Abstract
Infertility affects approximately 13% of women and 10% of men. The major causes of female infertility are anovulation, fallopian tube disease, pelvic adhesions, endometriosis, and unexplained infertility. Initial treatment for women with anovulatory infertility involves a sequential approach, moving from less to more resource-intensive therapies. Interventions that increase fecundability in anovulatory women include optimization of weight, letrozole or clomiphene ovulation induction for women with the polycystic ovary syndrome, and gonadotropin injections or pulsatile gonadotropin-releasing hormone (GnRH) therapy for women with functional hypothalamic amenorrhea. For women with distal tubal disease, effective treatments include in vitro fertilization (IVF) or laparoscopic tubal surgery. For couples with unexplained infertility, clomiphene plus intrauterine insemination (IUI), gonadotropin plus IUI and IVF are effective treatments. Most women who are infertile will conceive with fertility therapy.
Keywords
Anovulation, diminished ovarian reserve, tubal infertility, unexplained infertility, clomiphene, letrozole, metformin, chlamydia, hysterosalpingogram, fimbrioplasty, neosalpingostomy
Fertility is defined as the capacity to conceive and produce offspring. Infertility is the state of a diminished capacity to conceive and bear offspring. In contrast to sterility, infertility is not an irreversible state. The current clinical definition of infertility is the inability to conceive after 12 months of frequent coitus. Infertility prevalence is approximately 13% among women and 10% among men. Among women and men with infertility, 57% and 53%, respectively, were reported to seek infertility treatment. Women with higher income and more frequent use of the healthcare system are more likely to seek infertility treatment. Among women, the prevalence of infertility increases with age. In one study, at 32 and 38 years of age, 12% and 21% of women reported infertility, respectively. Since the fertility potential of the female partner decreases after 35 years of age, most authorities recommend initiating an infertility evaluation after 6 months of attempting conception in women 35 to 40 years of age and after 3 months in women over 40 years of age. Women with known causes of infertility, such as amenorrhea, should immediately start an evaluation to assess the cause and plan the treatment.
Statistical Model of Infertility
- ◆
The cumulative probability of conception, F , through month N , is calculated as follows: F = 1 − (l − f ) N , where f is the per cycle pregnancy rate.
The clinical definition of infertility is relatively crude because it does not reflect the wide range of fertility potential in couples that have not conceived after 12 months. The clinical definition of infertility implies the existence of a dichotomous state, either a pregnancy is achieved in 12 months and infertility is not present, or a pregnancy is not achieved in 12 months and, by definition, infertility is present. The current clinical definition of infertility is similar to analyzing a continuous variable, such as height, by using a dichotomous variable: “short” and “tall.” Height is clearly much better described by a continuous measure, such as centimeters, rather than by using a dichotomous variable like “short” and “tall.”
Our clinical approach to fertility and infertility would be advanced by an increased use of the statistical concept of fecundability in the fertility literature. Fecundability is the probability of achieving a pregnancy in one menstrual cycle. Fecundability is approximately 0.25 in healthy young couples just beginning attempts at conception. A related concept, fecundity , is the ability to achieve a pregnancy that results in a live birth based on attempts at conception in one menstrual cycle. Fecundability, a population estimate of the probability of achieving pregnancy in one menstrual cycle, is a valuable clinical and scientific concept because it creates a framework for the quantitative analysis of fertility potential. Based on the clinical characteristics of a population of infertile couples, the estimated fecundability may range from 0.00 in couples with an azoospermic male partner to approximately 0.04 in couples where the female partner has early stage endometriosis.
Fecundability provides a convenient quantitative estimate of the efficacy of various fertility treatments. An infertile couple with an estimated fecundability of 0.04, if left untreated, may have the choice of two approaches to the treatment of their fertility: a low-cost treatment (clomiphene plus intrauterine insemination [IUI]) that will increase fecundability to 0.08, or an expensive treatment (in vitro fertilization [IVF]) that will increase fecundability to 0.30. A clear quantitative presentation of the potential effect of each treatment on fecundability should assist the couple in choosing an optimal treatment plan. A practical problem with using fecundability as a central concept in infertility care is that the prediction models for estimating the fecundability of a couple are not well developed or validated. Factors that are important in estimating fecundability for a couple are age of the female partner, number of motile sperm, duration of subfertility, and presence of primary or secondary infertility.
The concept of fecundability can be used to derive a simple statistical description of the fertility process. Fecundability (f) is defined as the probability of conceiving during any one cycle. The probability of failing to conceive during any one cycle is 1 − f . Over a short period of time, the fecundability of a population is often stable. For a large group of couples, the probability of conception is f for the first month, f × (1 − f ) for the second month, f × (1 − f ) 2 for the third month, and f × (1 − f ) N −1 for the N th month. Using this model, the mean number of months required to achieve conception is 1/ f . The cumulative probability of conception, F , through month N is calculated as follows: F = 1 − (l − f ) N . Based on this simple statistical model, assuming a normal menstrual cycle fecundability of 0.25 and starting with 100 couples, approximately 98 couples should conceive within 13 cycles. If each cycle is 28 days, then 98% of couples should conceive within one calendar year (13 cycles × 28 days/cycle = 364 days).
Over a short period of follow-up, a population of couples attempting pregnancy behaves in a statistically stable manner, with a fixed proportion of the cohort becoming pregnant with each additional cycle of follow-up. As the follow-up is extended, however, the fecundability of the nonpregnant couples declines and the cumulative pregnancy rate approaches an asymptote, which is less than 100%. Conceptually, this issue can be managed by assuming that there is an asymptote to the cumulative pregnancy rate of the population, or by using complex mathematical modeling of the population fecundability based on the assumption that couples in the population have a range of per cycle pregnancy rates. This issue is of special importance in the analysis of fertility rates in populations over long periods of time, such as 2 years. This issue is of less practical importance in studies where the time period for analysis is 3 to 6 cycles.
Many studies report that the observed fecundability of a population diminishes with long-term follow-up. For example, Guttmacher assessed the number of months to conception in 5574 women who achieved pregnancy between 1946 and 1956. During the first 3 months of observation, the fecundability was 0.25. During the next 9 months of observation, the fecundability was 0.15. Zinaman et al. studied 200 healthy couples who wanted to conceive. During the first 3 months of observation, the fecundability was 0.25. During the next 9 months of observation, the fecundability was 0.11 ( Table 22.1 ). Other investigators have reported similar results.
Cycle | Number of Women Available for Study at Start of Cycle | Number of Pregnancies in Cycle | Per-Cycle Pregnancy Rate |
---|---|---|---|
1 | 200 | 59 | 0.30 |
2 | 137 | 41 | 0.30 |
3 | 95 | 16 | 0.17 |
4 | 78 | 12 | 0.15 |
5 | 66 | 14 | 0.21 |
6 | 52 | 4 | 0.08 |
7 | 48 | 5 | 0.10 |
8 | 43 | 3 | 0.07 |
9 | 40 | 2 | 0.05 |
10 | 38 | 1 | 0.03 |
11 | 37 | 2 | 0.05 |
12 | 35 | 1 | 0.03 |
The decrease in fecundability with time suggests that each large population consists of a heterogeneous mixture of couples. Some couples have completely normal fertility and achieve pregnancy at a high rate (0.25 per cycle). The remaining couples have a lower fecundability (ranging from 0.00 to 0.15). Some of the couples in this pool will eventually present to a clinician for the treatment of infertility. At the end of 12 months of attempting conception, the couples that have not achieved conception have a fecundability in the range of 0.00 to 0.04 if left untreated (see Table 22.1 ). Successful interventions to improve fertility must increase the per-cycle pregnancy rate over the spontaneous pregnancy rate.
Unfortunately, not all pregnancies produce a live birth. Many pregnancies are lost soon after implantation. The terms occult pregnancy and chemical pregnancy are often used to describe these early pregnancy losses. Occult pregnancy was defined by Bloch as a pregnancy that terminates so soon after implantation that there was no clinical suspicion of its existence. In one recent study, approximately 13% of pregnancies were occult. Unlike occult pregnancies, a chemical pregnancy typically occurs in the presence of a clinical suspicion that a pregnancy may exist. A blood or urine human chorionic gonadotropin (hCG) assay demonstrates the presence of a pregnancy, but no clinical evidence of the pregnancy is detectable by ultrasound. Of all clinical pregnancies, approximately 20% result in a spontaneous abortion. Of all pregnancies, approximately 30% are lost—either as occult, chemical, or clinical spontaneous abortions ( Table 22.2 ). Women older than 40 years of age who become pregnant have a spontaneous abortion rate more than double that observed in women younger than 30 years of age.
Cycle Outcome | Cycle Numbers | |||
---|---|---|---|---|
1 | 2 | 3 | Total | |
Not pregnant at start of cycle | 200 | 137 | 95 | |
Pregnant during cycle | 59 | 41 | 16 | 116 |
Chemical pregnancy | 7 | 7 | 1 | 15 |
Spontaneous abortion | 12 | 5 | 4 | 21 |
Live births | 40 | 29 | 10 | 79 |
Dropped out, not pregnant | 4 | 1 | 1 | 6 |
Lost to follow-up | 1 |
Diseases Associated With Infertility
- ◆
The most common causes of female infertility are anovulation, tubal disease, pelvic adhesions, and endometriosis. Many couples do not have an identifiable cause of infertility, and this condition is referred to as unexplained infertility.
Pregnancy is the result of the successful completion of a complex series of physiological events occurring in both the male and female that allows for the implantation of an embryo in the endometrium ( Fig. 22.1 ). At a minimum, pregnancy requires ovulation and the production of a competent oocyte, production of competent sperm, proximity of the sperm and oocyte in the reproductive tract, transport of the embryo into the uterine cavity, and implantation of the embryo into the endometrium.

Some diseases, such as those that cause azoospermia, clearly have a cause-effect relationship with infertility. For other disease processes, such as stage I endometriosis, there is no clear cause-effect relationship between the disease and the infertile state. In these situations, it is preferable to state that there is an association between the disease condition and the infertile state, but that causality has not been definitively established. Due to the limits of our current understanding of fertility in humans, it is often difficult to categorize disease conditions as either causing infertility (e.g., azoospermia) or associated with infertility (e.g., stage I endometriosis). Consequently, a discussion of the distribution of reproductive diseases that are diagnosed in infertile couples is not necessarily based on hard scientific data, but rather descriptive observations and assumptions about diseases that cause or might be associated with infertility.
Most tabulations of the medical conditions that “cause” infertility divide the problem into male factors and female factors. The World Health Organization (WHO) task force on Diagnosis and Treatment of Infertility conducted a study of 8500 infertile couples using a standardized diagnostic protocol. In developed countries, diseases that were identified as contributing to the infertile state were attributed to the female partner in 37% of couples, to the male partner in 8% of couples, and to both partners in 35% of couples. Five percent of the couples had no identifiable cause of infertility (unexplained infertility) and 15% of the couples became pregnant during the investigation. The diseases in the female most often identified were ovulatory disorders (25%), unexplained infertility (20%), endometriosis (15%), pelvic adhesions (12%), tubal occlusion (11%), other tubal abnormalities (11%), and hyperprolactinemia (7%).
In a review of 21 published reports containing a total of 14,141 infertile couples, Collins reported that the primary diagnoses in the couples were: ovulatory disorders (27%), abnormal semen parameter (25%), tubal defect (22%), unexplained (17%), endometriosis (5%), and other causes (4%), as shown in Fig. 22.2 . In another data set of 2198 infertile couples, the distribution of primary diagnoses was unexplained (26%), abnormal semen parameter (24%), tubal disease (23%), ovulatory disorders (18%), endometriosis (6%), and other (3%). These observations can be broadly grouped into five major conditions that influence fecundability:
- 1.
Abnormalities in the production of a competent oocyte (anovulation, depletion of the oocyte pool, or poor oocyte function/quality)
- 2.
Abnormalities in reproductive tract transport of the sperm, oocyte, and embryo (tubal, uterine, cervical, and peritoneal factors)
- 3.
Abnormalities in the implantation process, including early defects in embryo development and embryo-endometrial interaction (embryo-endometrial factors)
- 4.
Abnormalities of sperm production (male factor)
- 5.
Other conditions, including immunological factors that can affect multiple components of the process

The initial infertility evaluation focuses on these five major processes ( Box 22.1 ). Abnormalities of embryo-endometrial interaction are reviewed in Chapter 9 . Male infertility is reviewed in Chapter 23 .
Primary Tests for Infertility
Documentation of competent ovulation
Midluteal progesterone >3 ng/mL
Day 3 follicle-stimulating hormone or clomiphene challenge test (if female partner >35 years old)
Semen analysis
Volume ≥1.5 mL
Concentration ≥15 million/mL
Motility ≥32%
Morphology ≥4% (using “strict” criteria)
Terminology used to describe abnormal semen analysis: low sperm concentration, oligospermia; low sperm motility, asthenospermia; sperm morphology abnormal, teratospermia; elevated white blood cells, leukocytospermia
Documentation of tubal patency
Hysterosalpingogram or hysterosalpingo-contrast sonography
Assessment of the uterine cavity
Hysterosalpingogram, hysterosalpingo-contrast sonography, or hysteroscopy
Secondary Tests for Infertility
Laparoscopy
Hysteroscopy
Initial Infertility Evaluation
- ◆
Three tests should be completed early in the infertility evaluation: semen analysis, documentation of ovulation, and a test of tubal patency.
The standard components of the infertility evaluation include a thorough history and physical examination ( Table 22.3 ), a semen analysis (see Box 22.1 ), documentation of competent ovulation, documentation of female reproductive tract and tubal patency, and assessment of the uterine cavity. The evaluation of the semen analysis is discussed in Chapter 23 , Male Infertility. As discussed in more detail later, ovulation may be presumptively detected based on a history of regular menses every 28 days or observation of a luteinizing hormone (LH) surge in the urine using an immunochemical method, and definitively diagnosed by a serum progesterone greater than 3 ng/mL or the histological demonstration of secretory changes on an endometrial biopsy. For women ≥35 years of age, a test of the size of the ovarian follicle pool, also known as ovarian reserve, is warranted. Tests to assess the ovarian follicle pool include measurement of (1) anti-müllerian hormone (AMH), (2) follicle-stimulating hormone (FSH) and estradiol on menstrual cycle day 3, (3) ovarian antral follicle count (AFC) by ultrasound, and (4) FSH and estradiol on cycle days 3 and 10 of a clomiphene citrate challenge test (CCCT).
History | Physical Exam Findings |
---|---|
|
|
Documentation of tubal patency should be accomplished early in the infertility evaluation by a hysterosalpingogram (HSG), a hysterosalpingo-contrast sonogram (HyCoSy), or a laparoscopy. Detection of uterine abnormalities can be accomplished by an HSG, HyCoSy, or hysteroscopy. An HSG has high sensitivity and few false positives for the detection of distal tubal disease, but it is associated with an approximately 15% false-positive rate for diagnosis of proximal tubal occlusion. This means that if the HSG demonstrates that there is proximal tubal blockage, the finding should be confirmed by a second test (selective interventional radiology catheterization of each tube, hysteroscopic cannulation, or laparoscopy with tubal lavage). The HSG also provides evidence of the shape of the uterine cavity and will identify large intrauterine defects. As noted later (anatomical factors in the female), the HyCoSy is gaining in popularity as an initial test in the fertility work-up because it can detect tubal occlusion and is very sensitive for identifying small defects in the uterine cavity. For the detection of uterine abnormalities, hysteroscopy remains the gold standard.
Infertility tests that are probably not needed as part of the initial infertility evaluation include the postcoital test, an endometrial biopsy to detect luteal phase dysfunction, the hamster egg human sperm penetration test, a routine Mycoplasma culture, and antisperm antibody testing. A major problem with the postcoital test is that it has low reproducibility, low interobserver reliability, and has not been reliably shown to help guide treatment recommendations. In addition, there is little consensus on what constitutes an abnormal postcoital test. Given these limitations, there is little scientific rationale for performing a postcoital test.
The endometrial biopsy is abnormal in many infertile women, and in the past, clinicians believed that it was the gold standard for documenting ovulation and assessing endometrial competence for implantation. However, studies have demonstrated that the rate of abnormal out-of-phase endometrial histology is similar in fertile and infertile women. Given the weak correlation between abnormal (out-of-phase) biopsies and fertility, most clinicians are not performing endometrial biopsy as a first-line fertility diagnostic test.
Prior to attempting pregnancy, women should complete preconception testing, including measurement of antibody titers against the rubella, varicella, hepatitis B, and human immunodeficiency (HIV) viruses, a complete blood count to assess for hemoglobinopathies, and appropriate genetic testing. Women considering pregnancy should start a folic acid supplement to reduce the risk of birth defects, including spina bifida, and receive an influenza vaccination.
Abnormalities in Oocyte Production
Disorders of oocyte production are a common cause of female infertility. The most common disorders of oocyte production are anovulation, oligoovulation, depletion of the follicle pool, and aging of the ovarian follicle resulting in poor oocyte quality. Depletion of the follicle pool and aging of ovarian follicles is associated with poor oocyte function. The term diminished ovarian reserve is often used to describe this situation. Anovulation is typically associated with amenorrhea or severe oligomenorrhea. Oligoovulation is typically associated with oligomenorrhea (cycle lengths >35 days). A depleted ovarian follicle pool is usually detected by the measurement of AMH, FSH, and estradiol on day 3 of the menstrual cycle, ovarian AFC by ultrasound, and/or measurement of FSH and estradiol during a CCCT. Oocyte quality is difficult to assess. Poor oocyte quality should be suspected when the age of the female partner is older than 40 years, even if tests of the ovarian follicle pool indicate good ovarian reserve.
Women who have monthly menses and report moliminal symptoms—such as breast tenderness and dysmenorrhea—are typically ovulatory. In low-resource settings, the basal body temperature (BBT) measurement can be used to identify ovulation. For most women, the morning basal temperature obtained prior to rising from bed is less than 98°F before ovulation and over 98°F after ovulation. Progesterone production from the ovary appears to raise the hypothalamic set point for basal temperature by approximately 0.6°F. The temperature rise occurs 1 or 2 days following ovulation. The normal luteal phase is typically associated with a temperature rise, above 98°F, for at least 10 days in length. Occasionally BBT recordings may appear monophasic even in the presence of ovulation. A biphasic pattern is almost always associated with ovulation. If the pattern is biphasic, coitus can be recommended every other day for a period including the 5 days prior to and the day of ovulation ( Fig. 22.3 ). In most developed countries, sequential daily home measurement of urine LH in the periovulatory interval is the most common approach to detecting impending ovulation. A surge in urine LH is detected 1 or 2 days before ovulation.

A serum progesterone level greater than 3 ng/mL is diagnostic of ovulation. In the midluteal and late-luteal phase, progesterone secretion is pulsatile due to the pulsatile nature of luteinizing (LH) secretion. At a conceptual level, the pulsatile nature of progesterone secretion may make it difficult to reliably use a single progesterone measurement as a marker for the adequacy of ovulation. However, in most clinical situations, a single midluteal progesterone measurement appears to be a useful marker of the adequacy of ovulation. Hull et al. have suggested that a midluteal progesterone concentration less than 10 ng/mL is associated with a lower per-cycle pregnancy rate than progesterone levels above 10 ng/mL.
An endometrial biopsy with histological secretory changes is a definitive test demonstrating ovulation (see Chapter 9 ). Although not currently used in the infertility evaluation, it is likely that in the future the endometrial biopsy will be used to detect endometrial proteins that serve as useful markers of endometrial receptivity for implantation. Validation of the detection of implantation markers in endometrial biopsy specimens awaits future research.
Sonographic examination of the ovary and serial measurement of urine LH or estrone-3-glucuronide can be used to demonstrate the growth of a dominant follicle, which is a necessary precondition to ovulation. During menses, the follicles in the ovary are approximately 4 to 9 mm in diameter. Prior to ovulation, the dominant follicle reaches a diameter in the range of 20 to 25 mm. Demonstration of follicle growth and rupture of the dominant follicle is presumptive evidence that ovulation has occurred. Ovulation typically occurs about 36 to 44 hours after the onset of the urine LH surge, and approximately 24 hours after the urine LH peak. In one large prospective study, the detection of a urine LH surge by patients using a home detection kit was associated with ovulation as demonstrated by a secretory endometrial biopsy in 93% of cycles.
Many diseases can cause anovulatory infertility. The most common causes of adult-onset anovulation are hypothalamic dysfunction (35% of cases), pituitary disease (15%), and ovarian dysfunction (50%). The most common causes of hypothalamic dysfunction are abnormalities in weight and body composition, stress, and strenuous exercise (see Chapters 18 and 19 ). Less common causes of hypothalamic dysfunction are infiltrating diseases of the hypothalamus, such as lymphoma and histiocytosis. The pituitary disorders that cause anovulation (see Chapters 3 and 20 ) are prolactinoma, empty sella syndrome, Sheehan syndrome, Cushing disease, acromegaly, and other pituitary tumors. The most common ovarian causes of anovulation are ovarian failure (depletion of the oocyte pool) and ovarian hyperandrogenism (e.g., polycystic ovary syndrome [PCOS]). Occasionally, thyroid disease can be associated with anovulation.
Evaluation of the various causes of anovulation can be complex. Typically, measurement of body weight and height, and measurement of serum FSH, prolactin, thyroid-stimulating hormone (TSH), and androgens, if indicated, can help identify the cause of the anovulation. A progestin withdrawal test may be helpful to evaluate the degree of hypogonadism present, and may help guide treatment choices.
Patients with anovulation have the greatest success with infertility therapy (see Chapter 30 ). Treatment of anovulatory disorders can result in fecundability similar to that observed in normal couples (0.15 to 0.25). The choice of treatment is dependent upon the cause of the anovulation. Common treatment choices include:
- 1.
Interventions to modulate weight
- 2.
Letrozole
- 3.
Clomiphene citrate (CC)
- 4.
Clomiphene plus other hormone adjuvants
- 5.
Gonadotropin treatment (see Chapter 30 )
- 6.
Ovarian surgery
- 7.
Pulsatile administration of GnRH
- 8.
Bromocriptine and cabergoline
Interventions to Modulate Weight and Induce Ovulation
- ◆
Many obese anovulatory infertile women can achieve pregnancy by lifestyle changes including calorie restriction and moderate exercise. Many excessively lean anovulatory infertile women can achieve pregnancy by gaining weight, especially by increasing body fat.
Anovulation, oligoovulation, and subfertility are commonly observed in women above or below their ideal body weight (see also Chapter 18 ). In one study of 597 cases of women with anovulatory infertility and 1695 fertile controls, overweight women (body mass index [BMI] >27 kg/m 2 ) had a relative risk (RR) of anovulatory infertility of 3.1 compared with women of BMI 20 to 25 kg/m 2 . Excessively thin women with a BMI less than 17 kg/m 2 had an RR of anovulatory infertility of 1.6. The investigators concluded that the risk of ovulatory infertility is highest in overweight women, but is also increased in underweight women. For women who are far below or far above their ideal body weight, appropriate management of dietary intake may be associated with resumption of ovulation and pregnancy. For example, Pasquali et al. demonstrated that anovulation in obese women with PCOS could be successfully treated with weight loss. Obese women with anovulation and PCOS were placed on a 1000- to 1500-calorie diet for 6 months. The mean weight loss was 10 kg. After weight loss there was a 45% decrease in basal LH concentration and a 35% decrease in serum testosterone. Many women in this study became pregnant. Most studies of the impact of weight loss on reproductive function did not include a control group. Guzick et al. reported the results of a randomized, controlled trial of the impact of weight loss on reproductive function. Twelve obese, hyperandrogenic, oligoovulatory women were randomized to either a weight reduction program or a “waiting list” observation control group. The six women randomized to the weight reduction program had a mean decrease in weight of 16 kg, a significant decrease in circulating testosterone, a decrease in fasting insulin, and no change in LH pulse frequency and amplitude. In the women who were randomized to the weight reduction program, four of six resumed ovulation. All of the women in the control group who were anovulatory before the study remained anovulatory during the study.
Elevated BMI and sedentary lifestyle decrease fecundability (see Chapter 18 ). Weight loss and a modest increase activity can increase fertility potential. In one study 574 infertile women with a BMI of 29 kg/m 2 or more (median BMI of 36 kg/m 2 ) were randomized to receive a 6-month lifestyle intervention or to a control group. The lifestyle intervention included a reduction in calorie intake by 600 kcal daily with the goal of reducing body weight by ≥5% and increased activity including 10,000 steps daily plus 30 minutes of moderate exercise 2 to 3 times weekly. Natural conception was achieved by 26% of the women in the lifestyle intervention and 16% of the women in the control group (RR 1.61; 95% confidence interval [CI], 1.16 to 2.24). Weight loss prior to ovulation induction may result in a greater live birth rate in women with PCOS. Overweight and obesity are associated with an increased risk of spontaneous abortion and fetal loss, making normalization of weight an important prepregnancy goal.
Weight loss is difficult to achieve. Consultation with a nutritionist, encouragement by a physician, a hypocaloric diet, and initiation of an exercise program may be the most effective nonsurgical interventions that can help a woman lose weight. Surgical methods of weight reduction can be very effective, especially in women with a BMI over 40 kg/m 2 .
Excessively lean women are at increased risk for anovulatory infertility. In experimental models, oligoovulation ensues following interventions that result in daily energy expenditure greater than daily calorie intake. In the captive female monkey, regular ovulatory cycles are observed with routine activity and a steady calorie intake at 300 kcal daily. When calorie intake is maintained at 300 kcal daily, but activity is increased to include 6 miles of additional ambulation daily, anovulation and amenorrhea ensue. Increasing calorie intake to up to 600 kcal daily while maintaining 6 miles of exercise daily results in resumption of ovulation and menses. In the exercising monkey, resumption of ovulation also can be initiated without an increase in calorie intake by administering pulses of GnRH. In a study of sedentary women assigned to exercise plus calorie restriction or exercise plus a eucaloric diet, the exercise plus calorie restriction group produced greater declines in ovarian steroid production than exercise plus a eucaloric diet.
Hormones that help the brain assess the relative levels of calorie intake and energy expenditure include leptin, insulin, thyroid hormones (thyroxine and triiodothyronine), growth hormone, insulin-like growth factor 1 (IGF1), cholecystokinin, glucagon-like peptide-1, and ghrelin. Women with hypothalamic amenorrhea (hypothalamic hypogonadism) often have low levels of leptin. Two clinical trials reported that the administration of exogenous leptin or a leptin decapeptide (metreleptin) to lean women with hypothalamic amenorrhea resulted in the resumption of ovulatory menses in some of the subjects. In addition, metreleptin administration for 36 weeks increased free triiodothyronine, IGF1, and osteocalcin. Leptin may exert its effect on GnRH secretion by a direct action on kisspeptin-releasing neurons.
Lean women with anovulatory infertility are often reluctant to gain weight, alter their diet, or reduce their exercise regimen. However, in one study of 26 underweight women who practiced strict dieting and were infertile, the subjects were counseled by a dietician and given physician-directed advice to increase their BMI. After the intervention, the women gained a mean of 3.7 kg and 73% of the women became pregnant. Interpersonal psychodynamic psychotherapy or cognitive behavior therapy may help lean anovulatory women to resume ovulation. It is important to try to achieve a normal BMI prior to initiating ovulation induction in excessively lean anovulatory women because in a pregnant woman with a low BMI there is an increased risk of delivering an infant with low birth weight, small head circumference, and microcephaly.
Specific dietary factors may influence the risk of anovulatory infertility. For example, in one prospective study, women who consumed iron supplements were reported to have a 40% lower risk of anovulatory infertility. In another prospective study, dietary patterns characterized by high consumption of monounsaturated rather than trans fats, vegetable rather than animal protein, low glycemic carbohydrates, high fat dairy, and multivitamins were associated with a reduced risk of ovulatory infertility. For lean or normal-weight anovulatory infertile women, one practical recommendation for increasing high fat dairy intake is to eat ice cream and put butter on their vegetables. Myo-inositol is a dietary supplement that is a precursor of D-chiro-inositol, which is involved in the control of glucose metabolism and cell response to insulin stimulation. In one small observational study without a control group, oligoovulatory women with PCOS who took myo-inositol, 2 g twice daily, reported an increase in spontaneous menstrual cycles. Forty percent of the subjects became pregnant during 6 months of myo-inositol treatment.
Letrozole
- ◆
For the treatment of anovulatory infertility caused by PCOS, letrozole is gradually replacing clomiphene as the first-line treatment.
Aromatase inhibitors, including letrozole and anastrozole, block estradiol synthesis, reduce estradiol feedback on the hypothalamus-pituitary, and increase production of FSH in women with PCOS. Letrozole at doses of 2.5 to 7.5 mg daily for 5 days, and anastrozole at a dose of 1 mg daily for 5 days have been demonstrated to induce ovulation in women with PCOS. In addition, clinical trials have reported that letrozole is superior to clomiphene for ovulation induction, but anastrazole is NOT superior to clomiphene. These data suggest that letrozole may be a first-line treatment for anovulatory infertility due to PCOS, but anastrozole should generally not be used for this indication. The US Food and Drug Administration (FDA) has approved letrozole for the treatment of breast cancer in postmenopausal women, but it is not approved for ovulation induction. Infertile women using letrozole for ovulation induction should be aware of the off-label use of letrozole.
Legro et al. randomized 750 women with anovulatory infertility and PCOS to clomiphene or letrozole for ovulation induction. The medications were used in a step-wise dose escalation protocol. The doses of clomiphene were 50, 100, and 150 mg/day. The doses of letrozole were 2.5, 5, and 7.5 mg/day. The lowest dose that induced ovulation as determined by the measurement of progesterone was used in up to 5 cycles. The medications were given for 5 days on cycle days 3 to 7 following a spontaneous menses or a medroxyprogesterone acetate withdrawal bleed. The ovulation rates for letrozole and clomiphene were 62% and 48%, respectively ( P < .001). The live birth rates for letrozole and clomiphene were 28% and 19%, respectively ( P < .007). Among women with a BMI of ≤30.3 kg/m 2 , both letrozole and clomiphene resulted in a similar live birth rate, 35% and 30%, respectively. Among women with a BMI ≥30.3 kg/m 2 , the live birth rates with letrozole and clomiphene were 20% and 10%, respectively. For letrozole and clomiphene, the spontaneous abortion rate (32% and 29%) and the twinning rate (3.4% and 7.4%) were not statistically different. These results demonstrate the critical importance of achieving a BMI less than 30 kg/m 2 prior to ovulation induction. This study also indicates that letrozole is superior to clomiphene for ovulation induction in PCOS, especially in women with a BMI ≥30.3 kg/m 2 .
In contrast to letrozole, anastrozole is not superior to clomiphene. In clinical trials, anastrozole at doses of 1, 5, and 10 mg daily for 5 days was less effective for ovulation induction in the first cycle of treatment than clomiphene at a dose of 50 mg.
Letrozole and anastrozole are not approved by the FDA for ovulation induction. Pregnancy and birth registries indicate that pregnancy outcome following ovulation induction with aromatase inhibitors is good. In one registry, the risk of congenital cardiac malformations was greater with clomiphene-induced pregnancy than with letrozole-induced pregnancy. However, concern remains about the potential adverse effects of these agents on pregnancy, especially given the known adverse effects of aromatase inhibitors on rabbit and rodent pregnancies.
Ovulation induction and ovarian stimulation in women with estrogen-sensitive tumors, such as breast cancer, may be a special situation where the use of aromatase inhibitors should be prioritized. Aromatase inhibitors increase FSH levels, but block estradiol production, resulting in folliculogenesis with relatively reduced levels of circulating estradiol compared with clomiphene or gonadotropin treatment. In women with a history of estrogen-sensitive tumors, inducing folliculogenesis and ovulation while maintaining relatively low levels of circulating estradiol has a theoretical advantage. This effect may be especially advantageous in women with a history of breast cancer planning on undergoing an IVF cycle.
Aromatase inhibitors may be an effective monotherapy option for women who are clomiphene resistant. In one trial, 250 anovulatory women with PCOS who did not ovulate with standard doses of clomiphene were randomized to treatment with letrozole 2.5 mg daily for 5 days or metformin 1500 mg plus clomiphene 150 mg daily for 5 days. The ovulation rate was 65% in the letrozole group and 70% in the metformin-clomiphene group. The pregnancy rate was 15% and 14% in the letrozole and metformin-clomiphene groups, respectively. Given the superiority of letrozole compared to clomiphene or anastrozole, first-line therapy with letrozole is recognized as a reasonable option, even though it is not FDA approved for this purpose.
Clomiphene
- ◆
Many anovulatory women with PCOS do not become pregnant on clomiphene, and the use of alternative agents such as letrozole, or adjuvants such as glucocorticoids or metformin, should be considered if 3 cycles of clomiphene with escalating doses does not result in ovulation.
Clomiphene, a nonsteroidal triphenylethylene derivative estrogen agonist-antagonist related to tamoxifen and diethylstilbestrol, was first synthesized in 1956. In 1961, Greenblatt et al. reported clomiphene to be effective in the induction of ovulation, and the drug was approved by the FDA in 1967 (see also Chapter 30 ). CC is marketed as a racemic mixture of enclomiphene (E, trans) and zuclomiphene (Z, cis) in a ratio of approximately 3 to 2. The Z-isomer may have greater ovulation-inducing properties than the trans isomer. An important recent observation is that nonsteroidal triphenylethylene compounds like tamoxifen and clomiphene may require bioactivation by the liver cytochrome P450 enzyme 2D6. In one study, liver microsomes containing CYP2D6 , metabolized clomiphene to two potent estrogen antagonist compounds, (E) -4-hydroxyclomiphene and (E) -4-hydroxy- N -desethylclomiphene. These two compounds demonstrated 50% inhibition of estrogen receptor function at concentrations of 2.5 and 1.4 nM, respectively. The activity of CYP2D6 shows significant variation among women, suggesting that allelic variation in this enzyme may contribute to the variability in the response to clomiphene.
Clomiphene has a half-life of approximately 5 days. It is metabolized by the liver and excreted in the feces. Fecal clomiphene can be detected up to 6 weeks after discontinuing the drug. In normally cycling women , the administration of CC, 150 mg daily for 3 days, resulted in an increase in the serum concentration of LH and FSH of 40% and 50%, respectively. In addition, LH pulse frequency increased from 3.3 to 6.8 pulses per 8 hours. The clomiphene-induced increase in LH pulse frequency indicates that clomiphene has an action at the hypothalamus. In women with PCOS who already have a high LH pulse frequency, clomiphene does not further increase LH pulse frequency, but it does increase LH pulse amplitude and serum levels of LH and FSH. Successful induction of ovulation with clomiphene requires an intact hypothalamic-pituitary-ovarian axis. In contrast, exogenous gonadotropin treatment is effective even in the absence of a functional hypothalamus or pituitary.
Evidence that clomiphene has central nervous system effects includes the observation that clomiphene induces vasomotor symptoms, increases LH pulse frequency, and partially blocks the contraceptive potency of estrogen. Studies in laboratory animals demonstrate that clomiphene can decrease estrogen-stimulated hypothalamic tyrosine hydroxylase, and that clomiphene increases GnRH secretion from the rat medial basal hypothalamus.
In addition to a hypothalamic site of action, clomiphene also has biologic effects on the pituitary, ovary, endometrium, and cervix. In incubations of rat pituitary cells, both estradiol and clomiphene augmented GnRH-induced release of FSH and LH. Zhuang et al. demonstrated that clomiphene, estradiol, and diethylstilbestrol all augmented gonadotropin induction of aromatase activity in rat granulosa cells. In hypoestrogenic women receiving exogenous estrogen, clomiphene can cause endometrial atrophy. Clomiphene can diminish estrogen-induced cervical mucus quantity and quality, as demonstrated by decreased ferning and spinnbarkeit formation. Although clomiphene may induce ovulation, the adverse effects of on the endometrium and cervix may reduce the pregnancy rate per ovulatory cycle.
Clomiphene is most effective in inducing ovulation in women with euestrogenic anovulation, including women with PCOS. In women with severe hypoestrogenism and hypogonadotropic hypogonadism, clomiphene is typically ineffective in the induction of ovulation. In contrast, women with hypogonadotropic hypogonadism respond well to gonadotropin injections or pulsatile GnRH treatment. Failure to have a withdrawal uterine bleed following the administration of progesterone is presumptive evidence of severe hypoestrogenism in women with anovulation and an anatomically normal uterus. Clomiphene is unlikely to effectively induce ovulation in this setting. Maruo et al. have reported that clomiphene induction of ovulation has a low chance of success in women with triiodothyronine levels below 80 ng/mL, levels sometimes seen in hypothyroidism, malnutrition, or with eating disorders. CC is also unlikely to be effective in women with a consistently elevated FSH concentration (depletion of oocyte pool). Although clomiphene is relatively contraindicated in women with pituitary tumors, it has been reported to be effective in the induction of ovulation in women with a prolactinoma who did not ovulate with bromocriptine treatment alone.
The FDA-approved dosages for clomiphene are 50 or 100 mg daily for a maximum of 5 days/cycle. After spontaneous menses, or the induction of menses with a progestin withdrawal, clomiphene is started on cycle day 3, 4, or 5 at 50 mg daily for 5 days. Starting clomiphene on cycle day 3 or 5 does not appear to influence the per-cycle pregnancy rate. In properly chosen women, approximately 50% will ovulate at the 50 mg daily dosage; another 25% will ovulate if the dose is increased to 100 mg daily. During each cycle, determination of ovulation should be attempted. In most patients, ovulation occurs approximately 5 to 12 days after the last dose of clomiphene. Measurement of the urinary LH surge is recommended to assist the couple in prospectively determining the preovulatory interval, which is the optimal time for achieving pregnancy.
Although the FDA has approved maximal clomiphene doses of 100 mg daily, a woman who does not ovulate with a clomiphene dose of 100 mg daily for 5 days may ovulate if her dose is increased to 150 mg daily for 5 days. Of the women who do not ovulate at doses of 100 mg daily, up to 70% will ovulate at higher doses, but less than 30% become pregnant.
Anovulatory women have a fecundability of 0.00 without treatment. Over the first 3 to 6 cycles of clomiphene treatment, the fecundability is in the range of 0.08 to 0.25. In cases where the only fertility factor is anovulation in the female partner, fecundability with clomiphene treatment is in the range of 0.15 to 0.25 ( Fig. 22.4 ). Clomiphene is one of the few fertility treatments that cost less than $100 and can result in a significant increase in fecundability. Clomiphene is less likely to induce ovulation in women with hyperandrogenemia, markedly elevated BMI, amenorrhea, or advanced age. After 3 to 6 months of clomiphene treatment, fecundability appears to decline. Following 3 clomiphene cycles with documented ovulation and no pregnancy, experts recommend that a new approach to treatment be considered rather than continuing with clomiphene therapy.

Prior to initiating a clomiphene cycle, many experts obtain a pregnancy test to rule out an ongoing pregnancy, then prescribe a progestin withdrawal. A commonly used progestin withdrawal is medroxyprogesterone acetate (Provera) 10 mg daily for 5 days. The first day of full withdrawal flow following the cessation of the progestin treatment is day 1 of the cycle.
During the clomiphene treatment cycle, urine LH measurements can be measured by the patient at home to identify the preovulatory LH surge. The LH surge typically occurs 5 to 12 days after the last day of clomiphene medication. The woman’s maximal fertile time is the day before the urine LH surge, the day of the urine LH surge, and the day following the urine LH surge. Coitus should occur on at least 2 of these 3 days. Alternatively, if the woman prefers not to measure urine LH she can have coitus with her partner every other day for 8 days beginning 5 days after the last clomiphene tablet. Evidence for successful clomiphene-induced ovulation is an appropriately drawn serum progesterone level greater than 8 ng/mL. In most clomiphene cycles resulting in successful ovulation and pregnancy, the serum progesterone level is greater than 20 ng/mL. If a menses does not occur within 17 days following the LH surge, a pregnancy test can be obtained.
Some epidemiologic studies reported that clomiphene may increase the risk of ovarian tumors, including borderline tumors and ovarian cancer. However, most recent studies have not detected an increase in ovarian borderline tumors or ovarian cancer in women exposed to clomiphene.
The role of hCG administration in enhancing the pregnancy rate associated with clomiphene treatment is controversial. Some authorities believe that the combination of clomiphene and a single dose of hCG may increase the efficacy of clomiphene induction of ovulation when women do not ovulate on standard doses of clomiphene. However, in most trials, hCG administration does not consistently improve pregnancy rate compared to women who use urinary LH testing to detect the preovulatory window.
In one study of 2369 clomiphene-induced pregnancies, 7% were twins, 0.5% were triplets, 0.3% were quadruplets, and 0.13% were quintuplets. The most common symptoms experienced by women taking clomiphene include: vasomotor symptoms (20%), adnexal tenderness (5%), nausea (3%), headache (1%), and, rarely, blurring of vision or scotomata. Most clinicians permanently discontinue clomiphene treatment in women with clomiphene-induced visual changes.
Clomiphene Plus Glucocorticoid Induction of Ovulation
Women with PCOS who have failed to ovulate using standard doses of clomiphene are referred to as being clomiphene resistant. A consensus panel of expert fertility specialists recommended that for women with PCOS who are clomiphene resistant, the most appropriate next steps in treatment is gonadotropin injections or laparoscopic ovarian drilling. However, for many women these options may be prohibitively expensive. For the clomiphene-resistant woman with PCOS, what treatment can be prescribed that is affordable?
Many clomiphene-resistant women will ovulate if they are treated with a combination of both clomiphene and dexamethasone. Before initiating combination therapy with clomiphene plus dexamethasone, the results of the infertility work-up should be reviewed to be sure that tubal and male factors are not contributing to the fertility problem. Two randomized, clinical trials have reported that, in clomiphene-resistant women, dexamethasone plus clomiphene treatment results in an increase in ovulation and pregnancy rates compared to clomiphene alone. One regimen that has been reported to be successful is to treat the clomiphene-resistant woman with clomiphene 100 mg daily for cycle days 3 to 7, and simultaneously treat her with dexamethasone 2 mg daily for cycle days 3 to 12 ( Fig. 22.5 ). Treatment with dexamethasone reduces the serum concentration of androgens, thereby increasing the efficacy of the clomiphene. In the randomized trial that used this regimen to treat clomiphene resistant women, the ovulation rate was 75% in the clomiphene plus dexamethasone group and 15% in the clomiphene alone group ( P < .001). The pregnancy rate was 40% in the clomiphene plus dexamethasone group and 5% in the clomiphene alone group ( P < .05). Other investigators have also reported that a glucocorticoid is an effective adjuvant to clomiphene treatment for certain women.

Many clinicians instruct their patients to take the dexamethasone at night to maximally blunt the early morning corticotropin adrenocorticotropic hormone (ACTH) surge, which stimulates adrenal androgen production. However, experienced clinicians have found that for many women a nighttime dose of dexamethasone energizes them and causes difficulty in falling and remaining asleep. Some experts recommend that patients take the dexamethasone in the morning. If the combination of clomiphene (100 mg daily for 5 days) plus dexamethasone does not cause ovulation, a cycle with a clomiphene dose of 150 mg daily for cycle days 3 to 7 plus dexamethasone can be prescribed. If this regimen does not cause ovulation, the patient should consider other options for ovulation induction, such as weight loss, gonadotropin injections, laparoscopic ovarian drilling, or IVF.
Clomiphene and Estrogen-Progestin Contraceptive Pretreatment
A risk factor for failure to ovulate with clomiphene is a baseline elevated circulating testosterone level. Estrogen-progestin pretreatment prior to a cycle of clomiphene may improve ovulation rates by suppressing circulating testosterone prior to the initiation of a clomiphene cycle. In one small case series and one randomized trial, 2 months of continuous estrogen-progestin contraceptive pill prior to treatment with clomiphene was reported to decrease circulating testosterone and improve ovulation and pregnancy rates in women with PCOS who had failed to ovulate with clomiphene 150 mg daily for 5 days. In the randomized trial, 48 women who had failed to ovulate with clomiphene 150 mg daily for 5 days were randomized to 42 to 50 days of pretreatment with an estrogen-progestin contraceptive (ethinylestradiol 0.03 mg plus desogestrel 0.15 mg daily with no break) followed by clomiphene 100 mg daily for 5 days, or clomiphene alone. The oral contraceptive regimen (OCP) significantly reduced circulating testosterone prior to the initiation of clomiphene. The ovulation rate was 65% and 11% in the OCP-CC group versus the CC group, respectively. Per-cycle pregnancy rates were 54% and 4%, respectively. A regimen of OCPs followed by clomiphene may be clinically indicated in women who have failed to ovulate with clomiphene or in women known to have an elevated total testosterone. A large-scale randomized trial of this sequential regimen is warranted.
Clomiphene and Nonclassical Adrenal Hyperplasia
Many authorities recommend that infertile anovulatory women with nonclassical adrenal hyperplasia (NCAH) due to mutations in 21-hydroxylase receive glucocorticoids, such as prednisone 5 to 7.5 mg daily, for induction of ovulation. However, some women with long-standing NCAH also have evidence of ovarian hyperandrogenism and polycystic ovarian morphology on ultrasound. Clomiphene alone or clomiphene plus glucocorticoids can be used to induce ovulation and achieve pregnancy in infertile women with NCAH.
Clomiphene Plus Gonadotropin Induction of Ovulation
In women who do not ovulate with standard doses of CC, gonadotropin injections can be added to clomiphene treatment to induce ovulation. The main benefit of this approach to ovulation induction is that it tends to reduce the quantity of gonadotropins needed to induce ovulation during each cycle. The initial rise in LH and FSH induced by clomiphene increases the sensitivity of the follicles to respond to the gonadotropin injections. Typically, clomiphene at doses of 100 to 200 mg daily is administered for 5 days, followed by the initiation of FSH or LH-FSH injections. Investigators have reported that this regimen is associated with a 50% decrease in the dose of gonadotropin required to induce ovulation.
Clomiphene and Metformin
Hyperinsulinemia is a common endocrine abnormality observed in women with PCOS (see Chapter 21 ). The elevated insulin levels contribute to reproductive dysfunction by suppressing hepatic sex-hormone-binding globulin production and possibly by acting as a co-gonadotropin with LH resulting in the stimulation of thecal cell androgen synthesis. Thus, reducing insulin levels is a therapeutic goal in women with PCOS.
Metformin is an oral biguanide antihyperglycemic agent approved for the treatment of type 2 diabetes mellitus. Metformin decreases blood glucose by inhibiting hepatic glucose production and by enhancing peripheral glucose uptake, possibly by interacting with the Peutz-Jegher syndrome tumor suppressor gene (LKB1) , which activates adenosine-monophosphate-activated protein kinase. It increases insulin sensitivity at the postreceptor level and stimulates insulin-mediated glucose disposal. Generic extended release metformin is available in doses of 500, 750, and 1000 mg tablets. The target metformin dose is in the range of 1500 to 2550 mg daily. When using metformin extended-release tablets, the entire daily dose is given at dinner time. To minimize gastrointestinal adverse effects like nausea, many clinicians recommend that metformin should be started at 500 or 750 mg daily for 1 week, followed by an increase in the dose to the target range. If metformin is used as monotherapy, progesterone levels can be measured periodically at appropriate days to determine if ovulation has occurred, or the patient can keep a BBT record. If ovulation has not occurred after 5 to 10 weeks of metformin monotherapy, then clomiphene, 50 mg daily for 5 days, can be administered in conjunction with metformin. If the patient becomes pregnant, the metformin therapy can be discontinued. Metformin is a category B drug for pregnant women and has been used by some clinicians to treat type 2 diabetes and gestational diabetes in pregnant women.
The most common adverse effects associated with metformin are gastrointestinal disturbances, including diarrhea, nausea, vomiting, and abdominal bloating. In rare cases, metformin treatment has caused fatal lactic acidosis. In most of these cases, some degree of renal insufficiency was present. Although lactic acidosis caused by metformin is rare, the FDA recommends that prior to initiating treatment with metformin, the patient’s serum creatinine concentration should be measured and demonstrated to be less than 1.4 mg/dL. Other insulin sensitizers may also be effective in the induction of ovulation, either alone, or in combination with clomiphene or gonadotropins.
Clinical trials have reported conflicting results concerning the relative efficacy of metformin versus clomiphene. In general, the majority of large-scale clinical trials have reported that both metformin and clomiphene monotherapy are effective at inducing ovulation in women with PCOS, but clomiphene results in a greater per cycle ovulation, conception, and birth rates than metformin. In one study, 626 women with anovulatory infertility caused by PCOS were randomized to receive clomiphene alone, metformin alone, or clomiphene plus metformin. The live birth rate was 27% in the clomiphene-metformin group, 23% in the clomiphene group, and 7% in the metformin monotherapy group. In contrast, other investigators have reported that single-agent treatment with clomiphene or metformin results in similar pregnancy rates. In some studies, metformin appears to be more effective in inducing ovulation in women with an above average waist-to-hip ratio, a marker of increased visceral fat. For a woman with anovulatory infertility due to PCOS who has failed to become pregnant with clomiphene, FSH treatment or ovarian drilling is more likely to result in pregnancy than treatment with clomiphene plus metformin. However, if FSH treatment or ovarian drilling are not available to the patient because of their high cost, adding metformin to clomiphene or switching to letrozole ovulation induction are low-cost options.
Clomiphene versus Tamoxifen or Raloxifene for Ovulation Induction
Clomiphene, tamoxifen, and raloxifene are mixed estrogen agonists-antagonists with varying degrees of agonist or antagonist activity in different end organs. In a randomized trial, 371 with anovulatory infertile women with PCOS were randomized to receive CC 100 mg daily or tamoxifen 20 mg daily for 5 days. The ovulation rate was 64% in the clomiphene group and 52% in the tamoxifen group ( P = .01), and the pregnancy rate was 19% and 11% in the clomiphene and tamoxifen groups, respectively ( P = .04). Clomiphene at a dose of 100 mg daily appears to be superior to tamoxifen at a dose of 20 mg daily for the induction of ovulation in women with PCOS. In a small clinical trial, 5 days of treatment with clomiphene 100 mg daily or raloxifene 100 mg daily resulted in a similar rate of ovulation, but the pregnancy rate was not studied in this trial.
Gonadotropin Induction of Ovulation
- ◆
The Achilles heel of gonadotropin ovulation induction is the high risk of a multiple gestation pregnancy.
The use of gonadotropins, gonadotropins plus GnRH antagonists, and gonadotropins plus growth hormone to treat infertility; and the prevention and treatment ovarian hyperstimulation syndrome (OHSS) are discussed in detail in Chapter 30 . Important concepts related to the use of gonadotropins to treat anovulatory infertility is that women with the best prognosis for success have hypogonadotropic hypogonadism (WHO I) ( Fig. 22.6 ) and are younger than 35 years of age ( Figs. 22.7 and 22.8 ).



Ovarian Surgery for Ovulation Induction in Polycystic Ovary Syndrome
Ovarian wedge resection was one of the first treatments used to induce ovulation in women with PCOS. However, classical ovarian wedge resection was associated with ovarian and tubal adhesions. Laparoscopic ovarian drilling using insulated needle cautery has been reported to be associated with a 70% rate of ovulation and a 50% pregnancy rate. It should be noted that ovarian drilling is a second-line treatment for anovulatory infertility due to PCOS, and first-line treatments including clomiphene and letrozole should always be used before considering ovarian drilling. The procedure can be performed by immobilizing the ovary with a probe and inserting an insulated needle electrode into the ovary. A cutting current of 100 watts can be used to ease the needle into the ovarian cortex. After the needle is inserted into the ovary, a 40-watt coagulating current is applied for 2 seconds at each point of puncture. Each ovary can be treated with 5 to 10 punctures; smaller ovaries should be treated with fewer punctures. In general, one or two punctures are not adequate to ensure ovulation postoperatively. At the completion of the procedure, 1000 mL of crystalloid solution can be left in the pelvis.
In one clinical trial, 88 anovulatory, infertile women with PCOS who had not ovulated with clomiphene were randomized to ovarian surgery, FSH injections, or combination LH-FSH injections. The ovulation rate (70%) and the pregnancy rate (50%) were similar in all three groups. The spontaneous abortion rate was higher in the two groups that received gonadotropins. The investigators concluded that ovarian surgery was as efficacious as gonadotropin injections for ovulation induction in women with PCOS who did not ovulate with clomiphene. In another clinical trial, women with PCOS who failed to ovulate with clomiphene 150 mg daily for 5 days were randomized to gonadotropin injections or laparoscopic ovarian diathermy. Six months after surgery, the cumulative spontaneous pregnancy rate was 28%. After 3 cycles of gonadotropin injections, the cumulative pregnancy rate was 33%.
The risks of ovarian surgery for women with hirsutism are greater than the potential benefits. However, for women with PCOS and infertility who have been unable to become pregnant with weight loss, clomiphene and metformin treatment, the option of ovarian surgery for ovulation induction may be warranted prior to induction of ovulation with FSH. Survey data indicate that many patients prefer one surgical intervention compared to ovulation with FSH injections, if the two treatments have comparable success rates.
Cost-benefit analyses suggest that laparoscopic surgery for ovulation induction is associated with less healthcare costs than FSH injections. Other authorities believe that the rare serious risks associated with surgery makes FSH injections the preferable option.
Pulsatile Administration of Gonadotropin-Releasing Hormone
- ◆
Pulsatile administration of GnRH is not available in the United States but is available in many European countries.
A key feature of hypothalamic biology is the pulsatile release of the decapeptide GnRH from the arcuate nucleus into the pituitary portal circulation. The pulsatile release of GnRH stimulates the pituitary to produce LH and FSH in a pulsatile manner. In turn, pituitary gonadotropin secretion stimulates follicular development, ovulation, and progesterone secretion in the luteal phase. In women with hypogonadotropic hypogonadism and anovulation (low levels of endogenous gonadotropins and decreased endogenous estrogen production), the pulsatile administration of GnRH is effective in inducing ovulation ( Fig. 22.9 ). The advantages of GnRH induction of ovulation include a reduced need for cycle monitoring and a reduced risk of multiple gestation due in part to an intact pituitary feedback system. The main disadvantage of pulsatile GnRH therapy is that the infusion pump and subcutaneous delivery system may be difficult for patients to maintain. Pulsatile GnRH for ovulation induction is currently not available in the United States.

Santoro et al. proposed eight criteria for identifying women most likely to safely achieve ovulation with pulsatile GnRH:
- 1.
Primary or secondary amenorrhea for at least 6 months
- 2.
Absence of hirsutism, galactorrhea, or ovarian enlargement
- 3.
Weight not below 90% of ideal body weight
- 4.
No excessive exercise or stress
- 5.
Normal serum prolactin, TSH, dehydroepiandrosterone sulfate (DHEAS), and testosterone concentrations
- 6.
Low gonadotropin concentrations
- 7.
No evidence of a structural central nervous system lesion
- 8.
No recent hormone treatment
GnRH is administered using a computer-driven pump that delivers one pulse of GnRH every 90 minutes at a dose of 75 to 100 ng/kg per pulse. In Europe, doses of 10 to 30 µg per pulse are commonly used. Interpulse intervals as short as 1 hour or as long as 2 hours have been successfully used. GnRH doses as low as 25 ng/kg per pulse can successfully induce ovulation, but are associated with subnormal luteal phase progesterone secretion. Both intravenous and subcutaneous administration of GnRH have been successfully used to induce ovulation. Intravenous administration probably results in a more reliable induction of ovulation, but this route is associated with more technical problems (restarting the intravenous catheter) and risk of infection than subcutaneous administration. The intensity of clinical monitoring can range from regular follicle monitoring with sonography and serum estradiol measurements to BBT measurement with use of an ovulation predictor kit. Low-intensity monitoring is acceptable because the risk of multiple pregnancy or ovarian hyperstimulation is low with pulsatile GnRH therapy.
Studies that compare the efficacy of gonadotropin versus pulsatile GnRH induction of ovulation report that pulsatile GnRH induction of ovulation may be associated with superior ovulation and pregnancy rates than gonadotropin treatment. The risk of multiple gestation is higher with gonadotropin treatment (14%) than with pulsatile GnRH treatment (8%). This increased risk is due to a higher rate of multifollicular development with gonadotropin treatment (48% of cycles) than with pulsatile GnRH treatment (19% of cycles). Pulsatile GnRH results in a decreased risk of high-order multiple gestation when compared with gonadotropin treatment.
Hyperprolactinemia
Infertile women with hyperprolactinemia and anovulation often achieve pregnancy after treatment with a dopamine agonist, such as bromocriptine or cabergoline. The treatment of hyperprolactinemia is discussed in Chapter 3 .
Luteal Phase Deficiency
Luteal phase deficiency has been defined as a condition in which the ovarian secretion of progesterone is not sufficient to maintain a functional secretory endometrium that supports embryo implantation and growth. The gold standard test for identifying luteal phase deficiency is histologic dating of tissue from an endometrial biopsy that demonstrates a developmental lag of at least 2 days. However, most recent research indicates that delayed maturation of the endometrium is observed at a similar rate in both fertile and infertile women. Most authorities believe that luteal phase deficiency is not a major independent cause of infertility.
From an endocrine perspective, follicular or luteal function that is far outside the normative range is likely associated with reduced fertility. In the most extreme example, it is known that resection of the corpus luteum and the associated reduction in progesterone secretion reduces fertility and causes abortion in women prior to about 49 days of pregnancy. In addition, numerous clinical trials have observed that luteal phase progesterone support improves pregnancy rates following IVF cycles involving controlled ovarian hyperstimulation and ovarian follicle puncture to harvest oocytes. It is probable that an occasional case of infertility may be caused by poor follicle development and inadequate corpus luteal secretion of progesterone or a relative resistance to progesterone effect in the endometrium. However, it is unlikely that luteal phase deficiency is a common cause of infertility.
Aging Ovary, Aging Follicle
- ◆
Current approaches to fertility treatment cannot reverse the detrimental effect of ovarian aging on fecundability.
An immutable feature of ovarian physiology is that the number of oocytes and follicles are fixed in utero and decline following an exponential curve (mathematically similar to the curve for the decay of radioactive material) from the second trimester, as shown in Fig. 22.10 (see also Chapters 8 and 14 ). At birth, an estimate of the number of oocytes and follicles in a pair of human ovaries is approximately 2 million. At the completion of puberty, the number of oocytes in a pair of human ovaries is in the range of 250,000. After 35 to 37 years of age, the rate of loss of oocytes and follicles appears to accelerate. During adult reproductive life, follicles most sensitive to the growth-promoting effects of FSH are first selected to become the dominant follicle. As the ovary and the residual follicular pool age, the remaining follicles appear to be relatively resistant to FSH. The aging follicle contains an oocyte that is less likely to result in a successful pregnancy. The decrease in fecundability associated with aging is probably due to a decline in both the quantity and quality of follicles and oocytes. The decrease in quality is likely associated with dysfunction in intracellular mechanisms necessary for oocyte meiosis, fertilization, and embryo growth. Data to support the concept that the aging oocyte is less likely to result in a successful pregnancy come from multiple sources (see Figs. 22.7 and 22.8 ).

Most mammalian species do not have histologically detectable mitotic oogonia or germ stem cells in the adult ovary. However, recent studies in rodents and humans have reported that there are stem cells in the adult ovary that can be harvested, stimulated to undergo mitotic replication, and then be triggered to form competent oocytes. The generation of functional oocytes from primordial germ cells has been best documented in the mouse model. In the mouse, using primordial germ cells, functional oocytes have been generated in vitro, and a live birth has occurred following fertilization and intrauterine transfer of the fertilized oocyte. Mitochondrial dysfunction has been hypothesized to be a major cause of age-related decline in oocyte function. In an attempt to rejuvenate oocytes in older women, investigators have explored the role of autologous mitochondrial injection (AMI). In this process, the ovarian cortex is harvested with laparoscopic surgery and purified oogonial precursor cells (OPCs) are prepared from the tissue. Mitochondria are isolated from the OPCs and injected into aging oocytes during an IVF cycle using intracytoplasmic sperm injection. The AMI procedure has been reported to be associated with good oocyte fertilization rates (78%) and some pregnancies. A large randomized trial would be needed to definitively test the effectiveness of this innovative treatment and assess its impact on newborn outcomes, including congenital malformations.
Mathematical descriptions of the decline in ovarian follicles and oocytes favor one of three models: (1) an exponential rate of decline with a fixed “half-life,” similar to models describing the decay of radioactive material; (2) an exponential rate of decline with two distinct rates of decline, a slow rate of decline early in life, and a more rapid rate of decline later in reproductive life; and (3) a power model where the rate of decline accelerates throughout a woman’s life. The rate of decline of AMH, a biochemical marker of ovarian follicle number, and the AFC assessed by sonography also appear to accelerate with female aging, supporting the power model of decline.
The discovery of interventions that could prevent the decline of oocytes and follicles in the adult ovary is an important priority. In the mouse model, caloric restriction or knockout of the peroxisome proliferator-activated receptor γ coactivator-1α, a component of a metabolic regulatory pathway, were reported to delay the rate of oocyte loss.
For infertile couples where the cause of the infertility is azoospermia in the male partner, the success of donor sperm insemination is directly related to the age of the female partner. In a study of 1654 women undergoing donor insemination, the cumulative live birth rate after 12 cycles decreased from 87% for women 20 to 29 years of age to 66% and 52% for women 38 to 39 years and 40 to 45 years of age, respectively. Data from IVF programs demonstrate that the age of the female partner is an important determinant of pregnancy rates. In 2014 for women younger than 35 years of age, the live birth rate per cycle was 49% compared to 20% in women 38 to 40 years of age, and 11% in women 41 to 42 years of age. The poor functional quality of oocytes in the terminal follicle pool is a major cause of the relationship between female aging and diminishing fecundability. The poor functional quality of these oocytes is supported by the observed high rate of aneuploid blastocytes and trisomic pregnancy that occurs following their fertilization, and the poor performance of these oocytes in the process of IVF, including poor fertilization, embryonic growth, low implantation, and pregnancy rates.
Four major measurements are used to assess the size of the ovarian follicle and oocyte pool in infertile women: (1) AMH concentration measured at any time in the menstrual cycle; (2) menstrual cycle day 3 FSH and estradiol concentration; (3) AFC by transvaginal sonography; and (4) the CCCT. Measurement of cycle day 3 inhibin B concentration can also be used to assess the size of the ovarian follicle and oocyte pool, but this test is seldom used in clinical practice because of technical difficulties in the measurement of this analyte. In the normal menstrual cycle, decreases in estradiol and inhibin A during menses are associated with an increase in FSH production during the first 5 days of the menstrual cycle (see Chapters 7 and 8 ). The magnitude of the rise in FSH during menses is dependent on the magnitude of negative feedback provided by the circulating inhibin B concentration, a constitutively secreted hormone from small follicles. The greater the number of small follicles, the greater the inhibin B concentration during menses, the greater the inhibin B negative feedback on FSH, and the smaller the rise in FSH during menses. The increase in FSH during menses stimulates the growth of an ovarian follicle that will be selected to achieve dominance during the cycle. As the selected follicle grows, it secretes increasing quantities of estradiol, inhibin B, and inhibin A, thereby suppressing FSH production to low levels. As the follicular pool declines, inhibin B levels during menses decline and the magnitude of the FSH increase during menses increases, resulting in elevated serum FSH concentrations on cycle days 2, 3, and 4. A cycle day 3 FSH greater than 10 mIU/mL is associated with reduced fecundability, which is likely due to an aging ovarian follicle pool. Some women with diminished ovarian reserve are noted to have estradiol greater than 80 pg/mL on cycle day 3. This finding suggests that there was an increase in FSH late in the luteal phase, accelerating follicle growth during menses. Consequently, an estradiol greater than 80 pg/mL is a sign of diminished ovarian reserve. AMH is expressed by granulosa cells from small follicles, so as the follicle pool declines, serum AMH decreases. AMH levels are relatively constant throughout the menstrual cycle, increasing the convenience of obtaining a clinically useful AMH measurement compared with the timed measurements required for the interpretation of FSH values. An elevated serum FSH on cycle day 3 or a decreased cycle day 3 inhibin B or a decreased AMH at any time during the cycle are good biochemical markers of a depleted follicular pool. A depleted follicle and oocyte pool is associated with decreased fecundability, an increased rate of pregnancy loss, and an increased rate of aneuploid pregnancy. Particularly in infertile women older than 35 years of age, measurement of AMH, cycle day 3 FSH, CCCT, or AFC are very helpful in identifying women with a reduced follicular pool. In young healthy women without infertility, testing for diminished ovarian reserve is generally NOT useful in predicting time to pregnancy or fecundability.
AFC is the sum of the number of antral follicles 2 to 10 mm in diameter in each ovary assessed in the two-dimensional ovarian slice with the greatest area by high-resolution transvaginal sonography. AFC is commonly assessed during the early follicular phase of the menstrual cycles, but some authorities report it can be performed at any time in the menstrual cycle. An AFC of 4 or less is associated with a poor follicular response to ovarian hyperstimulation, resulting in the development of a low number of large antral follicles, and in IVF cycles, the retrieval of a low number of oocytes. An AFC of 10 or less is also associated with reduced ovarian response compared to an AFC greater than 10. The AFC has good interobserver reliability, and the AFC shows much more variation between women than within the same woman measured in multiple tests.
During menses, negative feedback control of FSH is largely from inhibin B, and to a lesser degree from estradiol. Following the administration of an estrogen antagonist such as clomiphene, the estradiol negative feedback is blocked and FSH negative feedback is only from inhibin B, resulting in a greater rise in FSH. This may increase the sensitivity of the measurement of FSH to detect a depleted follicle pool. The CCCT is performed by administering clomiphene, 100 mg daily for 5 days from cycle day 5 to 9. FSH levels are drawn on cycle days 3 and 10. An elevated FSH level on either cycle day 3 or 10 is associated with a diminished ovarian follicular pool and reduced fecundability. In some series, for every 100 women with an elevated day 10 FSH (postclomiphene challenge), only 40 have an elevated day 3 FSH (preclomiphene challenge). Women who may be candidates for the CCCT include those over 35 years of age, cigarette smokers, and women with unexplained infertility, stage III or IV endometriosis, previous bilateral ovarian surgery, or history of poor response to FSH stimulation. Investigators have reported that the measurement of FSH after a course of CC is more sensitive for identifying women with diminished ovarian reserve than is the cycle day 3 FSH test. Other investigators have reported that basal FSH and the clomiphene challenge test have similar performance in identifying women with decreased fecundity due to a depleted follicle pool.
Biological markers of diminished ovarian reserve may be discordant. For example, among 5354 women 20 to 45 years of age being treated at fertility centers, 20% had AMH and day 3 FSH measurements that were discordant. Of women with reassuring day 3 FSH measurements (FSH <10 mIU/mL), AMH was low (<0.8 ng/mL) in 9% of women less than 35 years of age and 33% of women more than 40 years of age. More data are needed to fully understand the clinical implications of discordant biological markers of diminished ovarian reserve.
Dozens of studies in IVF have reported that the number of mature follicles stimulated and the number of oocytes retrieved is related to basal FSH, inhibin B, AMH, and AFC. In a classic study of the relationship between cycle day 3 FSH and pregnancy rate in an IVF program, women with a day 3 FSH concentration of less than 10 mIU/mL had an ongoing pregnancy rate of 0.18. In contrast, women with a cycle day 3 FSH level greater than 25 mIU/mL had an ongoing pregnancy rate of 0.00. The cycle day 3 FSH predicts the magnitude of the ovarian response to exogenous gonadotropin stimulation—including the peak estradiol concentration, the number of follicles, and the number of oocytes that are obtained at follicular aspiration. Since measures of the size of the ovarian follicular pool predict the magnitude of the ovarian response to stimulation, these measures can be used to guide the choice of stimulation regimens. For example, using a basal AMH test to guide selection of stimulation regimens, women with low AMH levels (reduced follicle pool) would be stimulated with higher doses of gonadotropins, and women with higher levels of AMH (large follicle pool) would be stimulated with lower doses of gonadotropins. Measures of the size of the follicle pool, such as AMH, can also be used to assess the magnitude of the decrease in the ovarian follicle pool following ovarian cystectomy for diseases, such as endometriosis, or chemotherapy.
Many lifestyle factors and genetic factors determine the rate of follicular loss and the age at which serum FSH measured during menses begins to rise. For example, cigarette smoking appears to hasten the pace at which the follicular pool is depleted. Menopause occurs significantly earlier in women who smoke. In women in their mid-30s, cycle day 3 FSH also appears to be approximately 25% higher in cigarette smokers than in non-smokers. In cigarette smokers, the number of oocytes per pair of ovaries appears to be reduced.
Pelvic radiation and chemotherapy with alkylating agents are two important exposures that are associated with a diminished follicular pool. Women who are older than 30 years of age and have completed six courses of chemotherapy for Hodgkin disease typically lose more than 90% of their follicles and many enter menopause immediately after the chemotherapy. Radiation doses as low as 400 rad to the ovary will induce menopause in women over 35 years of age. Girls are much more resistant to the induction of menopause with chemotherapy or pelvic radiation, probably due to their large follicular pool.
Cancer Treatment and Infertility
Issues related to fertility preservation and the treatment of infertility in cancer patients, including oocyte and ovarian cortex cryopreservation, is discussed in Chapter 33 , Fertility Preservation.
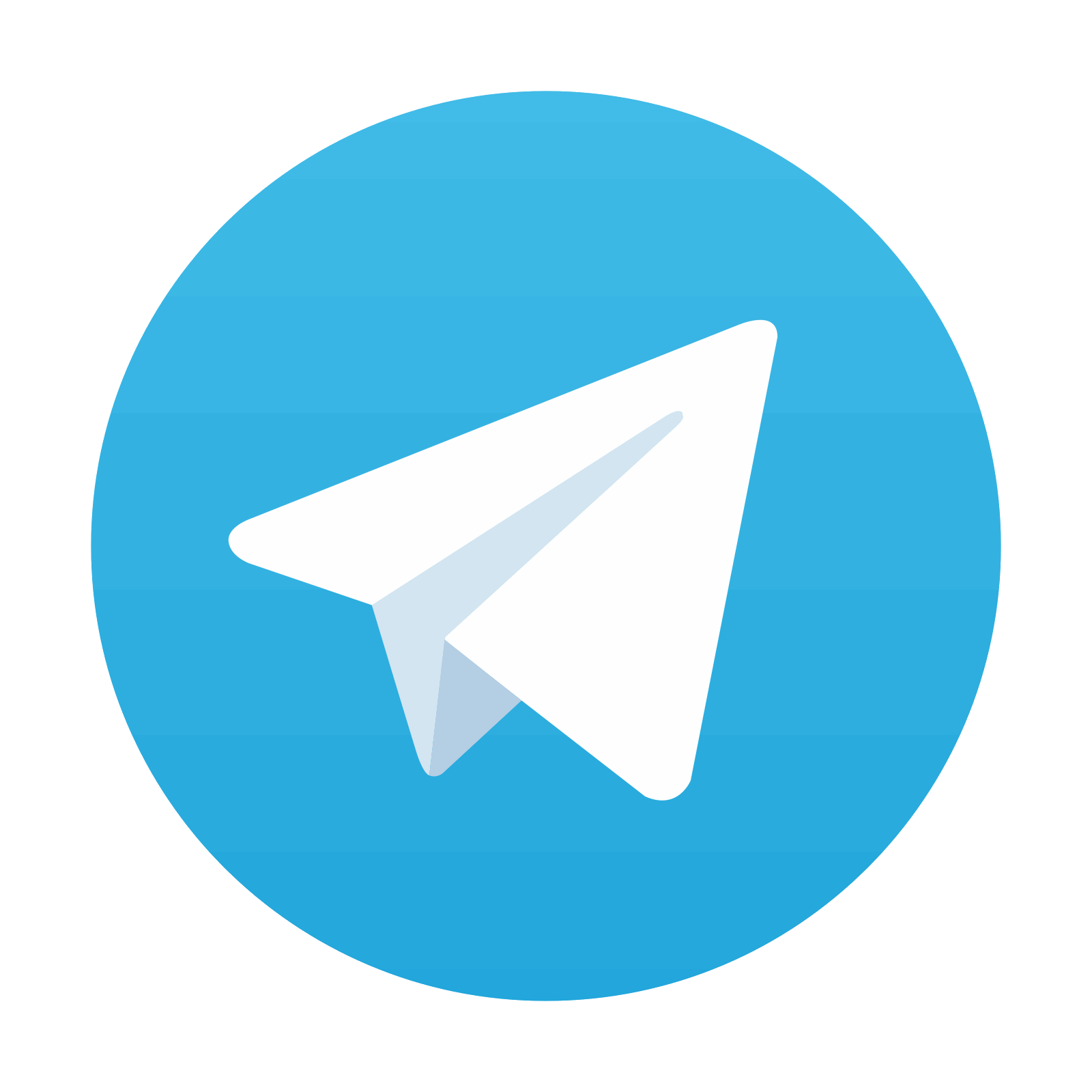
Stay updated, free articles. Join our Telegram channel

Full access? Get Clinical Tree
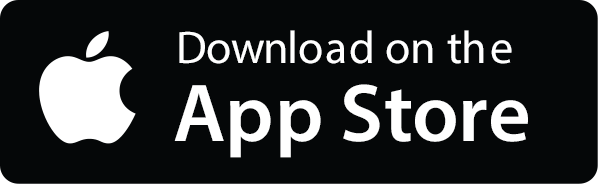
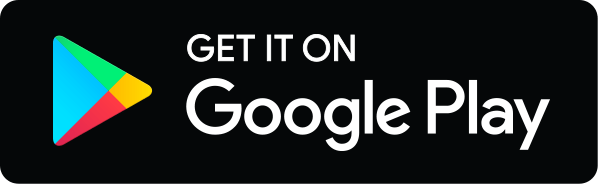