending on their molecular pathophysiology. It is hoped that these investigations will enable the development of tailored molecular therapies to specifically correct the aberrant signaling cascades.
Key points
- •
Considerable progress has been made in recent years in understanding of the genetic basis for congenital neutropenia syndromes.
- •
With the advent of high-throughput genomic analyzing technologies, the underlying genetic causes of other congenital neutropenia syndromes are expected to be resolved in the near future.
- •
This knowledge will provide the foundation for genotype-phenotype correlations for infection susceptibility, response to therapy, and risk of malignant transformation, enabling optimal care for individual patients depending on their molecular pathophysiology.
- •
It is hoped that these investigations will enable the development of tailored molecular therapies to specifically correct the aberrant signaling cascades.
Introduction
Congenital neutropenia represents a heterogeneous group of inherited disorders with the common denominator of persistent and genetically determined paucity of neutrophil granulocytes in the peripheral blood. Neutropenia is termed mild when absolute neutrophil counts (ANCs) range from 1.0 to 1.5 × 10 9 /L, moderate with ANCs from 0.5 to 1.0 × 10 9 /L, and severe when ANCs are less than 0.5 × 10 9 /L.
Congenital neutropenia has to be distinguished from autoimmune neutropenias (usually self-limiting and not causing severe bacterial infections) and benign ethnic neutropenia (prevalent in the Middle East and Africa, not predisposing to infections). By contrast, congenital neutropenia leads to recurrent and severe bacterial (and sometimes fungal) infections. The classic phenotype of autosomal recessive severe congenital neutropenia (SCN) was described by the Swedish pediatrician Rolf Kostmann almost 60 years ago.
In the past, the genetic basis for several mendelian diseases with congenital neutropenia were elucidated and improved understanding of the molecular processes underlying this group of disorders ( Table 1 ). In the United States and Europe, most patients with SCN have either sporadic or autosomal dominance inheritance patterns and bear monoallelic mutations in the neutrophil elastase ( ELANE ) gene (see the article by Horwitz and colleagues elsewhere in this issue for further exploration of this topic). The molecular basis for autosomal recessive SCN is heterogeneous. The classic variant, originally described by Rolf Kostmann, is caused by mutations in the gene encoding for the mitochondrial protein HS1-associated protein (HAX1). In Europe, mutations in HAX1 seem to be more common than in the United States and account for up to 15% to 20% of cases of SCN (European Severe Chronic Neutropenia International Registry, unpublished data). Biallelic mutations in the glucose-6-phosphatase catalytic subunit 3 ( G6PC3 ) gene cause a complex disorder associating congenital neutropenia and various developmental aberrations such as congenital heart defects, urogenital malformations, and facial dysmorphy. Mutations in the GFI1 gene or activating mutations in the Wiskott-Aldrich syndrome ( WAS ) gene represent rare molecular causes of SCN. The relative frequency of these distinct gene defects among patients with SCN differs depending on ethnicity. In particular, in populations with a high degree of consanguinity, autosomal recessive variants of SCN are more prevalent than mutations in ELANE . Despite recent progress in the field, in around one-third of patients with SCN, no pathogenic mutations can be identified.
Disease | Gene Mutated | Inheritance | Hematopoietic Manifestations | Extrahematopoietic Manifestations | Pathophysiologic Mechanism | References |
---|---|---|---|---|---|---|
(1) SCN | ||||||
ELANE deficiency (SCN1) | ELANE | AD | CN or CyN | — | Activation of the UPR, excessive apoptosis of myeloid cells | |
GFI1 deficiency (SCN2) | GFI1 | AD | CN, lymphopenia | — | Defective myeloid cell differentiation | |
HAX1 deficiency (SCN3) | HAX1 | AR | CN | Epilepsy, neurologic impairment in some patients | Destabilization of mitochondrial membrane potential, excessive apoptosis of myeloid cells | |
G6PC3 deficiency (SCN4) | G6PC3 | AR | CN, thrombocytopenia | Congenital heart defects, facial dysmorphy, increased visibility of superficial veins, urogenital malformations, failure to thrive, endocrine abnormalities, inner ear hearing loss, hyperelasticity of the skin | Impaired intracellular glucose homeostasis, activation of the UPR, excessive apoptosis of myeloid cells | |
XLN | WAS | XL | CN, lymphopenia, myelodysplasia | — | Enhanced and discoordinated actin polymerization, defective cytokinesis | |
(2) Congenital neutropenia: hypopigmentation disorders | ||||||
Chédiak-Higashi syndrome | LYST / CHS1 | AR | CN, defective NK cell function, lysosomal inclusion bodies in leukocytes, macrophage activation syndrome | Oculocutaneous albinism, neurodegeneration | — | |
Hermansky-Pudlak syndrome, type 2 | AP3B1 | AR | CN, impaired function of T and NK cells | Oculocutaneous albinism | Defective endosomal function | |
Griscelli syndrome, type 2 | RAB27A | AR | CN, defective cytotoxicity, macrophage activation syndrome | Oculocutaneous albinism | — | |
P14/ROBLD3/MAPBPIP deficiency | P14 / ROBLD3 / MAPBPIP | AR | CN, defective cytotoxicity, growth failure, lymphoid immunodeficiency | Oculocutaneous albinism | Aberrant distribution of late endosomes | |
(3) Complex disorders comprising congenital neutropenia | ||||||
AK2 deficiency | AK2 | AR | CN, severe lymphopenia (reticular dysgenesis) | Inner ear hearing loss | Aberrant mitochondrial metabolism caused by lack of adenylate kinase 2 | |
Shwachman-Diamond syndrome | SBDS | AR | CN | Exocrine pancreatic insufficiency, skeletal dysplasia, hepatic and cardiac disease | Mitotic spindle destabilization, genomic instability, enhanced apoptosis | |
WHIM syndrome | CXCR4 | AD | CN, myelokathexis, B cell deficiency | Warts, hypogammaglobulinemia, immunodeficiency | Constitutive activating mutations in CXCR4 associated with myelokathexis and lack of neutrophils in peripheral blood | |
Poikiloderma with neutropenia | C16ORF57 | AR | CN, bone marrow abnormalities | Poikiloderma, increased photosensitivity | Disturbed biogenesis of U6 small nuclear RNA, impaired cell viability | |
Cartilage-hair hypoplasia | RMRP | AR | CN, immunodeficiency | Hypoplastic hair, skeletal dysplasia | Defective assembly of ribosomes, aberrant cell cycle control and telomere function | |
CD40L deficiency (HIGM1) | CD40L / CD154 | XL | Intermittent CN, combined immunodeficiency including T and B cell deficiency, defective B cell class switch (hyper-IgM syndrome type I) | — | Defective class switch caused by impaired CD40-CD40L interaction, defective T cell priming | |
Barth syndrome | G4.5 / TAZ | XL | CN (in most patients) | Cardioskeletal myopathy, growth impairment, carnitine deficiency | Mitochondrial dysfunction, excessive apoptosis in myeloid cells | |
Cohen syndrome | VPS13B / COH | AR | Intermittent CN | Psychomotor retardation, skeletal dysplasia, hypotonia | Suspected function of COH in vesicular transport processes | |
Pearson syndrome | Deletion of mitochondrial DNA | mtDNA | CN, bone marrow failure | Exocrine pancreas insufficiency, endocrine abnormalities, neuromuscular degeneration | — |
This article discusses some of the most recent findings and discusses their implications for molecular understanding of neutrophil biology.
Introduction
Congenital neutropenia represents a heterogeneous group of inherited disorders with the common denominator of persistent and genetically determined paucity of neutrophil granulocytes in the peripheral blood. Neutropenia is termed mild when absolute neutrophil counts (ANCs) range from 1.0 to 1.5 × 10 9 /L, moderate with ANCs from 0.5 to 1.0 × 10 9 /L, and severe when ANCs are less than 0.5 × 10 9 /L.
Congenital neutropenia has to be distinguished from autoimmune neutropenias (usually self-limiting and not causing severe bacterial infections) and benign ethnic neutropenia (prevalent in the Middle East and Africa, not predisposing to infections). By contrast, congenital neutropenia leads to recurrent and severe bacterial (and sometimes fungal) infections. The classic phenotype of autosomal recessive severe congenital neutropenia (SCN) was described by the Swedish pediatrician Rolf Kostmann almost 60 years ago.
In the past, the genetic basis for several mendelian diseases with congenital neutropenia were elucidated and improved understanding of the molecular processes underlying this group of disorders ( Table 1 ). In the United States and Europe, most patients with SCN have either sporadic or autosomal dominance inheritance patterns and bear monoallelic mutations in the neutrophil elastase ( ELANE ) gene (see the article by Horwitz and colleagues elsewhere in this issue for further exploration of this topic). The molecular basis for autosomal recessive SCN is heterogeneous. The classic variant, originally described by Rolf Kostmann, is caused by mutations in the gene encoding for the mitochondrial protein HS1-associated protein (HAX1). In Europe, mutations in HAX1 seem to be more common than in the United States and account for up to 15% to 20% of cases of SCN (European Severe Chronic Neutropenia International Registry, unpublished data). Biallelic mutations in the glucose-6-phosphatase catalytic subunit 3 ( G6PC3 ) gene cause a complex disorder associating congenital neutropenia and various developmental aberrations such as congenital heart defects, urogenital malformations, and facial dysmorphy. Mutations in the GFI1 gene or activating mutations in the Wiskott-Aldrich syndrome ( WAS ) gene represent rare molecular causes of SCN. The relative frequency of these distinct gene defects among patients with SCN differs depending on ethnicity. In particular, in populations with a high degree of consanguinity, autosomal recessive variants of SCN are more prevalent than mutations in ELANE . Despite recent progress in the field, in around one-third of patients with SCN, no pathogenic mutations can be identified.
Disease | Gene Mutated | Inheritance | Hematopoietic Manifestations | Extrahematopoietic Manifestations | Pathophysiologic Mechanism | References |
---|---|---|---|---|---|---|
(1) SCN | ||||||
ELANE deficiency (SCN1) | ELANE | AD | CN or CyN | — | Activation of the UPR, excessive apoptosis of myeloid cells | |
GFI1 deficiency (SCN2) | GFI1 | AD | CN, lymphopenia | — | Defective myeloid cell differentiation | |
HAX1 deficiency (SCN3) | HAX1 | AR | CN | Epilepsy, neurologic impairment in some patients | Destabilization of mitochondrial membrane potential, excessive apoptosis of myeloid cells | |
G6PC3 deficiency (SCN4) | G6PC3 | AR | CN, thrombocytopenia | Congenital heart defects, facial dysmorphy, increased visibility of superficial veins, urogenital malformations, failure to thrive, endocrine abnormalities, inner ear hearing loss, hyperelasticity of the skin | Impaired intracellular glucose homeostasis, activation of the UPR, excessive apoptosis of myeloid cells | |
XLN | WAS | XL | CN, lymphopenia, myelodysplasia | — | Enhanced and discoordinated actin polymerization, defective cytokinesis | |
(2) Congenital neutropenia: hypopigmentation disorders | ||||||
Chédiak-Higashi syndrome | LYST / CHS1 | AR | CN, defective NK cell function, lysosomal inclusion bodies in leukocytes, macrophage activation syndrome | Oculocutaneous albinism, neurodegeneration | — | |
Hermansky-Pudlak syndrome, type 2 | AP3B1 | AR | CN, impaired function of T and NK cells | Oculocutaneous albinism | Defective endosomal function | |
Griscelli syndrome, type 2 | RAB27A | AR | CN, defective cytotoxicity, macrophage activation syndrome | Oculocutaneous albinism | — | |
P14/ROBLD3/MAPBPIP deficiency | P14 / ROBLD3 / MAPBPIP | AR | CN, defective cytotoxicity, growth failure, lymphoid immunodeficiency | Oculocutaneous albinism | Aberrant distribution of late endosomes | |
(3) Complex disorders comprising congenital neutropenia | ||||||
AK2 deficiency | AK2 | AR | CN, severe lymphopenia (reticular dysgenesis) | Inner ear hearing loss | Aberrant mitochondrial metabolism caused by lack of adenylate kinase 2 | |
Shwachman-Diamond syndrome | SBDS | AR | CN | Exocrine pancreatic insufficiency, skeletal dysplasia, hepatic and cardiac disease | Mitotic spindle destabilization, genomic instability, enhanced apoptosis | |
WHIM syndrome | CXCR4 | AD | CN, myelokathexis, B cell deficiency | Warts, hypogammaglobulinemia, immunodeficiency | Constitutive activating mutations in CXCR4 associated with myelokathexis and lack of neutrophils in peripheral blood | |
Poikiloderma with neutropenia | C16ORF57 | AR | CN, bone marrow abnormalities | Poikiloderma, increased photosensitivity | Disturbed biogenesis of U6 small nuclear RNA, impaired cell viability | |
Cartilage-hair hypoplasia | RMRP | AR | CN, immunodeficiency | Hypoplastic hair, skeletal dysplasia | Defective assembly of ribosomes, aberrant cell cycle control and telomere function | |
CD40L deficiency (HIGM1) | CD40L / CD154 | XL | Intermittent CN, combined immunodeficiency including T and B cell deficiency, defective B cell class switch (hyper-IgM syndrome type I) | — | Defective class switch caused by impaired CD40-CD40L interaction, defective T cell priming | |
Barth syndrome | G4.5 / TAZ | XL | CN (in most patients) | Cardioskeletal myopathy, growth impairment, carnitine deficiency | Mitochondrial dysfunction, excessive apoptosis in myeloid cells | |
Cohen syndrome | VPS13B / COH | AR | Intermittent CN | Psychomotor retardation, skeletal dysplasia, hypotonia | Suspected function of COH in vesicular transport processes | |
Pearson syndrome | Deletion of mitochondrial DNA | mtDNA | CN, bone marrow failure | Exocrine pancreas insufficiency, endocrine abnormalities, neuromuscular degeneration | — |
This article discusses some of the most recent findings and discusses their implications for molecular understanding of neutrophil biology.
Ethnic neutropenia
The most common genetically determined variant associated with congenital neutropenia occurs in areas of the world where Plasmodium vivax is endemic, such as Africa and some regions in the Middle East: a polymorphism in DARC (Duffy null) is associated with ethnic neutropenia and an increased risk of HIV infection on exposure, although it confers protection against P vivax infection. Ethnic neutropenia was described decades ago without knowledge of its genetic basis. In contrast with patients with SCN, ethnic neutropenia is not associated with severe infections. The molecular details of how the DARC polymorphism causes neutropenia and why affected individuals rarely show severe infections as expected for neutropenic patients have remained elusive.
HAX1 deficiency
Since the original description by Rolf Kostmann, it was evident that the classic variant of SCN is a disease with an autosomal recessive inheritance pattern. Using a combination of a genome-wide linkage analysis and candidate gene sequencing, a deleterious mutation in HAX1 was identified in 3 unrelated consanguineous kindreds from Turkey. The frequency of HAX1 mutations among patients with SCN is approximately 15%, with considerable differences in their ethnic backgrounds. Thus, a higher percentage of patients from Turkey and the Middle East bear HAX1 mutations, whereas mutations are rarer among the US population. HAX1 mutations were also discovered in descendants from the original families described by Rolf Kostmann in his sentinel article describing SCN as a novel disease entity more than 60 years ago.
A fraction of HAX1-deficient patients show, in addition to congenital neutropenia, neurologic disease manifestations such as cognitive impairment, developmental delay and/or epilepsy. These clinical observations could be associated with a clear genotype-phenotype pattern for 2 known isoforms of HAX1 : isoforms a and b. Although HAX1 mutations that affect exclusively isoform a show a phenotype of (isolated) congenital neutropenia, mutations affecting both isoforms are associated with additional neurologic aberrations. This view has recently been challenged by other investigators who described 1 patient with a compound heterozygous mutation in HAX1 without neurologic findings. Mice with a targeted deletion of Hax1 show increased neuronal cell apoptosis and a neurodegenerative disorder. The hematological aberrations of Hax1 deficiency in the murine knockout model comprise lymphopenia, including significant reduction of B cells but no overt neutropenia. The reasons for this species-related difference are unclear at present but are reminiscent of other disorders such as ELANE deficiency, in which the respective murine transgenic mouse does not model neutropenia. Thus, it is possible that murine neutrophil granulocytes behave differently with respect to resistance and susceptibility to apoptosis compared with human cells (see the article by Schäffer A elsewhere in this issue for more details).
HAX1 is a ubiquitously expressed protein that localizes predominantly to the mitochondria. Deficiency of HAX1 in hematopoietic and nonhematopoietic cells leads to destabilization of the mitochondrial membrane potential (Δψm), which explains the increased apoptosis observed in myeloid cells as well as nonhematopoietic cells such as fibroblasts. In this respect, the interaction of HAX1 with PARL (presenelin-associated, rhomboidlike) and Htr2A/Omi is intriguing, because activation of this pathway leads to activation of proapoptotic BAX. The antiapoptotic activity of HAX1 may alternatively be mediated through interaction with XIAP (X-linked inhibitor of apoptosis protein) by suppressing its polyubiquitination and thus protecting it from degradation in the proteosome. Another interesting observation with potential implications for the role of HAX1 in regulating apoptosis is that it interacts with phospholamban, a cardiac protein inhibiting SERCA2A, a sarcoplasmic reticulum Ca2+-ATPase. More recently, HAX1 was shown to directly interact with SERCA2A and downregulate its expression, which may modulate endoplasmic reticulum (ER) Ca2+ levels. Taken together, the molecular mechanisms that cause deficiency of HAX1 to lead to increased myeloid cell apoptosis are not yet clear. Nevertheless, it seems that HAX1 is a critical regulator of various cellular processes that are directly or indirectly associated with cell survival or apoptosis.
HAX1 interacts with a large number of other cytosolic proteins, including Gα13, Il1α, the polycystic kidney disease 2 (PKD2) protein, integrin αvβ6, and bile salt protein. These data suggest a role for HAX1 in intracellular transport and cell migration, and a recent study by Cavnar and colleagues revealed a critical role for HAX1 in regulating neutrophil adhesion and chemotaxis through modulation of RhoA signaling.
A large number of additional interaction partners have been identified to date, highlighting a complex proteomic network of HAX1. Interactions with viral proteins such as Karposi sarcoma–associated herpesvirus K15 protein (KSHV), Epstein-Barr virus (EBV) nuclear antigen 5 (EBNA5) and EBV nuclear antigen leader protein (EBNA-LP), HIV-vpr and HIV-rev, hepatitis C virus core protein, and swine fever virus N-terminal protease collectively suggest that several viruses may have usurped additional functions of HAX1 that have remained elusive to date. More recently, it has been recognized that HAX1 also interacts with the 3′untranslated region sequence of several mRNAs. Although the functional significance is not clear, these findings suggest a potential role of HAX1 in posttranscriptional control of mRNA processing, stability, and localization.
G6PC3 deficiency
A previously unrecognized immunodeficiency syndrome associating SCN and complex developmental aberrations has recently been described, caused by mutations in the glucose-6-phosphatase catalytic subunit 3 ( G6PC3 ) gene. G6PC3 is a member of the glucose-6-phosphatase family, consisting of G6PC1, G6PC2, and G6PC3 (reviewed by Hutton and O’Brien ).
G6PC1 is predominantly expressed in liver, gut, and kidney, and G6PC2 is expressed in β cells of the pancreas. G6PC3 is ubiquitously expressed. Mutations in G6PC1 cause glycogen storage disease type Ia, and, more recently, polymorphisms in G6PC2 have been associated with alterations in fasting glucose levels, implicating a critical role for G6PC2 in controlling system glucose levels, although a murine knockout model of G6pc2 does not show increased incidence or progression of type I diabetes. Mutations in G6PC3 have been observed in all exons of the gene, with partial clustering at exons 1 and 6, respectively, encoding for the N-terminal and C-terminal ends of the gene products. No clear-cut genotype-to-phenotype correlations have been observed, but it is possible that such effects will become more obvious when an even larger cohort of patients is available for systematic phenotypic analysis.
In addition to congenital neutropenia, intermittent or persistent thrombocytopenia is a feature of G6PC3 deficiency. Furthermore, G6PC3-deficient patients usually show extrahematopoietic manifestations such as congenital heart or urogenital defects. In a cohort of 28 systematically reviewed patients, congenital heart defects (24/28), unusual visibility of superficial veins (24/28), urogenital malformations (13/28), and facial dysmorphy (18/28) were the most frequent aberrations. Deficiency of G6PC3 may rarely manifest clinically by inflammatory bowel disease, a feature shared with glucose-6-phosphate translocase deficiency (authors’ own observations, see later discussion).
It has been known for a long time that patients with glycogen storage disease type Ib show a phenotype of congenital neutropenia combined with the typical features of glycogen storage disorder. The molecular cause of this disorder was subsequently clarified, when mutations in the SLC37A4 gene encoding the ubiquitously expressed glucose-6-phosphate transporter were found. Similar to G6PC1, G6PC3 is thought to act in a functional complex with G6PT on the membrane of the ER. In line with this model, functional deficiency of the G6PC1/G6PT complex is associated with glycogen storage disease, whereas deficiency of the G6PC3/G6PT complex causes congenital neutropenia (reviewed by Boztug and Klein ).
Similar to other genetically defined subforms of congenital neutropenia, including ELANE deficiency and HAX1 deficiency, G6PC3-deficient congenital neutropenia is associated with increased apoptosis in peripheral neutrophil granulocytes. In line with aberrant ER physiology, deficiency of G6PC3 is associated with increased apoptosis linked to the activation of the so-called unfolded protein response (UPR), as was also shown for ELANE-deficient congenital neutropenia. Studies in the respective murine knockout models have shown activation of the UPR, increased neutrophil apoptosis, and neutropenia in both G6pc3 -deficient as well as Slc37a4 -deficient mice.
G6PC3 deficiency has shown the critical importance of glucose homeostasis for neutrophil survival. In G6PC3 deficiency, glucose metabolism in neutrophil granulocytes is markedly disturbed with reduced levels of glucose uptake, glucose-6-phosphate, lactate, and adenosine triphosphate (ATP). Glucose deprivation using 2-dioxyglucose therefore leads to massive apoptosis in neutrophils from healthy controls, whereas other hematopoietic cells such as lymphocytes are less vulnerable. A glucose-sensitive apoptosis pathway involving glycogen synthase kinase 3β and the antiapoptotic Bcl2 molecule Mcl1 were described previously with Mcl1 as an essential antiapoptotic factor specifically in neutrophils. In G6PC3 deficiency, this pathway is activated, highlighting a nutrient-sensitive pathway of critical importance for survival of neutrophils. In G6PC3-deficient mice, treatment with granulocyte colony-stimulating factor (G-CSF) counteracts reduction of glucose-6-phosphate, lactate, and ATP and excessive apoptosis linked to activation of Akt and Caspase-3, and it is likely that G-CSF treatment has similar effects on human neutrophils from G6PC3-deficient patients. In the corresponding knockout mouse model, monocytes/macrophages also show a functional defect including impaired respiratory burst, chemotaxis, calcium flux, and phagocytosis linked to aberrant glucose metabolism. It is unclear at present whether these effects can also be observed in human G6PC3-deficient monocytes/macrophages.
Aberrant ER metabolism may entail not only a reduced threshold for apoptosis but also, more indirectly, on glycosylation patterns of various target proteins critical for neutrophil function, such as nicotinamide adenine dinucleotide phosphate hydrogen (NADPH) oxidase. As a consequence, G6PC3 deficiency can also be associated with qualitative neutrophil defects. Both quantitative and qualitative features of neutrophil dysfunction can be reversed by recombinant G-CSF therapy (Boztug and Klein, unpublished observation, 2010), a finding similar to the response in murine G6PC3-deficient neutrophils.
From a clinical point of view, most G6PC3-deficient patients respond well to low to moderate dosages of G-CSF with increase in neutrophil counts and decreasing frequency and severity of infections. In a cohort of 28 patients, we have not observed any transformation to myelodysplastic syndrome (MDS)/acute myeloblastic leukemia (AML) to date, suggesting that the risk of leukemogenesis may be lower in G6PC3-deficient SCN compared with other genetic subtypes of SCN. Patients with mutations in ELANE or HAX1 and also some patients with still unknown variants of SCN have a higher risk of developing a clonal hematopoietic disorder such as MDS or AML, which shows that delineation of the underlying genetic cause of SCN is of critical relevance for affected patients for long-term surveillance and tailored treatment decisions.
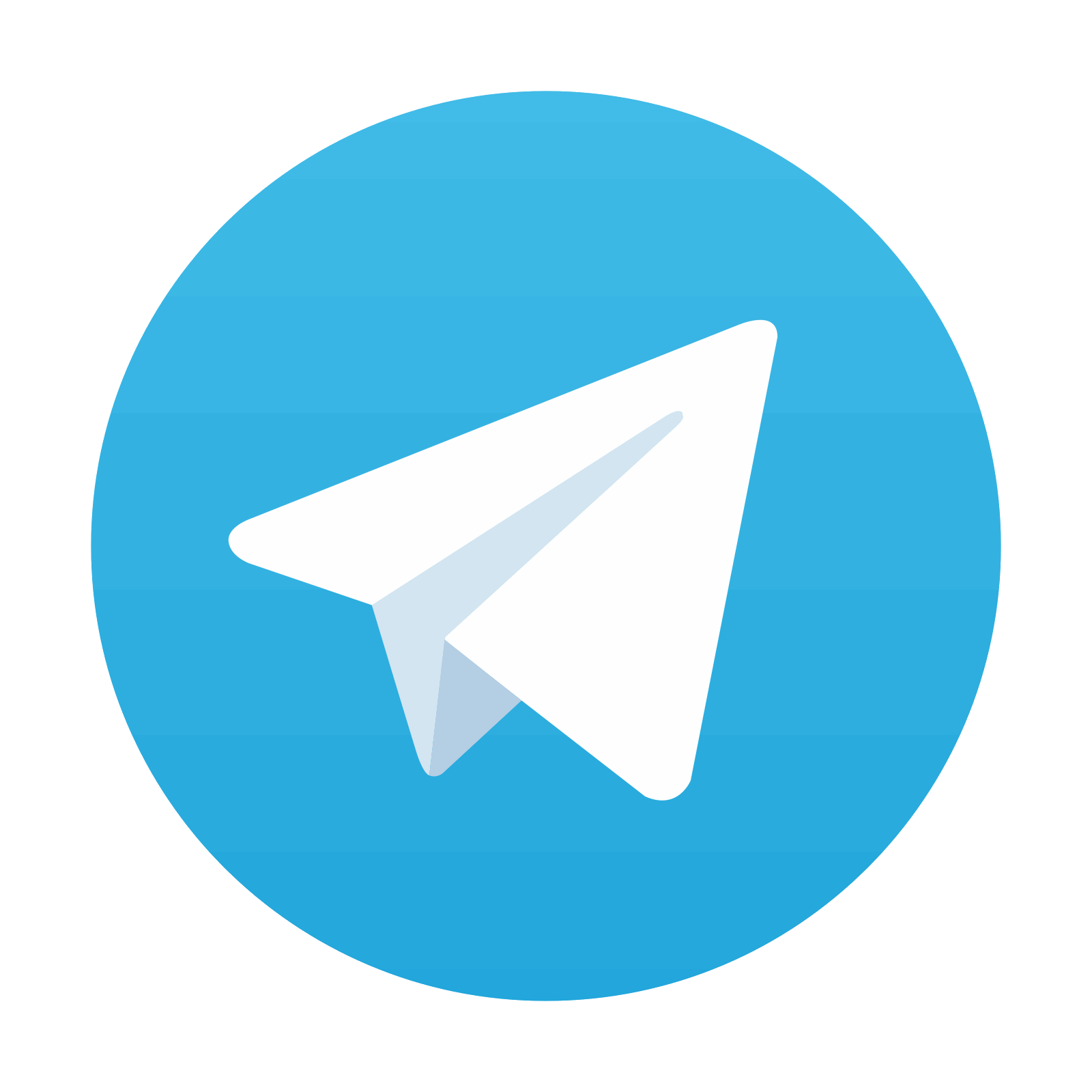
Stay updated, free articles. Join our Telegram channel

Full access? Get Clinical Tree
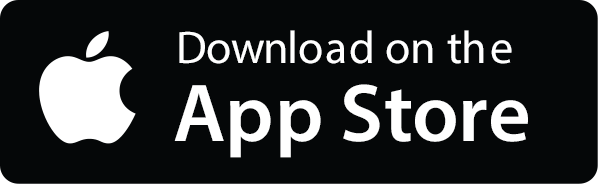
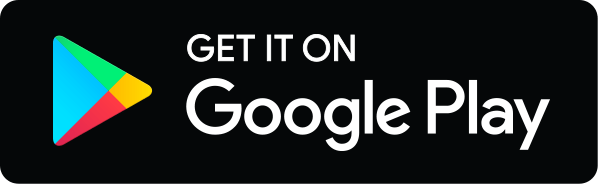