Endovascular Brachytherapy
Vascular brachytherapy (VBT) continues to have a role in the treatment of coronary in-stent restenosis and has a promising role in the treatment of restenosis following intervention of the peripheral arterial system. With the introduction of drug-eluting stents (DESs) midway through the last decade, the incidence of coronary in-stent restenosis has declined following percutaneous coronary intervention.1 The future of VBT in the treatment of coronary in-stent restenosis at one point seemed uncertain. However, after several years of declining use of VBT for in-stent restenosis, some centers including Scripps Green/Scripps Clinic (La Jolla, CA) have recently seen a gradual rise in the number of patients needing VBT for coronary in-stent restenosis as some patients have developed in-stent restenosis following multiple DESs.
Patients with small vessel disease and diabetes are particularly prone to develop coronary in-stent restenosis, with rates as high as 10% or more.2 Repeated percutaneous coronary interventions and surgical revascularization procedures are also associated with high rates of restenosis, with rates as high as 14% being reported.3 DESs can reduce recurrences of in-stent restenosis by 50% to 70%.4 Nonetheless, restenosis still can occur following DESs at rates between 2% and 10%.5
There are more than 1.5 million coronary interventions performed worldwide each year for coronary stenosis.6 Coronary stenting with metallic stents has become a standard of care in treating patients with coronary stenosis. Restenosis has been the major complication of percutaneous transluminal coronary angioplasty since the introduction of balloon angioplasty by Gruentzig in the mid-1970s.7–9 This process has been thought to consist of three separate mechanisms: vascular recoil, neointimal hyperplasia, and negative remodeling.
VBT has been shown in several double-blinded randomized trials to demonstrate its efficacy in reducing rates of in-stent restenosis. The potential usefulness of vascular radiation therapy to prevent restenosis emerged rapidly, from positive preclinical studies carried out in animals in the late 1980s and early 1990s, to a large number of feasibility trials and randomized clinical trials in the mid- to late 1990s, to U.S. Food and Drug Administration (FDA) approval of the first commercial devices in the last quarter of 2000. Although initial preclinical studies have included evaluation of both teletherapy and brachytherapy approaches, only vascular systems have been tested extensively in human coronary vessels.
Of the 1 million angioplasties expected in the United States annually, approximately 80% to 90% of these patients will undergo stenting. In-stent restenosis will develop in approximately 15% of these patients, and these patients present a considerable management problem to the interventional cardiologist. Restenosis rates approaching 80% for long, diffuse lesions in small vessels have been reported.
Studies on DESs have now matured. Interventional cardiologists have implanted several million DESs worldwide.10 DESs using antiproliferative agents such as paclitaxel11 and immunosuppressive agents such as sirolimus12–15 have been shown to be effective in treating in-stent restenosis. Published randomized trials show DESs to be superior to VBT in treating in-stent restenosis.16–18 DESs have replaced VBT as first-line therapy for coronary in-stent restenosis. Additionally, interventional cardiologists may simply prefer DESs over VBT in order to avoid the logistics and expense of having radiation oncology involved in the catheterization laboratory.12,14 However, adverse events such as acute stent thrombosis, aneurysm, and incomplete apposition do occur with DESs, although some randomized trials have not shown a difference in the incidence of stent thrombosis between patients with DESs or bare-metal stents (BMSs).19
VBT has found a niche in treatment of patients who fail DESs. The Checkmate system (Cordis Corporation, Miami Lakes, FL) and the Galileo system (Guidant Corporation, Indianapolis, IN) are no longer commercially available. The Novoste Beta-Cath 3.5F System (Novoste Corporation, Norcross, GA) using 90Sr is the only system clinically available for use in coronary artery in-stent restenosis following repeat intervention.
Approximately 400,000 peripheral vascular procedures are done each year in United States. The risk of restenosis after angioplasty and stent placement varies considerably in different parts of the peripheral arterial tree. Initial clinical trials of VBT in superficial femoral arteries and renal artery in-stent restenosis20,21 show similar results as observed in the coronary system. VBT appears to be safe and effective in certain patients with restenosis in the peripheral vascular system.
HISTORICAL PERSPECTIVE
VBT was empirically tried in Frankfurt, Germany, by Liermann and colleagues on restenosed femoral popliteal arteries starting in 1990. A small cohort of patients has been followed for 10 years, and no long-term adverse events have been reported.22 The first coronary brachytherapy procedure was performed in Caracas, Venezuela, by Condado et al.23 in 1994, and the results of their initial feasibility trial were reported in 1997. At 5-year follow-up, the data from this landmark study are durable and demonstrate the feasibility of brachytherapy for preventing coronary artery restenosis. The first randomized trial of VBT was carried out by investigators at the Scripps Clinic in 1995, and the positive results from this trial were subsequently published in the New England Journal of Medicine.24 That led to GAMMA I, the first multi-institutional, double-blind, randomized pivotal trial using 192Ir, which led to the approval of the Checkmate system (Cordis Corporation, Miami Lakes, FL) for native coronary in-stent restenosis.25
In the mid-1990s, a Geneva group and a second group at Emory University began testing the feasibility of intracoronary brachytherapy using β-emitting sources.26,27 The Beta-Cath System (developed by Novoste Corporation, now owned by Best Vascular, Inc., Norcross, GA), which was piloted at Emory, became the focus of the START trial, the second pivotal trial. In November 2000, it was approved, along with the Checkmate system, for in-stent restenosis.28 The INHIBIT trial testing the Galileo system represents the third pivotal trial of VBT for in-stent restenosis and led to the approval of this device in November 2001.29 The only randomized trial comparing VBT for de novo lesions in native coronary vessels to stents used the Beta-Cath System and was reported as a negative trial.30
The approval of VBT is unique in radiation oncology in a number of ways. It is the first time that level I evidence supported by multi-institutional, randomized trials was required by FDA mandate before VBT became available in the routine clinical setting. Likewise, under FDA and Nuclear Regulatory Commission mandate, it was the first time that all specialists, including radiation oncologists (therapeutic radiologists and oncologists), interventional cardiologists, and medical physicists, were required to be part of the team delivering VBT. Since 1995, more than 6,000 patients have been enrolled in approximately 50 protocols testing the efficacy of VBT.31
CORONARY ANATOMY
(This section is modified with permission from Windecker S, Meier B. Basics of interventional cardiology. In: Tripuraneni P, Jani S, Minar E, et al., eds. Intravascular brachytherapy: from theory to practice. London: Remedica, 2001:83–86.) The coronary arteries originate as the only branches of the ascending aorta from the aortic root.32 Coronary arteries usually have an epicardial course and terminate as arterioles in the capillary network. The left and right coronary arteries surround the epicardial surface as a ring-loop system in two orthogonal planes defined by the fibrous skeleton of the heart. Thus, the right coronary artery (RCA) and the left circumflex coronary artery (LCX) run around the atrioventricular groove and form a circle between the atria and ventricles at the base of the heart. Perpendicular to this plane, the left anterior descending coronary artery (LAD) and the posterior descending RCA constitute a semicircle around the interventricular groove and encircle the left ventricular apex.
Left Coronary Artery
The left coronary artery usually originates from a single ostium in the middle portion of the left sinus of Valsalva (Fig. 92.1). The vessel originating from the left ostium is termed the left main coronary artery (LM) if it subsequently gives rise to both the LAD and the LCX. The LM measures 3 to 10 mm in diameter and is usually <40 mm long. The LM has no side branches and divides into the LAD and the LCX, although in 20% to 40% of cases a trifurcation with an intermediate branch between the LAD and LCX can be seen.
The LAD leads, in direct continuation of the LM, to the anterior interventricular groove toward the apex and supplies 40% to 60% of the left ventricular myocardium.
The LAD gives rise to septal branches, diagonal branches, and branches to the free right ventricular wall. The LCX originates at an almost vertical angle from the LM and courses posteriorly at variable length along the left atrioventricular groove, beneath the left atrial appendage and toward the crux of the heart. The LCX gives off one to three obtuse marginal branches, which supply the free lateral left ventricular wall and have the same course as the diagonal branches of the LAD.
Right Coronary Artery
Usually the RCA arises from the right sinus of Valsalva and runs in the right atrioventricular groove toward the crux of the heart. The segment from the ostium to the right-angled turn into the vertical part of the RCA is called the proximal RCA, the mid-RCA is defined as the vertical segment, and the distal RCA extends from the right-angled turn at the distal end of the vertical segment to the bifurcation into the posterior descending coronary artery and posterolateral branches.
FIGURE 92.1. Schematic representation of the major epicardial coronary arteries as seen in an anteroposterior projection. The left coronary artery originates left and posterior from the cusp of the aortic root as the left main (LM) coronary artery. The LM coronary artery divides after a variable length into the left anterior descending (LAD) artery and the left circumflex (LCX) artery. The LAD runs as a direct continuation of the LM anteriorly along the interventricular groove to the ventricular apex. The LAD gives rise to the septal branches, which take off at a vertical angle and immediately become intramural in the interventricular septum. The LAD also gives rise to the diagonal branches, which course epicardially over the anterolateral free wall. The LCX originates at a nearly vertical angle from the LM and courses posteriorly along the left atrioventricular groove. It gives rise to the obtuse marginal branches, which course epicardially and supply the free lateral wall. The right coronary artery (RCA) takes off anteriorly from the right coronary cusp and follows the right atrioventricular groove. The first branch of the RCA is the conal or infundibular artery, followed by the sinus node artery as the second branch. In the vertical portion of the atrioventricular groove, the RCA gives rise to the right ventricular marginal branches, which supply the right ventricular free wall. The RCA then courses posteriorly and divides at the crux of the heart base into the posterior descending artery (PDA) and a variable number of posterolateral branches. The PDA courses along the posterior interventricular groove toward the left ventricular apex. It closes a loop with the LAD along with the interventricular groove, whereas the posterolateral branches of RCA close a second perpendicular loop of blood supply with the LCX along with the atrioventricular groove. C, conus branch; D, diagonal branch; OM, obtuse marginal branch; PA, pulmonary artery; PL, posterolateral branch; RV, right ventricular branch; S, septal branch; SN, sinus node artery.
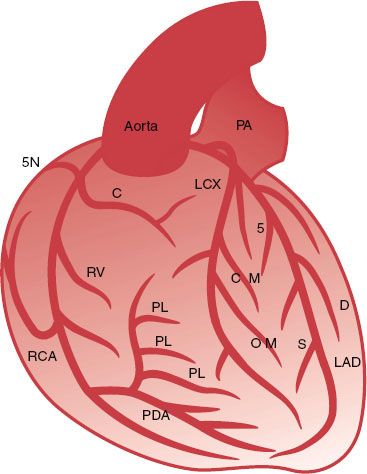
PERCUTANEOUS CORONARY INTERVENTION
(This section is modified with permission from Windecker S, Meier B. Basics of interventional cardiology. In: Tripuraneni P, Jani S, Minar E, et al., eds. Intravascular brachytherapy: from theory to practice. London: Remedica, 2001:88–100.) The indications for percutaneous coronary intervention (PCI) have expanded during the past two decades, and there is currently no absolute contraindication to this technique. Arterial access is usually gained through an anterior wall stick of the right femoral artery using the Seldinger technique. The coronary guidewire is advanced by the coronary guiding catheter into the coronary artery and is cautiously navigated through the narrowing (stenosis) into the periphery of the vessel to be treated. It secures access to the coronary artery during the intervention and allows for the rapid exchange of balloons, stents, and other devices (Fig. 92.2).
The balloon catheter not only is central to balloon angioplasty but also serves as a complementary instrument for other intracoronary interventions, such as delivery of stents, local drugs, or radiation sources. Balloon catheters consist of a shaft providing support when pushing the catheter through vessels, a central lumen for the coronary guidewire, and an inflation channel for balloon expansion.
During conventional balloon angioplasty (percutaneous transluminal coronary angioplasty), a balloon catheter is advanced over the previously inserted coronary guidewire into the target stenosis and subsequently inflated until the balloon is fully expanded. The chief limitations to event-free survival after balloon angioplasty have been abrupt vessel closure (a short-term complication) and restenosis (a long-term complication).6 Abrupt vessel closure, defined as the sudden occlusion of the target vessel during or after angioplasty, has been reported in 4% to 8% of cases. Restenosis, defined as stenosis >50% diameter at follow-up angiography, has been the most important long-term limitation of balloon angioplasty, with an incidence of 30% to 50% and with 20% to 30% of patients requiring target vessel revascularization. Most restenoses occur during the first 4 months after balloon angioplasty.
The therapeutic effect of arterial vessel enlargement through PCI is accompanied by various degrees of arterial injury with exposure of thrombogenic components. This may result in intracoronary thrombus formation with its subsequent ischemic sequelae. Therefore, inhibition of platelets and the coagulation system has always been a central focus of interventional investigations. In patients undergoing PCI, aspirin is recommended at a low dose (75 to 325 mg/day), ideally administered at least 1 day before the procedure and continued indefinitely thereafter. Ticlopidine and clopidogrel are typically administered with a loading dose before or after the procedure. Additionally, they are prescribed for ≥6 months after VBT if a new stent was not placed and ≥12 months if a new stent was placed under the discretion of the interventional cardiologist.18
EXTERNAL-BEAM IRRADIATION STUDIES
Results using external-beam irradiation have been mixed.33–38 Studies of external-beam irradiation in the pig coronary balloon angioplasty model, done at Emory University, revealed that when 14 Gy was administered immediately before or after or 2 days after balloon injury, there was reduced neointima formation compared with controls, but the lumens were smaller owing to negative remodeling (contracture) of the vessel. Results from studies using 21 Gy after either angioplasty or stenting indicate a profound and consistent suppression of neointima formation.38,39 The lack of benefit seen with a 14-Gy external beam compared with 14 Gy delivered by VBT at a 2-mm radius from the source suggests that the minimum dose delivered to the vessel wall is not the only factor in determining outcome. Studies of 14- and 21-Gy external radiation treatment have shown focal myocardial necrosis, an effect never seen with endovascular irradiation at any dose. This suggests that sophisticated treatment techniques, limiting the dose to normal tissues, will be essential for external-beam therapy to be adopted.
ENDOVASCULAR IRRADIATION STUDIES
In contrast to the disparate results from studies using external radiation, numerous studies have consistently demonstrated remarkable suppression of neointima formation using radiation from a variety of isotopes delivered by an endoluminal approach. At least three groups have documented similar results in the pig coronary artery model of restenosis after balloon angioplasty, using the γ emitter 192Ir at roughly comparable doses.40–42
VBT typically reduces the neointima formation and maintains the patency of the lumen. Scanning electron microscopy of arteries irradiated to 14 Gy showed no morphologic differences from controls at 2 weeks; a confluent layer of endothelial or endothelial-like cells was present throughout the region of the angioplasty injury. However, at 28 and 56 Gy, neointima formation was nearly eradicated and endothelial coverage was incomplete. Inadequate endothelial recovery of an irradiated artery after angioplasty might render its luminal surface prothrombotic. In the setting of an appropriate physiologic stimulus, this can result in thrombotic occlusion. The problem of late thrombosis observed in clinical trials certainly is compatible with the delayed healing observed in the animal studies.
With stents playing an increasingly important role in the management of coronary stenosis, it became important to test whether radiation might prove a useful adjunct to coronary stenting. Studies carried out with β and γ emitters have demonstrated conclusively that the excess intimal hyperplasia occurring in a stent can be effectively eliminated by radiation delivered either before or after stenting.43
FIGURE 92.2. Schematic diagram of access to the coronary arteries during percutaneous coronary interventions. A: An introducer sheath with side arm is placed in the artery chosen for vascular access. A guiding catheter is introduced through the vascular access sheath and advanced to the coronary artery ostium chosen for the intervention. B: A guidewire is advanced through the guiding catheter into the coronary artery, navigated through the artery, and placed distal to the stenosis to be treated. A balloon catheter is then placed in the coronary stenosis for dilatation.
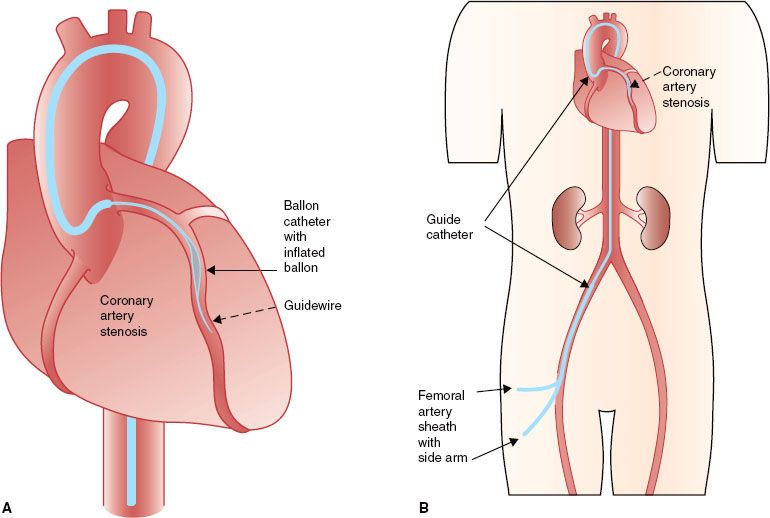
VASCULAR BRACHYTHERAPY PHYSICS AND DEVICES
The initial evaluation of radiation therapy in animal models of restenosis focused on testing the effect of radiation with commercially available radiation sources. Testing has included both teletherapy and brachytherapy. Although occasional positive results have been reported with teletherapy in experimental models of restenosis, consistently positive results emerged from a variety of brachytherapy approaches. These approaches have included both temporary (VBT) and permanent implants (radioactive stents). Although many isotopes have been proposed for VBT, only approximately half a dozen have been used in human clinical trials, and of those, only three have seen widespread use. The three isotopes, which have been used for well over 95% of all clinical trials of catheter-based systems, are 192Ir, 90Sr/Y, and 32P (Table 92.1).
BETA-CATH SYSTEM FOR CORONARY IN-STENT RESTENOSIS
The Beta-Cath System is the only system currently manufactured that is offered for in-stent restenosis. 90Sr is a pure β emitter with a 28.8-year half-life (10,519.25 days) and 546-KeV maximum β energy. The daughter isotope 90Y is also a pure β emitter with a 64-hour half-life and 2.27-MeV maximum β energy. It is primarily the 90Y β emissions that are used for therapy because the 90Sr β particles are mostly absorbed by the stainless steel encapsulation and the surrounding catheter. The Beta-Cath System contains sources that are 0.38 mm in diameter and 2.5 mm in length confined within a flexible steel coil between radiopaque end plug markers that are maximum 0.495 mm in diameter and 2.5 mm in length. Source trains of 12, 16, and 24 sources (30-, 40-, and 60-mm active lengths, respectively) are commercially available. The nonradioactive marker plugs are located both proximally and distally to the radioactive sources in the jacketed train (Fig. 92.3). The markers permit fluoroscopic verification of the source train arriving at the end of the delivery catheter for treatment. For lesions plus margins longer than the available source train, a “pullback technique” is used in which the most distal portion of the lesion is treated first, and then the catheter is carefully pulled back to treat the more proximal lesion. At Scripps Clinic, we have the 60-mm source train available. A 60-mm source train can be used with a pullback technique to cover a distance of 120 mm. This technique could also be used for treatment of lesions at vessel bifurcations.44 Significant overlap or gaps between the treatment fields should be avoided. A careful review of the cine angiograms and a thorough understanding of the quantity, spacing, and positions of the radiopaque markers in the system (i.e., the two source train end markers, the delivery catheter stop marker, and the removable indicator of the source train marker wire) are pertinent when using this technique.
The Beta-Cath System consists of four main components: the source train, transfer device, delivery catheter, and accessories. The sources are stored in a hand-held transfer device and are advanced by a closed-loop hydraulic system that uses sterile water to send (and then return) the source train. The advantage of the Beta-Cath System is the relatively short treatment times (3 to 5 minutes) and the absence of radiation exposure to catheterization laboratory staff. The long half-life of the isotope permits a total shelf life of 12 months divided into two 6-month use periods, which allows for a decay correction of a few seconds in the second 6-month dwell times. A potential disadvantage of this system is the inferior depth–dose gradient compared with the γ source, attenuation by calcifications or stents, and lack of utility in larger vessels (Fig. 92.4).
A dose of 18.4 Gy is recommended at a 2-mm radius from the centerline of the source train axis for vessels with a reference diameter between 2.7 and 3.35 mm. For reference diameters between 3.35 and 4 mm and for most saphenous vein grafts, a dose of 23 Gy is recommended. The gross target volume is the stenotic area itself. The clinical target volume is the dilated part of the vessel. The planning target includes at least 5 mm proximal and distal to the clinical target volume45–47 for the 30-mm source train and at least 10 mm for the 40-mm and 60-mm source trains. Although both minimum 5-mm and minimum 10-mm treatment margins were proven safe and effective in the Beta-Cath System START trials, a cumulative comparison of efficacy outcomes out to 5 years suggests that longer treatment margins yield significantly lower target vessel revascularization (TVR) rates of 51% for minimum 5-mm margins versus 19% for 10-mm minimum margins versus 55% for the placebo, and with nonsignificant differences in major adverse coronary event (MACE) rates of 63% with minimum 5-mm margins versus 67% for minimum 10-mm margins versus 68% for the placebo (User’s Manual, Novoste Beta-Cath System).
TABLE 92.1 SUMMARY OF CATHETER-BASED SYSTEMS
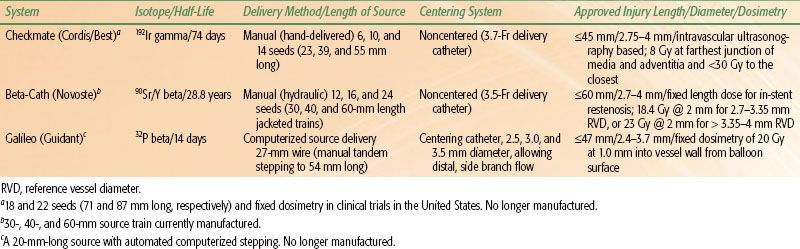
FIGURE 92.3. Radiation source train with proximal and distal radiopaque markers noted. (Courtesy of Novoste Corporation, Norcross, GA.)
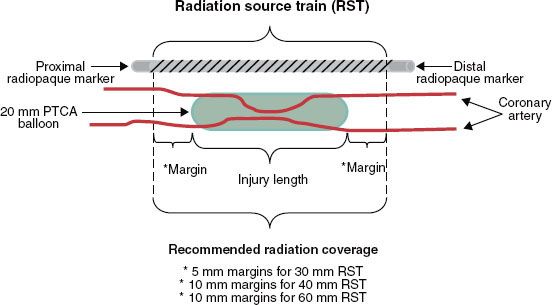
Roles and Responsibilities
(This text is modified and printed with permission from Tripuraneni P. In: Tripuraneni P, Jani S, Minar E, et al., eds. Intravascular brachytherapy: from theory to practice. London: Remedica, 2001:272–274.) The FDA has mandated that VBT be carried out by a team consisting of an interventional cardiologist/radiologist, a radiation oncologist/therapeutic radiologist and oncologist, and a medical physicist. The roles and responsibilities of these specialists are listed in the following sections.31
Interventional Cardiologist/Radiologist
1. Perform preprocedure evaluation and communicate patient’s status (risk factors, interventions) with radiation oncologist.
2. Perform angioplasty with or without stenting as necessary.
3. Define the anatomic location of diseased vessel amenable to intervention, length/volume of intervention, angioplasty and stenting, and reference vessel diameter (including preintervention and postintervention vessel segment diameters), and communicate with radiation oncologist.
4. Determine the target volumes, jointly with the radiation oncologist and medical physicist.
5. Place delivery catheter and make final adjustment, in consultation with the radiation oncologist.
6. Advise the radiation oncologist of any changes in the delivery catheter position during the delivery of radiation.
7. Assist the radiation oncologist with any procedural details.
8. Assist the radiation oncologist in source removal as needed. In cases of medical or radiation emergencies, have procedures in place to ensure that radiation treatments are preplanned and nothing is left to chance.
Therapeutic Radiologist/Oncologist (Authorized User)
1. Review preprocedure evaluation, including patient’s status (including risk factors and interventions), with interventional cardiologist for the advisability of using intravascular radiation.
2. Review the anatomic location of diseased vessels amenable to intervention, length/volume of intervention, angioplasty, and stenting, and reference vessel diameter (including preintervention and postintervention vessel segment) with the interventional cardiologist.
3. Determine the target volumes together with the interventional cardiologist.
4. Obtain proper informed consent for VBT after discussions with the patient.
5. Review the final placement of the delivery catheter and any adjustments as needed.
6. Prescribe radiation dose and sign prescription.
7. Calculate treatment times, along with the medical physicist.
8. Insert radiation source.
9. Provide appropriate delivery of radiation.
10. Remove radiation source.
11. Supervise overall radiation delivery.
12. Participate in decisions and implementation of emergency source removal in case of medical or radiation emergencies.
Medical Physicist
1. Survey catheterization laboratory or radiation source delivery room and make appropriate preparations and modifications as needed.
2. Order radiation sources, under the direction of the radiation oncologist.
3. Calibrate sources upon arrival.
4. Ensure safe-keeping of radiation sources.
5. Prepare sources for clinical use.
6. Calculate treatment delivery times.
7. Conduct radiation survey of patient before and during treatment.
8. Assist radiation oncologist and interventional cardiologist in source removal in cases of radiation or medical emergencies.
9. Conduct radiation survey of patient after source removal.
10. In case of radiation or medical mishaps, inform appropriate regulatory authorities.
11. Participate in preparation of license application for medical use of VBT sources.
12. Develop and oversee quality assurance and improvement programs for efficacious and safe use of VBT sources in consultation with the radiation oncologist and interventional cardiologist as appropriate.
FIGURE 92.4. The Beta-Cath system is a hydraulic delivery system with a noncentered, 5-Fr, closed, over-the-wire delivery catheter. 90Sr seeds 24 source train (60 mm in length) is available. The delivery unit is shown. (Courtesy of Novoste Corporation, Norcross, GA.)
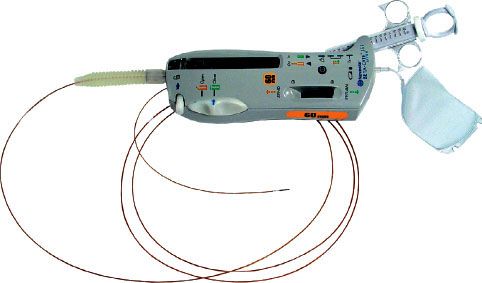
CLINICAL TRIALS OF CORONARY BRACHYTHERAPY
Nearly 5,000 patients have participated in clinical trials to determine the safety and efficacy of VBT.25,48,49 There have been seven double-blind randomized trials investigating the use of VBT on patients with in-stent restenosis.24,25,48,50–55 These trials led to the approval of one γ system using 192Ir and two β systems using 32P and 90Sr/Y isotopes.25,56,57
Of note, Rha et al.58 have reported that results from commercially available VBT are superior to the results obtained during investigational VBT trials as MACEs were lower with commercial radiation. This is likely from the lessons learned through clinical trials as dosimetry was optimized (higher doses, wider margins) and prolonged antiplatelet therapy was administered to reduce late thrombotic events. More recently, a rhenium-188–filled balloon source has also been shown to be effective for in-stent restenosis and improving long-term outcomes.59
TABLE 92.2 SUMMARY OF PUBLISHED OR PRESENTED TRIAL RESULTS FOR CORONARY IN-STENT RESTENOSISA
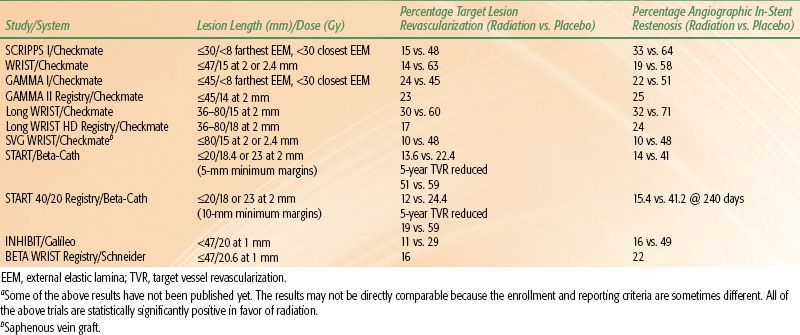
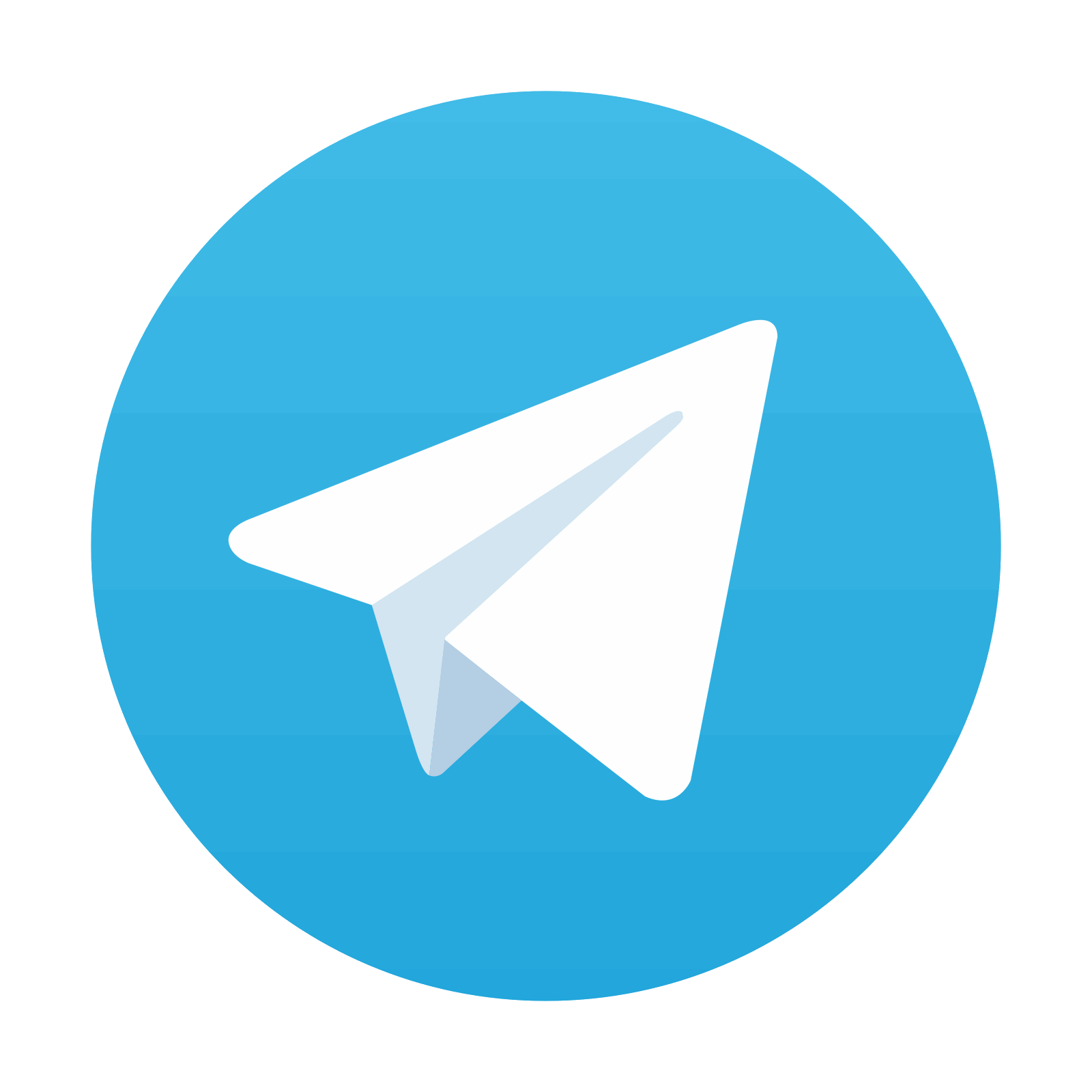