Fig. 31.1
Clinical flowchart for melanoma management. This diagram is simplified from the 2014 National Comprehensive Cancer Network Clinical Practice Guidelines [24]
Light-based visual technologies were developed in the 1990s to help with the visualization of the skin. Dermoscopy (also called dermatoscopy) uses a hand-held, magnifier (typically 10×) with polarized light as a non-invasive method to better examine pigmented lesions. Contact dermoscopy (or epiluminescence) uses a thin layer of oil or alcohol to reduce light reflectance while newer, non-contact dermatoscopes use polarizing light filters to achieve a similar effect. With the removal of this obscuring visual “noise,” it becomes possible to appreciate skin structures that would otherwise be unappreciated by the eye alone [10]. Pattern recognition across a number of variables and a general overall impression of a lesion, rather than a strict set of guidelines for required characteristics, is the most widely used method and has proven to be useful in the detection of melanoma.
Several novel techniques are being developed to help in the early diagnosis of melanoma, including digitized dermoscopy, laser-based imaging methods, ultrasound techniques, and lesion electrical conductance measurement [9]. One preliminary molecular method involves extracting mRNA from superficial epidermal cells removed by applying an adhesive tape over the lesion. mRNA is isolated from these cells to perform gene expression profiling to identify specific molecular alterations [11].
Wachsman and colleagues used this tape-stripping method to compare gene expression levels between melanoma and benign nevi, identifying 17 genes that might be used to detect malignancy. They evaluated this 17-gene classifier on a test dataset of 39 melanomas and 89 nevi, resulting in 100 % sensitivity and 88 % specificity. The majority of the genes are involved with cell death and cellular development [11].
31.1.4 Clinical Subtypes of Melanoma
Melanoma is a heterogeneous cancer, manifesting in a range of clinical subtypes. This heterogeneity has been classically captured by four subtypes characterized by morphological aspects of the radial growth phase and body site of the primary tumor: superficial spreading melanoma (SSM), lentigo maligna melanoma (LMM), nodular melanoma (NM), and acral lentiginous melanoma (ALM). The clinical characteristics and epidemiology of these four subtypes are described below.
SSM is the most common subtype, responsible for 50–80 % of all melanomas [12, 13]. This subtype often arises from a preexisting collection of benign melanocytes called nevi, slowly evolving into a malignancy over months to years. SSM typically have irregular borders and pigmentation, containing hues of brown, black, red, and white [14]. NM is the second most common subtype of melanoma, accounting for 20–30 % of all melanomas [12, 13]. These lesions are found most commonly on the trunk and may not be associated with preexisting nevi. In contrast to SSM, NM rapidly develops over weeks to months. NM tends to be raised on palpation and can appear dark blue-to-black, but may also appear amelanotic. Notably, analysis from SEER data from 1978 to 2007 found that SSM accounted for 66 % of incident melanomas and 46 % of fatal melanomas, NM accounted for 14 % of all melanomas and 37 % of melanoma deaths. Thus, despite accounting for fewer melanomas in total, NM accounts for a similar proportion of mortality as SSM. These findings are concordant with the observation that tumor thickness is an important prognostic factor in melanoma [13].
LMM is the third most common melanoma, accounting for 10–15 % of melanomas [12, 15]. LMM typically occurs in the elderly on chronically sun-damaged skin such as the head, neck, and forearms. Clinically, LMM presents as a flat, brown tumor and may typically appear with irregular borders. LMM differs from NM and SSM in that it typically does not have a nevus precursor, occurs more frequently in older individuals, and has a longer prolonged period of intra-epidermal growth as compared with SSM [14]. In regards to trends over time, SEER analysis revealed that LM is the most prevalent in situ subtype (79–83 %) and that incidence of LMM is increasing at a higher rate than other subtypes, especially in older men [15].
Finally, ALM is the least common subtype of melanomas, but drastically differs in prevalence among ethnicities [16]. This subtype accounts for the majority of melanomas among individuals with darker pigment, representing the majority of melanomas in African Americans and Asian populations [17–20]. In Caucasian populations, ALM only represents approximately 5 % of melanomas [16]. ALM is most commonly found on hairless areas of the body, including the soles, palms, and subungual regions. ALM typically appears as a flat lesion that develops irregular borders but can present with variable pigmentation and nail plate destruction. Subungual ALM typically arises from the nail matrix on the great toe or thumb and develops into a longitudinal black band. Notably, ALM appears to have a greater frequency of focused gene amplifications as compared to other subtypes, such as SSM [21].
31.1.5 Staging
When melanoma is confirmed by histopathology, staging of disease is important for treatment decisions and prognosis estimation. The American Joint Committee on Cancer (AJCC) has developed a tumor-node-metastasis (TNM) based clinical and histologic scheme that incorporates important prognostic indicators such as metastases, tumor thickness, ulceration, and mitotic activity [22]. The details of this staging scheme will be discussed in greater length in Sect. 31.4. Clinically, macroscopic metastasis can often be detected by physical exam, looking for palpable lymph or dermal/subcutaneous nodules around the area of the primary lesion. If a nodule concerning macroscopic metastasis is appreciated, fine needle aspiration or biopsy of the nodule is indicated. Subclinical, microscopic metastases can only be identified by lymph node biopsy.
In aggregated, localized melanoma (Stage I or II) is associated with 5-year survival rates of >90 % and 80 % respectively [23]; however, many features such as ulceration and lymphovascular invasion may reduce the survival rate. In contrast, survival decreases to 30–50 % with localized lymph node metastases (Stage III) disease. Distant metastatic disease (Stage IV) has historically been linked to a median survival of 8–9 months and a 15 % 3-year survival rate [22]. However, this survival data, reported in the most recent AJCC guidelines in 2009, predates much of the recent exciting advances in melanoma treatment.
31.1.6 Treatment
31.1.6.1 Surgery
Wide surgical excision is the main treatment for primary cutaneous melanoma, with the goal of directly resecting all malignant cells from the primary site. The National Comprehensive Cancer Network (NCCN) Clinical Practice Recommendations vary regarding the extent of clean surgical margins required for melanoma based on tumor depth: melanoma in situ and primary melanomas of depth ≤1.0, 1.01–2, 2.01–4, and >4 mm are recommended to be excised with clean margins of 0.5–1, 1, 1–2, 2, and 2 cm, respectively [24]. In the presence of regional metastasis, complete lymph node dissection is recommended of the surrounding regional lymph node basin. Interferon-α [alpha] 2b (both pegylated and non-pegylated) and ipilimumab have been approved by the US Food and Drug Administration (FDA) for adjuvant treatment in the setting of Stage III disease.
31.1.6.2 Systemic Therapy
Cytotoxic chemotherapy was the mainstay of treatment for metastatic melanoma for over three decades, but treatment options are fast evolving with the development of novel agents propelled by the revolution in our molecular understanding of melanoma and immunology (see Fig. 31.2).


Fig. 31.2
Timeline of FDA-approval of melanoma drugs
31.1.6.3 Chemotherapy
Alkylating agents, platinum analogs, and microtubular toxins have all been used to treat melanoma [25]. However, dacarbazine, an alkylating agent, is the only FDA-approved chemotherapeutic agent for metastatic melanoma, with a demonstrated 10–20 % response rate that has limited durability [25, 26]. In addition to monotherapy, combinations of multiple chemotherapeutic agents have also been tested. These combined regimens have yielded very modest response rates that are typically less than 20 % in first and second line settings [24].
31.1.6.4 Immune Therapy
Several clinical observations suggest that melanoma is an immunogenic tumor. Melanoma has been transmitted from immunocompetent organ donors to immunosuppressed organ recipients [27–30]. Spontaneous regression of melanoma lesions has been observed, and approximately 3 % of all melanomas present with metastatic disease without a known primary lesion, which may be due to regression of the primary lesion from immunosurveillance [31, 32]. In addition, the presence of tumor-infiltrating lymphocytes in lesions may be associated with longer survival [32, 33].
Before 2011, immunotherapy was limited to cytokine-based treatment with IFN-α[alpha] in the adjuvant setting and high-dose interleukin-2 (IL-2) in Stage IV disease. Interferon’s mechanism of action as an adjuvant therapy remains unclear as it is an immunomodulatory and anti-angiogenic cytokine that can promote melanocyte apoptosis [36–39]. Moreover, its use remains controversial given that it has been demonstrated to improve disease free survival but not overall survival, while having a severe side-effect profile including neuropsychiatric, constitutional, and hepatic toxicity [34, 35]. Studies have not demonstrated consistent survival benefit [36, 37]. IL-2, a T cell growth factor, was subsequently developed and approved to treat patients with metastatic melanoma, with clinical trials demonstrating a modest but durable 16 % response rate [38, 39].
Since 2011, monoclonal antibody targeting of immune activating checkpoint blockade has emerged as a field-changing addition to our therapeutic armamentarium. Immunosurveillance begins with T cell recognition of malignancy-generated, intracellular peptides that are presented in the context of MHC-I molecules on the surface of tumor and other antigen presenting cells. This interaction is facilitated by receptor-specific, T cell binding of B7 on antigen presenting cells. CD28 and CTLA-4 receptors on the T cell surface compete to bind to B7, either resulting in a T cell stimulatory or inhibitory signal, respectively. Thus, CTLA-4 serves as a “brake,” regulating the amplitude of early T cell activation [40, 41]. In 2011 and 2015, the FDA approved the first monoclonal antibody to CTLA-4, ipilimumab, for use in Stage IV and Stage III melanoma, respectively. Ipilimumab removes this brake and results in a more immune-activated state. Another relevant immune checkpoint is the inhibitory receptor programmed cell death receptor-1 (PD-1) on activated T cells. This inhibitory receptor, when bound to tumor associated PD-L1, downregulates the immune response [40]. The FDA approved two monoclonal antibodies targeting PD-1, pembrolizumab and nivolumab, in 2014.
31.1.6.5 Targeted Therapy
Therapies that target a specific mutated protein implicated in melanomagenesis have become an important addition to our therapeutic arsenal. In 2002, it was discovered that 50 % of all melanomas carry a mutated protein kinase B-raf (BRAF), which constitutively activates the pro-proliferative mitogen-activated protein kinase (MAPK) pathway [42]. 75 % of BRAF mutations are the result of a valine to glutamate substitution at the 600th amino acid (V600E)[43]. Given the proportion of tumors bearing this mutation and the mutation’s oncogenic potential, researchers began to search for an inhibitor. Sorafenib, an early BRAF inhibitor, proved to be a poor treatment for melanoma given an intolerable side-effect profile due to inhibition of wild type BRAF and other off-target effects [44]. In contrast, V600E-specific BRAF inhibitors—including vemurafenib and dabrafenib—have been developed with stunning results. In the phase III study, vemurafenib yielded a 48 % response rate and an overall survival advantage among metastatic melanoma, as compared with dacarbazine [45].
Despite these triumphs, the greatest obstacle for targeted therapy has been the rapid development of drug resistance after months of treatment. Approximately 50 % of patients treated with BRAF inhibitors demonstrate progression of disease after several months [46, 47]. Genomic profiling of tumors have helped identify pathways to resistance, including mutations in the downstream kinase, mitogen/extracellular signal-regulated kinase (MEK), that results in recovery of MAPK [48]. Other pathways to resistance that have been identified include mutational activation of NRAS, emergence of alternative BRAF splice products resistant to treatment, and BRAF amplification [49].
Combination therapy with BRAF inhibitors and an inhibitor of MEK has been tested with promising results. For instance, the combination of dabrafenib with trametinib, a MEK inhibitor, yields higher response rate and longer progression-free survival as compared to the BRAF inhibitor alone [50]. Studies exploring different combinations are currently underway and will hopefully yield higher, durable response rates.
31.1.7 Prognosis
Beyond the prognostic information that staging provides, several studies have searched for molecular signatures that may be predictive of clinic course. Whole-genome expression profiling has been conducted in both local and aggressive melanomas to find genes that might predict melanoma progression. An early study identified 254 genes that were associated with progression [51]. Another study using RNA profiling of primary tumor samples demonstrated that high-grade tumors had increased expression of proliferation and DNA damage signaling genes, while low-grade melanomas had increased expression of immune genes [49, 52]. Another study found a nine gene-signature predicted overall survival and distant metastasis-free patient survival that was independent of AJCC staging. Many of these genes coded for stromal-related proteins, suggesting an important interaction between the malignancy and its surrounding micro-environment in predicting prognosis [53].
31.1.8 Other Melanoma Types
While the focus of the chapter is on cutaneous melanoma, we will briefly discuss two other forms of melanoma, ocular and mucosal, to present a comprehensive overview of melanoma.
31.1.8.1 Ocular Melanoma
Ocular melanoma is the most common intraocular cancer and the second most common melanoma after cutaneous melanoma, accounting for 3.7 % of all melanoma cases [54]. In the United States, incidence of ocular melanoma is six per a million [54]. Ocular melanoma rates are 8–10 times higher in Whites as compared to Blacks, but it should be noted that the difference is less pronounced when compared to cutaneous melanoma, in which 16 times higher rates among Caucasians are noted [54]. These melanomas arise from melanocytes positioned in the conjunctival membrane and the uveal tract of the eye [55]. However, the uvea is the most common site of origin of primary ocular melanomas, representing over 80 % of all ocular melanomas [54].
While melanoma can arise anywhere along the uveal tract, choroidal melanoma accounts for the majority of uveal melanomas, representing over 80 % of cases [54]. The clinical presentation of uveal melanoma depends primarily on the size and location, ranging from asymptomatic to complete vision loss in the affected eye. The most frequently reported symptoms include blurred vision, visual field defects, and irritation [55]. Diagnosis is frequently made by ophthalmic examination, ranging from slit lamp biomicroscopy and indirect ophthalmoscopy to ancillary testing such as ultrasonography [55]. Management of tumors varies between careful observation to orbital exenteration, depending on the size and location. Most individuals will obtain radiotherapy or enucleation. Regarding prognosis, less than 4 % of patients with uveal melanoma have detectable metastatic disease, but over half will develop metastatic disease due to the lack of effective systemic treatments [56]. Size of tumors is one of the most important prognostic factors for uveal melanoma. Risk of metastasis appears to increase with each millimeter increase in tumor thickness [57].
Similar to cutaneous melanoma, much has been learned about the molecular etiology of ocular melanoma. Among uveal melanomas, 80 % carry activating mutations in either GNAQ or GNA11. These genes encode for the GTP-binding protein α [alpha]—subunits that link G-protein-coupled receptor signaling to the MAPK pathway, resulting in constitutive activation of the MAPK pathway [58, 59]. In addition, inactivating mutations in BAP1 (BRCA1 associated protein-1), a deubquitinating enzyme, has been linked to a subgroup of uveal melanomas that are most likely to metastasize [60].
31.1.8.2 Mucosal Melanoma
Mucosal melanomas are relatively rare, representing only 1 % of all melanomas [54]. In the United States, there are approximately 2.2 mucosal melanoma cases per million per year [54]. Primary mucosal melanomas may arise from melanocytes lining the respiratory, gastrointestinal, or urogenital tracts [61]. Mucosal melanomas in the respiratory tract are most frequently found in the nasal cavity and paranasal sinuses, and the most common symptoms include nasal obstruction and epistaxis, with patients first exhibiting epistaxis before exhibiting obstructive symptoms [62]. In contrast, mucosal melanomas arising from the gastrointestinal tract occur most frequently in the anorectal and oropharyngeal regions, and the most common symptoms include bleeding, pain, and discomfort [63]. Finally, mucosal melanomas of the urogenital tract are more common in females, and occur most frequently on the vulva and vaginal canal, with common presenting symptoms of vaginal bleeding and discharge [54]. In general, mucosal melanomas are typically discovered after work-up of presenting symptoms and are more advanced in nature than cutaneous melanomas given their hidden locations.
Mucosal melanomas differ from cutaneous and ocular melanomas in several notable ways. Unlike other subtypes of melanoma, there is a female predominance as compared to men (2.8 vs. 1.5 per million per year), which is related to the higher rates of genital tract melanomas found in women [54]. In addition, sun radiation is an important risk factor for cutaneous and uveal melanomas (see Sect. 31.3 for discussion), but is not thought to contribute risk for mucosal melanoma, given the shielded nature of the sites. Prognostically, primary mucosal melanomas are much more aggressive, with one study finding that the 5-year survival rates for cutaneous, ocular, and mucosal melanomas were 80.8, 74.6, and 25 %, respectively [61]. Finally, the molecular etiology of mucosal melanoma appears to be different than either cutaneous and ocular melanomas. Mucosal melanomas often harbor KIT (receptor tyrosine kinase) amplifications or mutations. KIT-inhibitors, such as imatinib and sunitinib, have been trialed with some limited response [64, 65]. Given the rarity of mucosal melanoma and that only a subset carry KIT aberrations, no large clinical trials have been possible [62]. Like cutaneous melanoma, surgery is the primary treatment with potential roles for chemotherapy and immunotherapy [62].
31.2 Descriptive Epidemiology
31.2.1 Geographic Trends
According to World Health Organization, there were over 230,000 new cases of melanoma and over 55,000 melanoma-related deaths worldwide in 2012 [66]. Notably, the burden is not constant across populations; geographic heterogeneity in melanoma incidence is a striking feature of this malignancy. Australia and New Zealand report the highest reported incidence rates with approximately 50 cases per 100,000 individuals per year [67–69]. In the United States, rates are approximately 20 cases per 100,000 per year, while Qatar cataloged only 0.3 cases per 100,000 per year (see Fig. 31.3) [66].


Fig. 31.3
Global crude melanoma incidence rates. Data presented here was downloaded from the International Agency for Research on Cancer [66]
Generally, individuals living at lower latitudes experience higher rates of melanoma than those in higher latitudes. For instance, in Australia, residents of Queensland (capital city Brisbane, latitude 27°S) have higher rates of melanoma (65:100,000 per year) than residents of Victoria (capital city Melbourne, latitude 38°S; 36:100,000 per year) [70]. Indeed, similar latitude gradients have been observed in other regions, including the United States, New Zealand, and Scandinavia [69–72]. These observations may be explained, in part, by higher rates of ultraviolet (UV) exposure at lower latitudes, but as discussed in Sect. 31.3, the relationship between UV exposure and melanoma risk is not a simple dose response. Notably, a latitude gradient is not observed in all areas; for instance, in Europe, southern populations experience lower rates of melanoma than populations in the north, which is thought to be related to the protective effects of darker pigmentation of populations in this southern region [70, 73].
31.2.2 Trends Over Time
Melanoma is an ancient disease: skeletons in Peru, dating over 2000 years old, have findings consistent with metastatic melanoma bone lesions [74]. However, at the beginning of the twentieth century, melanoma was a relatively uncommon cancer, with the earliest epidemiological report dated in the United States to the 1930s, estimating an incidence of 1.0 per 100,000 [75, 76]. Melanoma’s incidence has been steadily increasing in the fair-skinned population since the mid-1960 s and has been projected to increase for, at least, another two decades [77–79]. The annual incidence increase varies across populations, ranging from 3 to 7 %, but indicates a doubling of rates approximately every 10–20 years [80]. In the United States, melanoma incidence rates are among the fastest growing cancers based on Surveillance Epidemiology and End Results (SEER) data (Fig. 31.4) [81]. This trend is thought to be driven by changes in risk-modifying behavior, including clothing, sun-seeking behavior, and skin awareness/ screening efforts (see Sect. 31.3) [82, 83].


Fig. 31.4
Percent change of age-adjusted incidence from 1975 rates among 9 cancers. Data downloaded from SEER database [81]. Notably, melanoma has the highest percent increase in incidence
Mortality rates appear to have peaked in the late 1980s to early 1990s in Australia, the United States, and parts of Europe (See rates in Fig. 31.5) [84]. Some have suggested that this dissociation between increasing incidence and stabilizing mortality rates may be the result of “over-diagnosis” [85, 86]. However, the distribution of melanoma thickness has remained stable and an alternative hypothesis is that the improvement in intervention may contribute to the observed mortality trend [87, 88].


Fig. 31.5
Age-adjusted incidence and mortality rates in the United States. Data collected and analyzed by SEER [81]. Age-adjusted to the 2000 US standard population
31.2.3 Trends by Sex
In the United States, melanoma incidence is higher in females than in males in the age group less than 44 and is the most common cancer in females in the age group between 15 and 24 (See Fig. 31.6) [84, 89]. Indeed, melanoma incidence appears to be increasing at alarming rates in young women. An analysis of the SEER data suggests that the age-adjusted rate of melanoma more than doubled from 8.1 to 17.4 per 100,000 between 1975 and 2008 among a relatively young age group (20–49 years), while incidence among men in this age group changed more modestly from 8.3 to 12.5 per 100,000 during the same time interval [89, 90]. These observations are most likely related to indoor tanning use among the young female demographic. However, the burden of deaths remain higher among young males versus young females in the United States, even after adjusting for tumor thickness and other prognostic factors [91]. Young men accounted for approximately 40 % of all cases but 60 % of all melanoma deaths among young adults. Indeed, males uniformly appear to do worse at all tumor thickness, histological subtypes, and anatomic sites [92]. A recent study observed that melanomas excised from men were more likely to have greater missense mutation burden than tumors from women, even after adjusting for important confounders such as age, stage, and site of primary tumor [93]. This observation supports the possibility of a biological mechanism underpinning the epidemiological sex discrepancy in melanoma outcome, which remains to be elucidated. One possibility is that sex differences in immunity may lead to more effective cancer surveillance and tumor clearance in women [93].


Fig. 31.6
Age-specific incidence and death rates in the United States between 2007 and 2011. Data collected and analyzed by SEER [81]
31.2.4 Patterns by Anatomic Site
The odds of developing melanoma by a specific anatomic site vary by sex. In general, women have an increased proportion of lesions on their legs, while males have an increased proportion of cancers on their trunk [94–96]. These differences are thought to be related, in part, to behaviors that are sex-specific, such as clothing, hairstyles, sun-seeking behavior, among others. Indeed, epidemiological trends suggest a non-biological component. As previously noted, melanoma incidence has been increasing at an alarming rate among young women. The increase in incidence has been most pronounced on the trunk region—a site hypothesized to be less shielded to UV exposure due to increasing popularity for full-body, indoor sun tanning [90].
When adjusting for anatomic surface area and thus yielding a proportion of melanoma per a unit of area, both sexes have highest propensity on the face [97]. The head and neck region accounts for 9 % of total body surface area, yet accounts for approximately 12–20 % of all cutaneous melanomas [98]. This increased propensity is likely related to the fact that the head and neck region has one of the highest densities of melanocytes and also is exposed to the highest level of UV radiation [98, 99]. Interestingly, sex-related differences on the head and neck location have also been noted: in a study of patients in France, head and neck melanomas in males were found in peripheral areas of the head and neck (scalp, forehead, temples, ears and neck), while these melanomas were more likely to be found in central facial regions (nose and cheeks) in women [98]. These gender differences likely suggest a photo-protective effect of hair.
The distribution of melanomas by anatomic site appears to significantly differ by age. Two studies in different populations both found that area-adjusted incidence of melanoma were much higher on the trunk among young adults than on the head and neck. The area-adjusted incidence on the head and neck appeared to increase rapidly in older individuals [69, 100]. This distribution is notable as it potentially suggests two distinct pathways for melanoma development that will be discussed more in Sect. 31.2.6.
There is some evidence that melanoma prognosis may differ by anatomic site. As noted in Sect. 31.1, melanoma prognosis and staging is calculated using thickness of tumor, ulceration, and nodal and distant metastases. However, individuals with melanoma of the scalp and neck died of melanoma almost at twice the rate of melanomas found on the extremities, even after controlling for age, tumor thickness, gender, and ulceration [101].
31.2.5 Patterns of Somatic Mutations
Mutations in genes in the pro-proliferative MAPK pathway play a significant role in melanomagenesis. In particular, mutations in BRAF and NRAS genes have been identified in a majority of melanomas. Constitutively activating BRAF mutations and NRAS mutations have been found in approximately 40 % and 18 % in cutaneous melanomas, respectively [102]. Approximately 75 % of all BRAF mutations appear to be the result of a T1799A transversion in exon 15 of the gene, causing a valine to glutamate amino acid substitution in the protein. It is important to note that 80 % of melanocytic precancerous nevi contain BRAF mutations, suggesting that these mutations may be necessary but not sufficient to drive malignant transformation [103]. The most common NRAS mutations occur in codon 61 and result in a glutamine to arginine or lysine transition that results in NRAS activation [102].
Notably, mutations in BRAF and NRAS are thought to be mutually exclusive and do not appear to co-exist in the same tumors, suggesting that double-hits in both genes may not be well tolerated. BRAF-mutated melanomas have been associated with younger age at diagnosis, localization to trunk site, and absence of chronic sun damage. In contrast, NRAS-mutated melanomas have been associated with thicker tumors and occurrence on the extremities [102].
31.2.6 Implications of Epidemiology Trends
The observation that melanomas in non-chronically exposed areas, such as the trunk, peak in incidence at earlier ages than chronically sun-exposed areas, such as the head and neck, led to the hypothesis of “two divergent pathways” in melanomagenesis [104]. This model hypothesizes that individuals with low propensity for melanocyte proliferation—i.e., those persons with low density of benign melanocytic tumors called nevi—need chronic sun exposure leading to melanomas on anatomic sites with habitual exposure such as on the head and neck. On the other hand, some patients have a high propensity for melanocyte proliferation—i.e., those individuals with high density of nevi—develop melanomas on sites with intermittent sun exposure such as on the trunk [70, 104]. Molecular studies that subsequently demonstrated higher proportion of BRAF mutations in truncal melanomas and higher proportions of NRAS mutations in more chronically exposed regions reified the notion that different processes likely contribute to melanomagenesis in these respective populations. However, differences in risk factors and causes of these pathways are not well understood.
31.3 Risk Factors
The “divergent pathway” hypothesis incorporates our knowledge about two risk factors, sun exposure and nevi (one is environmental and the other, in part, genetic). It is clear that melanoma develops from a complex interaction between environmental and host-specific factors, which are explored further in this section. Traditional host factors, such as pigmentation, nevi, and highly penetrant risk genes, are discussed first, followed by non-hereditary risk factors, including ultraviolet (UV) radiation—the most important environmental risk factor for melanoma—as well as evidence for risk modulation by commonly used drugs.
31.3.1 Host Factors
31.3.1.1 Pigmentation
Fair-skinned individuals have higher rates of melanoma. Photoprotection of the skin is based primarily on the amount and type of melanin produced in the skin. The melanocortin 1 receptor (MC1R) is an important regulator of skin pigmentation, and is also the most studied germline melanoma-susceptibility locus. MC1R codes for a seven-transmembrane G-protein coupled receptor that, when bound to α[alpha]-melanocyte-stimulating hormone (MSH), increases cAMP levels within the cell. MSH binding ultimately regulates the type of pigment produced, switching from the production of the potentially mutagenic red/ yellow pheomelanin pigment to the photo-protective brown/ black eumelanin pigment [105].
MC1R is highly polymorphic, with over 75 allelic variants, and contributes to variation in skin phenotypes in humans [106]. Wild type MC1R is predominantly expressed in Africa, where high levels of eumelanin are important for photoprotection. It is thought that photoprotection could not have evolved to protect against skin cancer, given that these cancers only rarely cause death to individuals during ages of reproductive age. The evolutionary pressure that led to the development of photoprotection is debated, but the folate deficiency hypothesis suggests that folate, an essential nutrient required for nucleotide and hence DNA biosynthesis, is degraded by UV light, and dark pigmentation evolved to protect against this degradation [107].
Several MC1R variants that result in amino acid substitutions typically decrease receptor function, increasing the amount of pheomelanin in the cell. These increased levels result in red hair, pale skin, poor tanning ability, and freckles. MC1R variants likely contribute to melanoma risk by two mechanisms: modulating pigmentary contribution to UV protection as well as generation of reactive oxygen species [49]. Indeed, individuals with darker phenotypes still carry increased risk of melanoma with MC1R mutations [108]. Interestingly, some MC1R alleles appear to be double risk of melanoma for each allele carried by a patient, with an additive effect observed when carrying multiple alleles. In one meta-analysis, five MC1R alleles (D84E, R142H, R151C, I155T, R160 W) were statistically significantly associated with developing melanoma and the phenotype of red hair color and fair skin [109]. The meta-analysis also identified two variants, R163Q (rs885479) and D294H (rs1805009), that increased melanoma risk, but were not associated with red hair color, supporting the existence of a secondary pathway leading to melanoma risk other than the UV-protective effect of pigmentation [109]. The allele frequency distribution of R163Q is depicted in Fig. 31.7 to show that this allele is found globally and not restricted to populations typically expressing the red hair phenotype (data from [110]). Notably, other pigmentation-related genes have been associated with melanoma susceptibility, including SLC45A2, OCA2, TYR, and ASIP. Variants in these five genes have been reported to explain up to 50 % of the observed difference in melanoma risk contributed by the skin pigmentation phenotype [111].


Fig. 31.7
Global distribution of MC1R R163Q Allele frequency. Data was extracted from the Allele frequency database [110]
31.3.1.2 Nevi
Another melanoma risk factor, which is pigment related, is nevus density. Nevi are benign clonal proliferations of melanocytic cells [112]. Higher number of nevi increases risk of melanoma, with some estimates suggesting that each additional common nevus increases risk by 1.02 [113–115]. Twins studies support the hypothesis that the propensity for nevi development is a genetic trait, as monozygotic twins had higher correlations of nevus counts than dizygotic twins [116]. More recently, genome-wide association studies (GWAS) identified two genetic variants, 9p21 and 22q13, associated with nevus development and melanoma [117].
31.3.1.3 Sex
Sex-specific differences in melanoma incidence and mortality are well established: women accounted for 42 % of the 73,870 new melanoma cases but only for 33 % of the 9710 melanoma-related deaths recorded in the United States in 2015 [118]. Population-based studies in other countries reflect a similar trend [119–123]. For instance, a population-based study of 11,000 melanoma patients in Germany found that female melanoma patients were 38 % more likely to survive and 42 % less likely to progress than males [123]. Among patients who did experience disease progression, women kept a 20 % survival advantage [123].
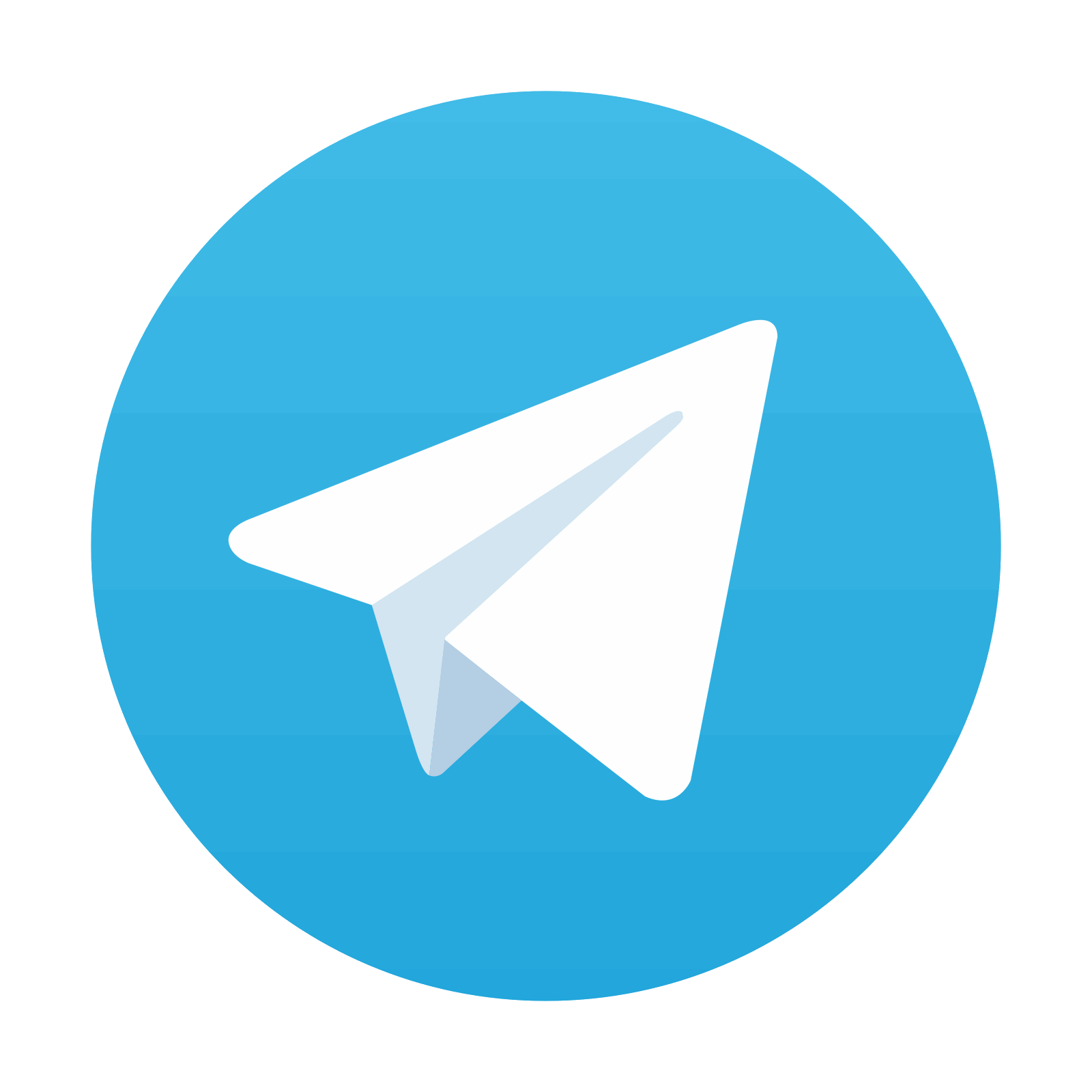
Stay updated, free articles. Join our Telegram channel

Full access? Get Clinical Tree
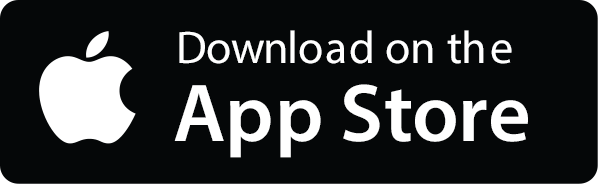
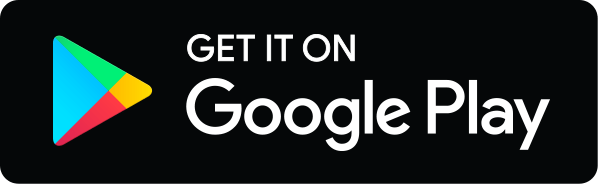