Endothelial Cell Function
William C. Aird
The endothelium is an expansive organ, reaching all recesses of the human body. At one time, it was believed that endothelial cells (ECs) were relatively inert, acting as a physical barrier between blood and underlying tissue. It is now well established that ECs are highly metabolically active, and play a critical role in many physiologic processes, including the control of vasomotor tone, the trafficking of leukocytes between blood and underlying tissue, the maintenance of blood fluidity, permeability, angiogenesis, and both innate and adaptive immunity. EC phenotypes display remarkable heterogeneity in structure and function, in space and time, and in health and disease (reviewed in1,2,3). The goals of this chapter are to review the scope and nature of endothelial phenotypes and to apply these principles to an understanding of the endothelium in hemostasis and thrombosis.
ENDOTHELIAL CELL PHENOTYPES
Defining the Endothelium
An interesting question is how do we define the endothelium? Anatomically, the endothelium is the cell layer that lines the inner wall of blood and lymphatic vessels. However, there are examples of vascular mimicry in which other cell types (e.g., trophoblasts) form the inner lining of blood vessels. ECs tend to be extremely flat or thin. In that way, the diffusional path length for gases and solutes across the capillary wall is minimized. However, there are exceptions, including the plump, cuboidal ECs of high endothelial venules (HEV) (reviewed in Ref.4). Weibel-Palade bodies, which contain von Willebrand factor (vWF) and P-selectin, are specific to the endothelium, but there is no evidence that these storage granules are present in all ECs. Other ultrastructural features that are characteristic of ECs, such as caveolae, are also observed in other cell types. During development, endothelium arises from mesoderm via the differentiation of hemangioblasts and/or angioblasts. However, other cell lineages may transdifferentiate into ECs, and ECs into other lineages. From a molecular perspective, few, if any, genes are both specifically and uniformly expressed in the endothelium. For example vWF is also expressed in megakaryocytes and its expression in the endothelium is patchy. CD31 (also termed platelet-endothelial cell adhesion molecule 1) is also expressed in monocyte/macrophages, and vascular endothelial (VE)-cadherin in trophoblasts and fetal stem cells. Functionally, the endothelium displays a remarkable division of labor, whereby cells from different segments of the vasculature carry out unique activities. Finally, when endothelial-specific promoters are targeted to the mouse genome, they invariably fail to direct expression throughout the endothelium. Rather, they promote expression in specific subsets of ECs (reviewed in5). In summary, each of the above definitions—anatomical, structural, functional, and molecular—falls short of fully capturing the endothelium. The “elusiveness” of the endothelium undoubtedly reflects its marked heterogeneity in structure and function. Indeed, far from being a giant monopoly of identical cells, the endothelium is a consortium of smaller enterprises, each uniquely adapted to the needs of the local tissue. In the final analysis, a full understanding of this cell type in health and disease requires a consideration of both the spatial and temporal dynamics of the system.
Scope of Heterogeneity
EC heterogeneity has been described at the level of cell morphology, function, gene expression, and antigen composition. EC phenotypes vary between different organs, between different segments of the vascular loop within the same organ, and between neighboring ECs of the same organ and blood vessel type. In vivo proteomic approaches have revealed a striking array of vascular bed-specific phenotypes (reviewed in6,7). For example, antibody and subfractionation strategies have been employed to generate monoclonal antibodies that specifically target the caveolae in one vascular bed or another.8 Others have used phage display peptide libraries to select for peptides that home to specific vascular beds in vivo.9,10 These latter studies have uncovered a vascular address system that allows for site-specific targeting of biologically active compounds, for example, to the endothelial lining of tumor blood vessels.11
Structural Heterogeneity
ECs that line straight segments of arteries, but not those lining veins, are oriented along the longitudinal axis of blood flow. Previous studies have demonstrated that flow-dependent alignment of ECs represents reversible endothelial structural remodeling in response to hemodynamic shear stress.12,13 The transfer of material across the endothelium, a process termed transcytosis, is mediated by caveolae and vesiculo-vacuolar organelles (VVOs). Caveolae are 70-nm membrane-bound, flask-shaped vesicles that open to the luminal or abluminal side of the cell. The density of caveolae in capillary endothelium (up to 10,000 per cell) greatly exceeds that in the arteries, arterioles, veins, or venules (reviewed in Refs.2,14). An exception is the blood-brain barrier, where the number of caveolae is greatly reduced.15 VVOs comprise focal collections of membrane-bound vesicles and vacuoles. They are most prominent in venular endothelium.16 In contrast, clathrin-coated pits, which are involved in endocytosis, are particularly prominent in liver sinusoidal endothelium.
The endothelium of arteries and veins forms a continuous uninterrupted layer of cells, held together by zipper-like tight junctions. The endothelium of capillaries may be continuous, fenestrated, or discontinuous, according to the needs of the underlying tissue. For example, fenestrated endothelium is characteristic of organs involved in filtration or secretion, including exocrine and endocrine glands, gastric and intestinal mucosa, choroid plexus, glomeruli, and a subpopulation of renal tubules. Discontinuous endothelium is similar to fenestrated endothelium, with the exception that the fenestrations are larger in diameter (they may manifest as gaps in the cell) and they lack a diaphragm. Moreover, the underlying basement membrane is poorly formed. Discontinuous endothelium is found in certain sinusoidal vascular beds, including the liver.
Functional Heterogeneity
The endothelium has many functions. In almost every case, the activity is carried out by distinct subsets of ECs within the vascular tree. Below, we discuss three examples of functional heterogeneity: permeability, leukocyte trafficking, and angiogenesis. We review vascular bed-specific hemostasis and thrombosis separately in a subsequent section.
Permeability
Basal and inducible permeability is differentially regulated across the vascular tree. Constitutive flow of material between blood and underlying tissue takes place primarily at the level of capillaries. Transport of fluids and small solutes occurs in between cells (the so-called paracellular route), whereas macromolecules are transferred through the cell (the transcellular route) via caveolae, VVOs, and transendothelial channels. Basal permeability is lower in nonfenestrated vascular beds that are enriched in tight junctions and possess fewer caveolae (e.g., the blood-brain barrier). As evidence for the role of tight junctions in mediating barrier function, mice that null for the tight junction molecule, claudin-5, have a selective barrier defect in the brain.17 Inducible permeability refers to changes in barrier function that occur in acute or chronic inflammation. These changes take place primarily in postcapillary venules. The extent to which regulated leakiness is mediated by paracellular or transcellular pathways is debated. The predilection for postcapillary venules as a site for inducible permeability may be explained by the relative abundance of VVOs, the relatively low number of tight junctions, and/or high expression levels of agonist-responsive receptors (reviewed in Ref.2). Severe inflammation may result in increased permeability in sites other than postcapillary venules, including large veins, arterioles, and capillaries.
Leukocyte Trafficking
The endothelium plays a key role in regulating the trafficking of leukocytes between blood and underlying tissue (hence the metaphor of the endothelium as gatekeeper). Leukocyte trafficking involves a multistep cascade that begins with rolling, followed by firm adhesion and transmigration (reviewed in Ref.2,18,19,20). As with transfer of fluids and solutes, transmigration may occur between ECs (the paracellular pathway) or through ECs (the transcellular pathway). Each step in the cascade is mediated by the upregulation of cell adhesion molecules on the surface of the endothelium as well as the expression of chemokines. Binding of endothelial E-selectin and P-selectin to leukocyte carbohydrate-based ligands promotes rolling of leukocytes on the endothelium, whereas binding of endothelial cell adhesion molecules, including vascular cell adhesion molecule (VCAM)-1 and intercellular adhesion molecule (ICAM)-1 to leukocyte integrins mediates firm adhesion. The mechanisms of transmigration are poorly understood, but involve CD31 and junctional adhesion molecule-1. Similar to inducible permeability, transfer of white blood cells occurs primarily in postcapillary venules. One mechanism underlying this sitespecificity is the preferential expression of E-selectin, P-selectin, VCAM-1, and ICAM-1 in the endothelium of postcapillary venules. Under certain conditions, leukocyte trafficking may occur in other segments of the vascular tree, including large veins, arterioles, and capillaries. For example, previous studies suggest that leukocyte sequestration and transmigration in the pulmonary circulation occurs primarily in alveolar capillaries by a rolling- and E-/P-selectin-independent mechanism that involves trapping of poorly deformed activated leukocytes on activated endothelium.21,22 Similarly, in liver inflammation, the majority of leukocyte adhesion occurs in the sinusoidal endothelium.23 Another form of leukocyte trafficking is the transmigration of lymphocytes across the specialized postcapillary venules, termed HEV, which are located in secondary lymphoid organs (reviewed in Ref.24). Trafficking at these sites occurs constitutively, and is mediated by interactions between lymphocyte L-selectin and its ligand (peripheral node addressin) on HEV ECs. In summary, the “universal cascade” for leukocyte trafficking is, in fact, mediated by spatially and temporally diverse mechanisms.
Angiogenesis
Recent studies have emphasized the existence of structural and functional heterogeneity of ECs during angiogenesis. For example, endothelial sprouts comprise leading-edge tip cells followed by morphologically and functionally distinct stalk cells. The tip cells are enriched in filopodia and in response to guidance cues, they promote the extension of new sprouts and fusion with adjacent sprouting capillaries to form new vascular connections (reviewed in Ref.25). This process involves the coordinated activity of Notch and Wnt signaling.
Molecular Heterogeneity
Spatial and temporal differences in the structure and function of ECs ultimately reflect differences in mRNA and protein expression. Endothelial genes may be classified according to whether they are constitutively or inducibly expressed, whether they are specific or not to ECs, and whether they are expressed throughout the endothelium or in subsets of ECs (reviewed in5). There are remarkably few endothelial-specific genes that are constitutively expressed throughout the vascular tree. VE-cadherin and Robo4 are two such genes. There are many more examples of endothelial-restricted genes whose constitutive and/or inducible expression is limited to subsets of ECs. For example, several endothelial markers are expressed specifically on the venous versus arterial side of the circulation (reviewed in Ref.26). ECs from different arteries, and even from different sites of the same artery, express overlapping but distinct molecular profiles. An example of an inducible, endothelial-restricted gene is E-selectin, which is expressed in activated, but not “resting”
endothelium. In activated endothelium, E-selectin expression is largely confined to postcapillary venules.
endothelium. In activated endothelium, E-selectin expression is largely confined to postcapillary venules.
Mechanisms of Endothelial Heterogeneity
EC heterogeneity is mediated by one of two proximate mechanisms.27 First, some site- and time-dependent differences in endothelial properties are governed by differences in the extracellular milieu. Because blood vessels are distributed throughout the body, their endothelial lining is exposed to an enormous variety of tissue microenvironments. In so far as ECs are capable of sensing and responding to their environment, the wide range of signal inputs from one organ to the next is sufficient to generate phenotypic heterogeneity across the vascular tree. When ECs are removed from their native tissue and grown in tissue culture, they become uncoupled from critical extracellular cues and undergo phenotypic drift. For this reason, studies of cultured ECs are fraught with limitations. Second, certain site-specific properties are epigenetically “fixed” and impervious to changes in the extracellular environment. Such properties are mitotically stable, and are thus retained under in vitro culture conditions. The relative roles of epigenetic and nonepigenetic forces in mediating phenotypic heterogeneity are not fully understood. A previous DNA microarray study of multiple human EC types grown in culture revealed site-specific signatures even in multiply passaged cells, providing genome-wide evidence for the importance of epigenetics in mediating differential gene expression.28 A study of ECs from human tonsils revealed that approximately 50% of the vascular bed-specific genes were “washed out” when cultured in vitro, implicating a role for both the tissue environment and epigenetics in mediating differential gene expression.29 This conclusion was supported by a DNA microarray study in which the transcriptome of ECs was compared between porcine coronary and iliac arteries. The data revealed that most, but not all, differences observed in vivo were lost in multiply passaged cells.30
In addition to proximate mechanisms, every biologic trait requires an evolutionary explanation. Proximate explanations (how?) employ traditional approaches of cell biology and molecular biology to determine the anatomy, physiology, and ontogeny (developmental history) of a trait at the level of a single organism. By contrast, evolutionary explanations (why?) draw on the fossil record and comparative morphology and DNA sequences to uncover the phylogeny (evolutionary history) of a trait as well as the fitness advantage that the trait provides at the level of a population or species. Comparative studies have revealed that the endothelium is present in all vertebrate species, but absent in invertebrates. Thus, the endothelium evolved in a common ancestor of the vertebrates following the divergence of the urochordates and cephalochordates some 500-600 million years ago over a period of only 50-100 million years. This same short period witnessed the evolution of a closed circulation, the clotting cascade (see Chapter 2), and acquired immunity.
An interesting question is whether EC heterogeneity, as a trait, appeared early on during evolution of the endothelium or whether it appeared later during vertebrate evolution. One way to address this question is to study hagfish, the oldest extant vertebrate. Any feature that is shared by hagfish and humans may be inferred to have evolved in the ancestral vertebrate. Unlike other vertebrates, hagfish are completely devoid of bone and cartilage. However, similar to the jawed vertebrates, hagfish have a closed circulation lined by endothelium. Importantly, our studies revealed that hagfish endothelium is remarkably heterogeneous at the level of ultrastructure, lectin binding, and function.31,32 We may conclude from these observations that phenotypic heterogeneity evolved as an early feature of the endothelium. In other words, endothelial heterogeneity is not simply a descriptor of many distinct properties of the endothelium, but rather is itself a core property of the endothelium.
Evolutionary approaches also seek to explain the fitness advantage that a trait confers at a population level. This is a difficult question to definitively answer, but there are two important considerations. One is that the endothelium in traversing the body must provide a broad menu of functions that are adapted to the diverse needs of the underlying tissues. Site-specific differences in function will, in turn, be reflected by structural and molecular heterogeneity. Second, ECs must adapt to different environments simply to survive. For example, ECs in the vasa recta of the inner medulla of the kidney are exposed to profoundly low levels of oxygen, in addition to a hyperosmolar hyperkalemic environment. Although the precise properties of these cells are unknown, it is certain that they are uniquely adapted to this “harsh” environment in ways that render them phenotypically distinct from other types of ECs.
ENDOTHELIAL CELL ACTIVATION AND DYSFUNCTION
The two most common terms used to describe the endothelium in disease are activation and dysfunction. The term endothelial cell activation was introduced in the 1980s when it was observed that numerous inflammatory mediators, including endotoxin, tumor necrosis factor, and interleukin (IL)-1, induced the expression of new antigens (so-called activation antigens) on the surface of cultured ECs, and was correlated with the expression of proadhesive, antigen-presenting, and procoagulant activities (reviewed in33). Today, the term activation is used more broadly to describe the response of ECs to an inflammatory stimulus in vitro or in vivo (some investigators in the angiogenesis field employ the term to describe proliferating ECs). The activation phenotype varies according to the nature of the activation agonist, the net signal input, and the vascular bed of origin. Typically, it consists of some combination of a proadhesive and procoagulant surface, increased permeability, and the release of inflammatory mediators. Activation should not be confused with activity. The normal endothelium is highly active, constantly sensing and responding to cues in its extracellular environment. EC activation describes a phenotype. It does not refer to the cost of that phenotype to the host. In other words, EC activation may be adaptive or nonadaptive.
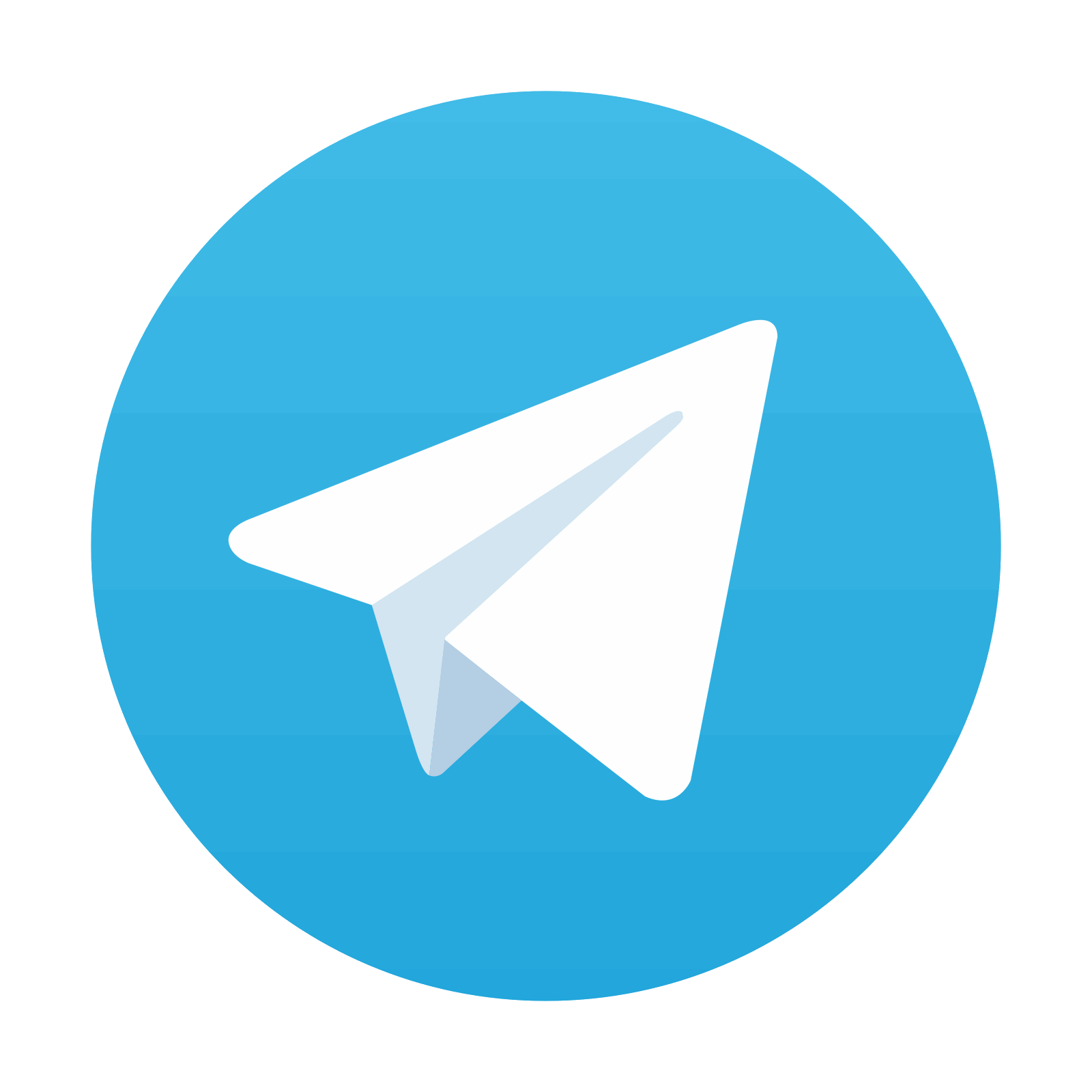
Stay updated, free articles. Join our Telegram channel

Full access? Get Clinical Tree
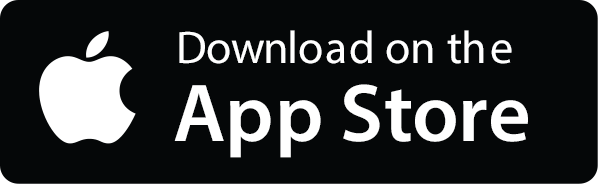
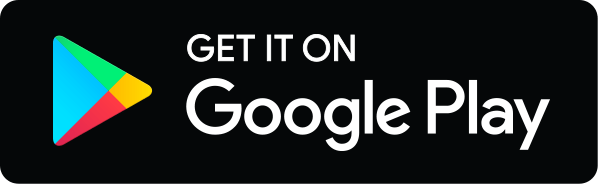