Objectives
- 1.
Describe the synchronization among fertilization, early embryonic events, and the human menstrual cycle.
- 2.
Describe the events involved in fertilization.
- 3.
Explain how implantation and placentation occur.
- 4.
Discuss the endocrine and transport functions of the placenta.
- 5.
Describe the development of the fetal endocrine system.
- 6.
Discuss maternal endocrine changes during pregnancy.
- 7.
Discuss the current models for the initiation and progression of labor (parturition) in humans.
- 8.
Describe the development and regulation of the mammary glands.
- 9.
Discuss the endocrine basis for contraception, the “morning after” pill, and the abortion pill.
Human reproduction involves internal insemination, internal fertilization, and internal gestation, all within the female tract. Internal gestation also involves the development of a transient organ, the placenta. The placenta is remarkable in that it is composed of tissues from two organisms:
- •
An extraembryonic membrane (called the chorion ) of the fetus
- •
Endometrial tissue (called decidua ) of the mother
From an endocrine point of view, pregnancy represents a state in which three separate endocrine systems— maternal, fetal, and placental —interact to promote adequate growth and nutrition of the fetus, the timing of parturition, and preparation of the maternal mammary glands to support extrauterine life of the fetus.
Fertilization, Early Embryogenesis, Implantation, and Placentation
Synchronization With Maternal Ovarian and Reproductive Tract Function
Fertilization, early embryogenesis, implantation , and early gestation are all synchronized with the human menstrual cycle ( Fig. 11.1 ). Just before ovulation, the ovary is in the late follicular stage and produces high levels of estrogen . Estrogen promotes growth of the uterine endometrium and induces expression of the progesterone receptor. Estrogen ultimately induces the luteinizing hormone (LH) surge , which in turn induces meiotic maturation of the oocyte and ovulation of the cumulus-oocyte complex.
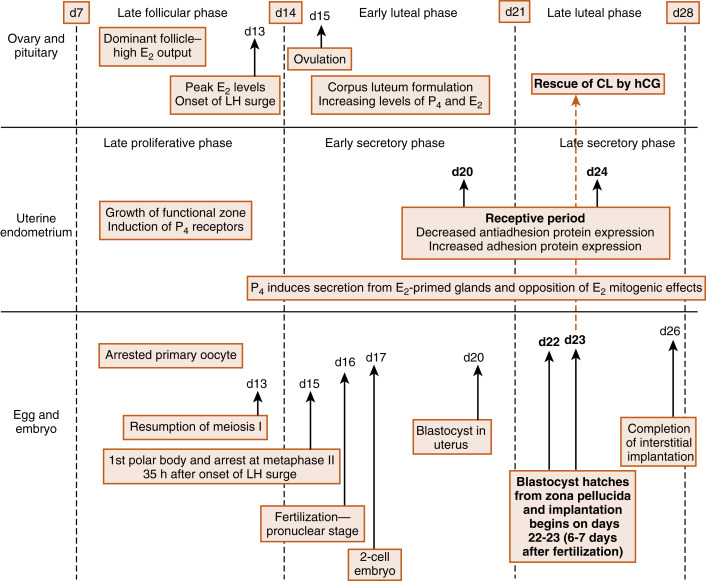
The events between fertilization and implantation take about 6 days to complete, and implantation occurs at about day 21 of the menstrual cycle. At this time, the ovary is in the midluteal phase , secreting large amounts of progesterone . Progesterone stimulates secretion from uterine glands, which provide nutrients to the embryo. This is referred to as histotrophic nutrition and is an important mode of maternal-to-fetal transfer of nutrients for about the first trimester of pregnancy, after which it is replaced by hemotrophic nutrition. Progesterone inhibits myometrial contraction and prevents the release of paracrine factors that lead to menstruation.
Progesterone induces the window of receptivity in the uterine endometrium, which exists from about day 20 to 24 of the menstrual cycle. This receptive phase is associated with increased adhesivity of the endometrial epithelium and involves the formation of cellular extensions, called pinopodes , on the apical surface of endometrial epithelia, along with increased expression of adhesive proteins (e.g., integrins, cadherins) and decreased expression of antiadhesive proteins (e.g., mucins) in the apical cell membrane.
Thus during the time it takes a fertilized egg to implant in the uterus, the uterine endometrium is at its full thickness, actively secretory, and capable of tightly adhering to the implanting embryo. It should also be noted that the uterine endometrium is well vascularized at the time of implantation. Spiral arteries extend to the basal lamina of the surface epithelium (see Fig. 10.17 in Chapter 10 ) and give rise to rich capillary beds and postcapillary venous lakes (also called lacunae) . Apart from its nutrient supply to all cells of the endometrium, the extensive blood supply immediately adjacent to the surface epithelium plays a critical role in capturing embryonic human chorionic gonadotropin (hCG ) and transporting hCG to the ovary, where it rescues the corpus luteum. The rich endometrial blood supply is also important for efficient delivery of progesterone to the endometrium.
Fertilization
Fertilization accomplishes both the recombination of genetic material to form a new, genetically distinct organism and the initiation of events that begin embryonic development. There are several steps that must occur for successful fertilization to be achieved. The sperm must find its way to the egg , and the sperm and the egg must contact, recognize one another, and fuse. After sperm-egg fusion, an intracellular signaling cascade occurs within the egg that has two major consequences. First, it allows the egg to regulate sperm entry such that only one sperm can fuse with the egg. This prevents polyspermy , which is lethal. Second, it “wakes up” the metabolically quiescent egg so that it can resume meiosis and begin embryonic development. This process is called egg activation .
Spermatozoa present in the male ejaculate enter the vagina near the cervix and must reach the ampulla of the oviduct where fertilization occurs. Sperm transport is largely dependent on the female reproductive tract and, while the sperm are still in the uterus, is essentially independent of swimming. Large numbers of sperm in the ejaculate generally are required for successful fertilization of the egg by a single sperm—of the 300 million sperm typically ejaculated, only about 200 reach the oviduct. Clinically, males with fewer than 20 million sperm per mL of ejaculate are considered to be infertile .
The female reproductive tract is an important regulator of sperm transport. Toward the end of the follicular phase of the menstrual cycle, before ovulation, estrogen levels are high. Estrogen causes the cervix to produce a watery mucus , often called “egg white cervical mucus” because of its consistency. This mucus forms channels to aid the passage of sperm through the cervix, and only motile sperm can pass through this barrier. Estrogen also causes contractions of the myometrium to help propel sperm upward toward the oviduct (i.e., cervical-to-fundal contractions).
Sperm must undergo a process called capacitation in the female reproductive tract before they are able to fertilize the egg. Sperm capacitation is an incompletely understood, transient event that occurs largely in the oviduct and modifies the spermatozoan in several ways so that it becomes capable of fertilizing the egg. These changes include the following:
- 1.
An altered membrane fluidity due to the removal of cholesterol from the sperm membrane
- 2.
The removal of proteins and carbohydrates from the membrane that may otherwise block sites that bind to the egg
- 3.
A change in membrane potential that may permit Ca 2+ to enter the sperm and thereby facilitate the acrosome reaction (see later in the text)
- 4.
Phosphorylation of numerous proteins
Incapacitated sperm bind actively to the epithelial cells of the oviductal isthmus and become unbound when they are capacitated. This binding slows the capacitation process, extends the sperm life span, prevents too many sperm from reaching the egg, and increases the probability that sperm will be in the oviduct when the egg is ovulated. Sperm can therefore survive in the female reproductive tract for several days. Hyperactivation is another phenomenon that occurs in the oviduct. Hyperactivation involves a change in flagellar motion from a wavelike to a whiplike motion and is caused by Ca 2+ entry through sperm-specific Ca 2+ channels called CatSper channels , which are activated by progesterone produced by the cumulus cells. This whiplike flagellar movement is necessary for the sperm to detach from the oviductal epithelium, is well suited to swimming through the thick oviductal fluid, and helps propel the sperm through the outer layers of the egg to reach the egg’s plasma membrane.
Capacitated sperm reach the egg, surrounded by its expanded cumulus cells , in the ampulla of the oviduct. Fertilization involves the penetration of the egg by the sperm. To do this, the sperm must breach three barriers ( Fig. 11.2 ):
- •
Expanded cumulus
- •
Zona pellucida
- •
Plasma membrane of the egg (called the oolemma )
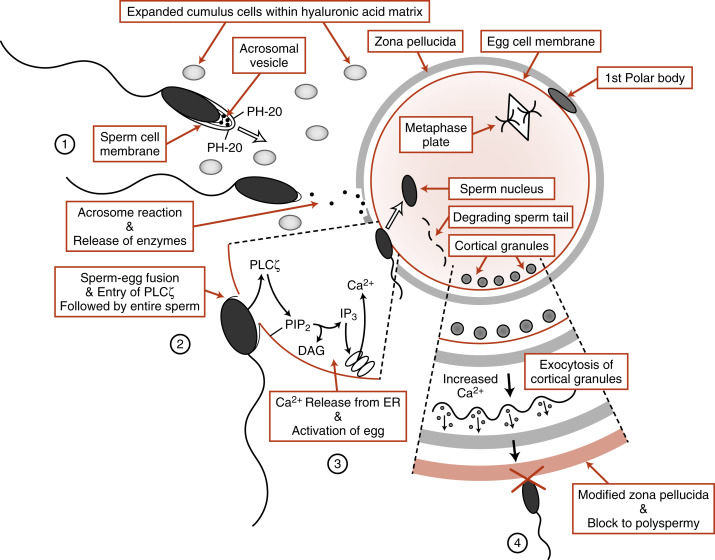
The cumulus cell matrix is composed predominantly of hyaluronic acid , and the sperm are able to digest through this layer with a membrane-bound hyaluronidase called PH-20 (also called sperm adhesion molecule 1, or SPAM1 ) (see Fig.11.2 , step 1). The next obstacle the sperm encounters is the zona pellucida , an extracellular coat made up of four glycoproteins ( ZP1, ZP2, ZP3, and ZP4 ). Sperm transport through the zona is achieved by the acrosome reaction , a Ca 2+ -dependent event in which the sperm plasma membrane fuses with the outer acrosomal membrane to release the contents of the acrosomal vesicle (see Fig. 11.2 , step 2). The enzymes released from the acrosomal vesicle then digest the zona pellucida. The acrosome reaction appears to begin while the sperm is in contact with the cumulus cells and may be enhanced through the binding to zona pellucida proteins.
The molecular mechanisms involved in the interactions of the sperm and egg plasma membranes are not completely understood. Sperm and eggs recognize and bind to each other through the proteins IZUMO1 and JUNO , respectively. Sperm-egg fusion is likely to involve tetraspanin proteins , such as CD9, in the egg (see Fig. 11.2 ; step 3).
The entire sperm enters the egg during fusion. The flagellum and mitochondria disintegrate, so most of the mitochondrial DNA in cells is maternally derived. Once the sperm is inside the egg, protamines associated with the tightly condensed sperm DNA are uncoiled by the highly reducing egg cytoplasm, causing decondensation of the sperm DNA. A membrane called the pronucleus forms around the sperm DNA while the newly activated egg completes the second meiotic division .
The egg is a metabolically quiescent cell that is “woken up” as a result of sperm-egg fusion, in a process called egg activation . All of the events associated with egg activation depend on intracellular release of Ca 2+ in the egg . Ca 2+ release is stimulated by the production of inositol 1,4,5-trisphosphate (IP3) in response to the sperm-specific phospholipase C enzyme, PLCζ which is introduced into the egg upon sperm-egg fusion (see Fig. 11.2 , step 3). PLCζ cleaves the egg membrane phospholipid, phosphatidylinositol-4,5-bisphosphate (PIP 2 ), to form IP 3 and diacylglycerol. IP 3 binds to its receptor on the endoplasmic reticulum and opens Ca 2+ channels (see Chapter 1 ). In human eggs, an initial burst of Ca 2+ occurs soon after sperm-egg fusion that is followed by a series of Ca 2+ oscillations that last for several hours after fertilization. These Ca 2+ pulses are absolutely required for all the major events of egg activation.
One of the earliest Ca 2+ -dependent events that occurs at fertilization of mammalian eggs is the prevention of polyspermy. Enzyme-filled vesicles, called cortical granules , reside in the outermost, or cortical, region of the unfertilized egg (see Fig. 11.2 , step 4). In response to increases in intracellular Ca 2+ , these vesicles translocate to the plasma membrane and release hydrolytic enzymes through exocytosis. These enzymes modify ZP2, generating ZP f , which blocks binding of any more sperm. Thus only one sperm usually enters the egg. Occasionally, more than one sperm does enter the egg. This results in a triploid cell , which is lethal. It is thought that up to 10% to 15% of miscarriages are caused by polyspermy.
Ca 2+ release also stimulates the egg to reenter the cell cycle, complete meiosis , and recruit maternal messenger RNAs (mRNAs ) after fertilization. The unfertilized egg is held in meiotic arrest at metaphase of meiosis II by the cell cycle regulatory protein complex , maturation-promoting factor (MPF) , as well as cytostatic factor (CSF), which contains components of the mitogen-activating protein kinase (MAPK) pathway . Ca 2+ -calmodulin–dependent pathways inactivate both MPF and CSF so that the metaphase II chromosomes decondense, the anaphase-promoting complex becomes active, and the egg can form a pronucleus . The unfertilized egg is transcriptionally inactive, and Ca 2+ release at fertilization is also needed for the recruitment of stored maternal mRNAs for translation into maternally derived proteins needed for early embryonic development.
The activated egg completes the second meiotic division while the sperm DNA decondenses and a pronucleus forms around it ( Fig. 11.3 ). Once the egg has completed meiosis, a pronucleus forms around the female chromosomes as well. A centrosome , contributed by the sperm, becomes a microtubule-organizing center from which microtubules extend until they contact the female pronucleus. The male and female DNAs replicate while the two pronuclei are pulled together. Once the pronuclei contact each other, the nuclear membranes break down, the chromosomes align on a common metaphase plate, and the first embryonic cleavage occurs.
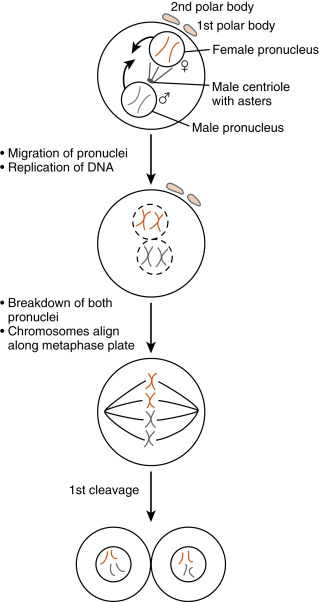
Early Embryogenesis and Implantation
Fertilization typically occurs on day 15 or 16 of the menstrual cycle, and implantation occurs about 6 days later. Thus the first week of embryogenesis occurs within the lumina of the oviduct and uterus ( Fig. 11.4 ). For most of this time, the embryo remains encapsulated by the zona pellucida . The first two cleavages take about 2 days, and the embryo reaches a 16-cell morula by 3 days. The outer cells of the morula become tightly adhesive with each other and begin transporting fluid into the embryonic mass. During days 4 and 5, the transport of fluid generates a cavity, called the blastocyst cavity (blastocoele), and the embryo is now called a blastocyst ( Fig. 11.5 ). The blastocyst is composed of two subpopulations of cells:
- •
The eccentric inner cell mass
- •
An outer, epithelial-like layer of trophoblasts. The region of trophoblast layer immediately adjacent to the inner cell mass is referred to as the embryonic pole, and it is this region that attaches to the uterine endometrium at implantation.
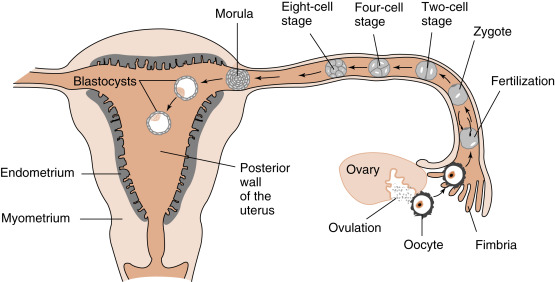
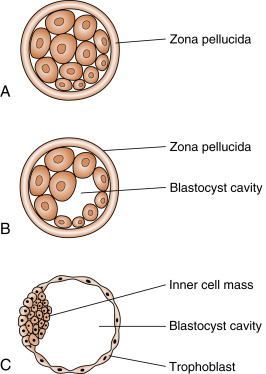
The embryo resides within the oviduct during the first 3 days and then enters the uterus. By 5 to 6 days of development, the trophoblasts of the blastocyst secrete proteases that digest the outer-lying zona pellucida. At this point, corresponding to about day 22 of the menstrual cycle, the hatched blastocyst ( Fig. 11.6 ) is able to adhere to and implant into the receptive uterine endometrium.
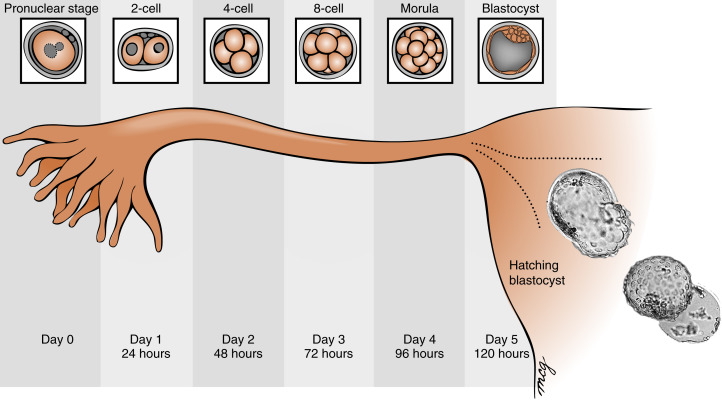
At the time of attachment and implantation , the trophoblasts differentiate into two cell types: an inner layer of cytotrophoblasts and an outer layer of multinuclear and multicellular syncytiotrophoblasts ( Fig. 11.7 ). The cytotrophoblasts initially provide a feeder layer of continuously dividing cells. Syncytiotrophoblasts perform three general types of functions: adhesive, invasive, and endocrine . Syncytiotrophoblasts express adhesive surface proteins (i.e., cadherins and integrins) that bind to uterine surface epithelia, and as the embryo implants, these bind to components of the uterine extracellular matrix. In humans, the embryo completely burrows into the superficial layer of the endometrium (see Fig. 11.7 ). This mode of implantation, called interstitial implantation , is the most invasive among placental mammals. Interstitial implantation involves adhesion-supported invasion and migration of syncytiotrophoblasts into the endometrium, along with the breakdown of extracellular matrix by the secretion of matrix metalloproteases and other hydrolytic enzymes.
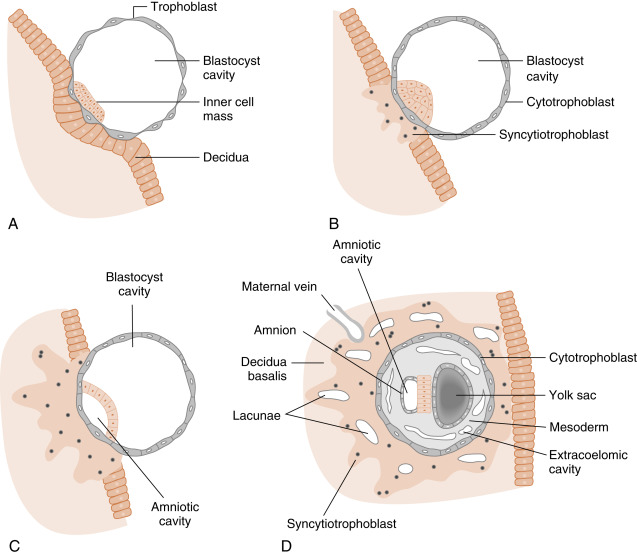
The endocrine function begins with the onset of implantation, when syncytiotrophoblasts begin secreting the LH-like protein hCG (see later in the text), which maintains the viability of the corpus luteum ( corpus luteum of pregnancy ) and, thus, maintains progesterone secretion. Syncytiotrophoblasts become highly steroidogenic by 10 weeks and make progesterone at sufficient levels to maintain pregnancy independently of the corpus luteum. Syncytiotrophoblasts produce several other hormones, as well as enzymes that modify hormones (see later in the text).
While implantation and placentation progress, syncytiotrophoblasts take on the important functions of phagocytosis (during histotrophic nutrition) and bidirectional placental transfer of gases, nutrients, and wastes. Exchange across the syncytiotrophoblasts involves diffusion (e.g., gases), facilitated transport (e.g., GLUT1-mediated transfer of glucose), active transport (e.g., amino acids by specific transporters), and pinocytosis-transcytosis (e.g., of iron-transferrin complexes).
There also is a maternal response to implantation, called decidualization , which involves the transformation of the endometrial stroma into enlarged and glycogen-filled decidual cells (the endometrium is now referred to as the decidua ). The decidua forms an epithelial-like sheet with adhesive junctions that inhibit migration of the implanting embryo. The decidua also secretes factors, such as tissue inhibitors of metalloproteases (TIMPs), which moderate the activity of syncytiotrophoblastic-derived hydrolytic enzymes in the endometrial matrix. Consequently, decidualization allows for regulated invasion during implantation.
Normally, the implanting embryo and placenta do not extend to and involve the myometrium . Placental accreta is the destruction of the endometrium and adherence of the placenta to the myometrium, a condition associated with potentially life-threatening postpartum hemorrhage . It is important to note that the decidual response occurs only in the uterus. Thus the highly invasive nature of the human embryo poses considerable risk to the mother in the case of ectopic implantations . The most common site of ectopic implantation is the oviduct (giving rise to a tubal pregnancy ), but implantations also rarely occur in the ovary and cervix and within the abdominal cavity.
Structure of the Mature Placenta
The progression of placental development is complicated, and the reader is referred to embryology texts for a more complete discussion than the one presented here. It is useful to consider placental development with a focus first on the entire gravid uterus and then on the fine structure of the mature placenta.
Initially, the growing syncytiotrophoblasts extend evenly from the embryo into the outer-lying decidua. At about 9 days, spaces appear within the syncytiotrophoblast layer, called lacunae . These spaces become filled with the secretions of endometrial glands, maternal blood from degraded vessels, and the remnants of enzymatically digested matrix, referred to as the embryotroph , which provides for histotrophic nutrition ( Fig. 11.8 ).
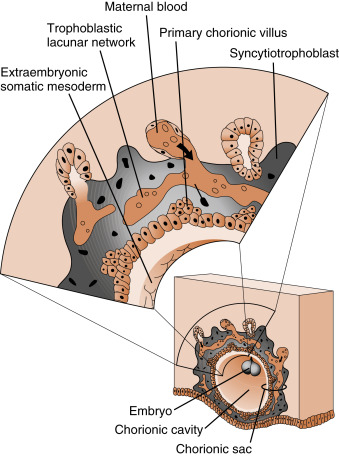
By the end of the second week of development, the columns of syncytiotrophoblasts with a core of cytotrophoblasts are distinguishable as primary villi ( Fig. 11.9 ). By this time, a new extraembryonic layer, called extraembryonic mesoderm , becomes associated with the cytotrophoblast and syncytiotrophoblast layers. The three layers are now referred to as the chorionic membrane . After primary villi gain a mesodermal core, they are referred to as secondary villi. The extraembryonic mesoderm provides a connection, called the connecting stalk, between the chorion and the embryo . It is within this mesoderm that the fetal ( umbilical ) circulation develops. Once villi contain umbilical blood vessels, they are referred to as tertiary villi (see Fig. 11.9 ). Chorionic villi represent the functional unit of the placenta and, through extensive branching, greatly increase the surface area for maternal-fetal exchange.
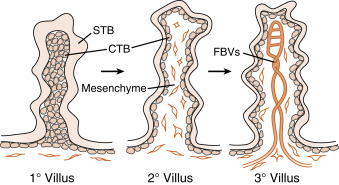
Although villi develop from the entire spherical chorionic membrane, they quickly degenerate around most of the chorion, forming a smooth chorion, or the chorion laeve ( Fig. 11.10 ). In the region of the original embryonic pole, however, the chorion develops into a highly branching villous chorion, called the chorion frondosum (see Fig. 11.10 ). The chorion frondosum represents the fetal side of the mature placenta.
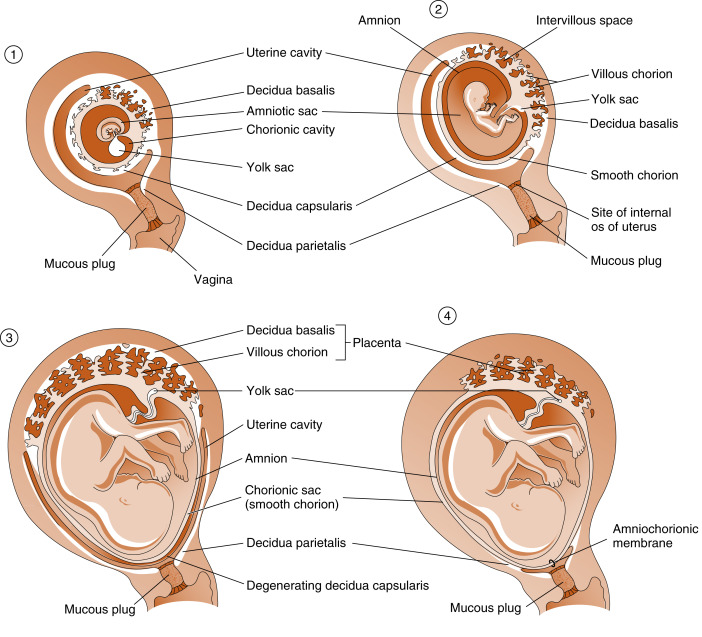
The uterine decidua immediately apposed to the chorion frondosum is called the decidua basalis and forms the maternal side of the mature placenta (see Fig. 11.10 ). The decidua that is subjacent to the chorion laeve is called the decidua capsularis . With time, the decidua capsularis fuses with the decidua parietalis , which is the part of the uterine endometrium that is not directly associated with the chorionic membrane (see Fig. 11.10 ). This means that the original uterine lumen is obliterated. The decidua capsularis ultimately degenerates.
Another extraembryonic membrane, called the amnion , grows and surrounds the developing fetus. The amnion becomes a fluid-filled sac, allowing for a nonadhesive environment in which the fetus can develop. By the beginning of the third trimester, the amnion fuses with the chorion, forming the amniochorionic membrane , which in turn fuses with the decidua parietalis (see Fig. 11.10 ). With the disappearance of the decidua capsularis, only the fetal amniochorionic membrane stretches across the internal opening of the cervical canal, and it is the amniochorionic membrane that ruptures during childbirth.
The mature placenta ( Fig. 11.11 ) is composed of three major structures:
- 1.
The chorionic villi , which are lined externally by the syncytiotrophoblast layer and contain the termini of umbilical blood vessels within their core. While chorionic villi branch, they become increasingly smaller, thinner, and more involved in maternal-fetal exchange. The smallest villi, called terminal villi , are the predominant sites of maternal-fetal exchange ( Fig. 11.12 ). Terminal villi have an outer layer of syncytiotrophoblasts, which becomes extremely thin in certain regions. Subjacent to the thinnest regions of syncytiotrophoblasts, the cytotrophoblasts disappear, and an umbilical capillary presses against the syncytiotrophoblast layer. Thus nutrients from the maternal blood that bathes the terminal villi (see intervillous space in next entry) have to cross only a single, flat layer of syncytiotrophoblast, the fused basal lamina of the syncytiotrophoblast and capillary endothelium, and a flattened umbilical endothelial cell. This barrier between maternal blood and the umbilical circulation is called the placental membrane (see Fig. 11.12 ) and is also called a vasculosyncytial membrane . It represents the thinnest barrier to maternal-fetal exchange among placental (i.e., eutherian) mammals.
